Agent
Cumulative dose associated with azoospermia
Cyclophosphamide
>5–7.5 g/m2 associated with abnormal semen parameters, >19 g/m2 consistently result in azoospermia
Ifosfamide
>60 g/m2
Procarbazine
>4 g/m2
Busulfan
>600 mg/m2
Melphalan
>140 mg/m2
Cisplatin
>600 mg/m2
More recently, in order to be able to better assess alkylating agent exposure and risk of gonadotoxicity, two methods, the summed alkylating agent dose (AAD) score (Table 25.2) and cyclophosphamide equivalent dose (CED) (see Table 25.3), have been used.
Table 25.2
Summed alkylating agent dose score
Alkylating agent | Tertile score | ||
---|---|---|---|
1 | 2 | 3 | |
BCNU (carmustine) | 1–300 | 301–529 | 530–5370 |
Busulfan | 1–317 | 318–509 | 510–6845 |
CCNU (lomustine) | 1–361 | 362–610 | 611–3139 |
Chlorambucil | 1–165 | 166–634 | 635–3349 |
Parenteral cyclophosphamide | 1–3704 | 3705–9200 | 9201–58,648 |
Oral cyclophosphamide | 1–4722 | 4723–10,636 | 10,637–143,802 |
Ifosfamide | 1–16,771 | 16,772–55,758 | 55,759–192,391 |
Melphalan | 1–39 | 40–137 | 138–574 |
Nitrogen mustard | 1–44 | 45–64 | 65–336 |
Procarbazine | 1–4200 | 4201–7000 | 7001–58,680 |
Intrathecal thiotepa, mg | 1–80 | 81–320 | 321–914 |
Thiotepa | 1–77 | 78–220 | 221–3749 |
Cumulative doses in mg/m2 except where noted |
Table 25.3
Cyclophosphamide equivalent dose
CED (mg/m2) = | 1.0 × cumulative cyclophosphamide dose (mg/m2) |
+0.244 × cumulative ifosfamide dose (mg/m2) | |
+0.857 × cumulative procarbazine dose (mg/m2) | |
+14.286 × cumulative chlorambucil dose (mg/m2) | |
+15.0 × cumulative BCNU dose (mg/m2) | |
+16.0 × cumulative CCNU dose (mg/m2) | |
+40 × cumulative melphalan dose (mg/m2) | |
+50 × cumulative thiotepa dose (mg/m2) | |
+100 × cumulative nitrogen mustard dose (mg/m2) | |
+8.823 × cumulative busulfan dose (mg/m2) |
In a large CCSS study [11], the summed AAD score was found to be inversely correlated with the likelihood of siring a pregnancy with those with higher AAD scores having a lower likelihood of siring a pregnancy: summed AAD score of 2 (HR, 0.67), 3(HR, 0.48), 4 (HR, 0.34), 5 (HR, 0.38), or 6–11 (HR, 0.16). In addition, those who received a higher cumulative dose of cyclophosphamide (third tertile HR 0.42) or procarbazine (second tertile HR 0.48, third tertile HR 0.17) were also less likely to sire a pregnancy. The summed AAD score or 3 or higher was also found to be a risk factor for infertility on multivariate analysis in another CCSS study of 938 male childhood cancer survivors and 174 siblings who had tried to become pregnant [12].
Similarly, the CED was inversely correlated with the likelihood of siring a pregnancy, with a statistically significantly decreased likelihood of siring a pregnancy in those who received a CED ≥ 4000 mg/m2 (4000–<8000 HR 0.72; 8000–<12,000 HR 0.49; 12,000–<16,000 HR 0.37; 16,000–<20,000 HR 0.53; ≥20,000 HR 0.17) [32]. The CED was also found to be negatively correlated with sperm concentration in 214 adult male long-term childhood cancer survivors on the St. Jude Life Cohort [33], with a mean CED of 10,830 mg/m2 in those who were azoospermic, 8480 mg/m2 in the oligospermic, and 6626 mg/m2 in the normospermic. Those with a CED <4000 mg/m2 were very likely to be normospermic. However, there was a substantial overlap of CED with normospermia, oligospermia, and azoospermia. It is thus postulated that genetics, pharmacogenomics, and other factors may play a role.
Younger age or prepubertal state does not seem to be gonadoprotective [11, 21, 22]. They exhibit similar rates of azoospermia or oligospermia as postpubertal males. Despite this, prepubertal males who received gonadotoxic chemotherapy still seem to be just as likely to be able to undergo puberty after chemotherapy [22].
Radiation has significant gonadotoxic effects as well. Patients treated for tumors in the pituitary-hypothalamic region or with testicular radiation have severe gonadal and sexual dysfunction [23]. In a CCSS study, partners of male survivors whose testes were in or near the radiation field or whose testes were shielded had very few live births [34].
The testicular germinal epithelium is sensitive to the gonadotoxic effects of radiation. Testicular radiation doses as low as 0.1–1.2 Gy can cause oligo- or azoospermia by damaging dividing spermatogonia and disrupting cell morphology [35, 36]. Permanent azoospermia has also been reported following a single fraction of testicular radiation with 4 Gy or 1.2 Gy fractionated [35, 36].
Leydig cells are more resistant to damage from radiation, and despite severe impairment of spermatogenesis, survivors are frequently noted to have normal pubertal progression and normal potency. The extent of damage may be related to the dose and age at which radiation is delivered [36]. Testicular radiation dose >20 Gy in prepubertal males and >30 Gy in sexually mature males is associated with Leydig cell dysfunction [37].
25.1.1.1 Pregnancy Outcome
Many male childhood cancer survivors are able to sire pregnancies. In a Childhood Cancer Survivor Study (CCSS) [24], 1227 out of 4106 sexually active male childhood cancer survivors reported siring 2323 pregnancies. Of these, 69 % resulted in live births, 1 % stillbirths, 13 % miscarriages, 13 % abortions, and 5 % had unknown outcomes or were in gestation. The proportion of live births was lower for partners of childhood cancer survivors compared to partners of male siblings of cancer survivors. Pregnancy outcome did not seem to be affected by the type of chemotherapy the survivors received. However, the rate of miscarriage was higher for partners of male survivors treated with >5000 mg/m2 procarbazine compared to those who received less or no procarbazine. Even among survivors who met the definition for infertility, a little over a third reported at least one pregnancy with a female partner that resulted in a live birth, suggesting there may be periods of fertility and infertility [12]. Although sperm concentration is reduced after cancer therapy, studies have shown that the sperm produced seems to carry as much healthy DNA as sperm from the healthy population [13]. This suggests that assisted reproduction may be considered in these patients.
25.1.2 Female
After cancer treatment, females can develop acute ovarian failure which may or may not recover, premature menopause, and varying degrees of sub- or infertility [3]. Acute ovarian failure occurred in 6.3 % and premature nonsurgical menopause in 8 % of childhood cancer survivors in the CCSS [38]. Adult female childhood cancer survivors are less likely to have ever been pregnant [31] with an increased risk of clinical infertility compared to siblings, most pronounced in the early reproductive ages, and an increased time to first pregnancy [10]. Particularly at risk are those who are of or approaching reproductive age at the time of cancer treatment, those who have received high doses of alkylating agents (particularly cyclophosphamide, lomustine, and procarbazine), and those diagnosed with Hodgkin lymphoma [31, 38–43].
Those who had a summed AAD score (Table 25.2) ≥3 were less likely to have ever been pregnant and to develop nonsurgical premature menopause [31, 44]. Similarly, increasing CED (Table 25.3) was associated with a higher likelihood of developing nonsurgical premature menopause (CED 4000–<8000 mg/m2 HR 0.72; 8000–<12,000 HR 0.49; 12,000–<16,000 HR 0.37; 16,000–<20,000 HR 0.53; ≥20,000 HR 0.17) [32].
Direct or scatter radiation to the female reproductive organs (either by total body irradiation TBI), spinal, abdominal and/or pelvic radiation) may also cause ovarian and/or uterine damage.
The human oocyte is sensitive to radiation with an estimated lethal dose (LD50) <2 Gy [45]. Abdominal radiation results in severely damaged ovaries with follicle growth inhibited in most cases, and the number of small, nongrowing follicles markedly reduced in most [46]. Increasing doses of radiation to the ovaries (especially >10 Gy) result in increased likelihood of developing acute ovarian failure or nonsurgical premature menopause [38]. Predictions to estimate the age of menopause after radiation may be made using an adaptation of the Faddy-Gosden model by Wallace et al. [45].
Uterine radiation in childhood increases the incidence of nulliparity, spontaneous miscarriage, and intrauterine growth retardation. Although the mechanism is unknown, it is believed to be due to reduced elasticity of uterine musculature and uterine vascular damage [47, 48].
Female childhood cancer survivors who received hypothalamic/pituitary radiation ≥30 Gy (RR 0.61) or ovarian/uterine radiation dose >5 Gy (RR0 0.56 for 5–10 Gy, RR 0.18 for >10 Gy) were less likely to have ever been pregnant [10, 31].
25.1.2.1 Pregnancy Outcomes
Pregnancy outcomes may be affected by cancer treatment in females as well. Female childhood cancer survivors are less likely to have a live birth. In the CCSS study [49] of 4029 pregnancies in 1915 survivors, 63 % resulted in live births, 1 % stillbirths, 15 % miscarriages, and 17 % abortions and 3 % had unknown status or were in gestation. The type of treatment did not have a significant impact on pregnancy outcome although there was a trend toward a higher risk of miscarriage if the ovaries were irradiated or near the radiation field and there was a higher risk of miscarriage with spinal radiation. No adverse pregnancy outcomes were noted with most chemotherapy agents.
Children born to female childhood cancer survivors were more likely to be born preterm and small for gestational age, especially those born to women who received uterine radiation doses >5 Gy [38, 50]. The effect of uterine radiation may be greater and the threshold lower for girls treated prior to menarche. Differences in gender ratio and increases in simple malformations, cytogenetic syndromes, or single-gene defects have not been noted in offspring of female survivors [49].
25.2 Surrogate Markers of Fertility
Pregnancy or siring a pregnancy is the best measure of fertility. However, the use of pregnancies as a measure of fertility is limited by the need to wait until adulthood and attempts for pregnancy. Surrogate measures of fertility are therefore necessary to assess the effect of cancer treatment on fertility. Several markers have been used to assess ovarian and testicular reserve as surrogate measures of fertility (Table 25.4). Of note, most of the research regarding these markers has been performed in adults seeking treatment for infertility [51]. These markers can be affected by a variety of factors, including age at the time of testing, age at the time of chemotherapy and/or radiation therapy and/or gonadal surgery, type and cumulative dose of chemotherapy received, dose and target site of radiation received, genetic factors, other illnesses, and history of infertility.
Table 25.4
Measures of ovarian and testicular reserve
(A) Measures of ovarian reserve |
(a) Menstrual cycles |
(b) Antral follicle counts |
(c) Endocrine hormones |
(i) Follicle-stimulating hormone (FSH) |
(ii) Luteinizing hormone (LH) |
(iii) Estradiol (E2) |
(iv) Inhibin B |
(v) Anti-Mullerian hormone (AMH) |
(B) Measures of testicular reserve |
(a) Semen analysis |
(b) Endocrine hormones |
(i) Follicle-stimulating hormone (FSH) |
(ii) Luteinizing hormone (LH) |
(iii) Testosterone |
(iv) Inhibin B |
The differences in male and female reproductive systems influence the available methods for assessing ovarian and testicular reserve as well as options for fertility preservation. In females, it is generally accepted that oocyte production ceases in fetal life and a female is born with a finite number of follicles (ovarian reserve) which diminishes throughout life until menopause [52]. At 5-month gestation, the initial number of follicles in humans is approximately ten million primordial follicles. By the time a girl reaches menarche, this number has declined to nearly 500,000 and continues to decline thereafter until menopause through the release of a few oocytes during the menstrual cycle, with the majority being lost as a result of atresia. There is limited evidence that ovarian regeneration may occur from stem cells of a variety of sources, including bone marrow [53–55].
In males, spermatogenesis begins in the prepubertal stage. Spermarche (release of spermatozoa) occurs in early to mid-puberty with age-appropriate gonadotropin production, preceding the ability to produce an ejaculate.
25.2.1 Assessing Ovarian Reserve
25.2.1.1 Menstrual Cycles
The presence or absence of menses has traditionally been used as the primary measure of fertility. In the United States, menarche (onset of menstruation) occurs at a median age of 12.43 years [56]. Menopause is the absence of menstrual cycles for 12 consecutive months, with the average age of menopause in the United States being 51 years. Menopause before the age of 40 years is called premature menopause.
Survivors of childhood cancer in the United States had a cumulative incidence of nonsurgical premature menopause of 8 % compared to 0.8 % in their siblings (RR = 13.21, 95 % CI = 3.26–53.51; P < 0.001) [40]. In contrast, in Europe (Euro2K cohort), the median age at menopause among childhood cancer survivors was noted to be 44 years old, with only 2.1 % having nonsurgical premature menopause [43]. Survivors who received both alkylating agents and abdominal-pelvic radiation were more likely to be postmenopausal than were those who underwent surgery alone, and there was a dose correlation of both radiation and alkylating agent and the risks of menopause and infertility [42].
In the perimenopausal period, despite a decreased ovarian reserve or fertility, women may continue to menstruate, suggesting that the presence of regular menstrual cycles does not correlate with intact fertility or ovarian reserve. Adult childhood cancer survivors with regular menstrual cycles and basal FSH <10 IU/l have been noted to have diminished ovarian reserve with smaller ovarian volume, lower number of small antral follicles per ovary, and lower total number of follicles per ovary [41]. Bath et al. found subtle ovulatory disorders in adult female childhood leukemia survivors who had normal menstrual cycles [57]. In addition, 22 % of survivors who suffered chemotherapy-induced amenorrhea were able to have children [58].
25.2.1.2 Antral Follicle Counts (AFC) and Total Ovarian Volume
Antral follicle counts (AFC) and total ovarian volume (TOV) can be measured in adult women through transvaginal ultrasonography. In normal women, AFC, TOV, and chronological age are individually predictive of menopausal status [59] with an age-related decline in both AFC and TOV [60].
In adults, the mean premenopausal ovarian volume is 4.9 ± 0.03 cm3, while the postmenopausal volume is 2.2 ± 0.01 cm3. An evaluation of the ovarian reserve and reproductive lifespan could be assessed using ovarian volume in correlation with the Faddy-Gosden model [61]. In adult survivors of childhood cancer, ovarian volume is reduced compared to controls [41, 62].
The mean AFC declines from 15 in normal women aged 25–34 years to 4 in those aged 41–46 years [63]. In assisted reproduction, AFC predicts ovarian response and pregnancy results with no pregnancy occurring when the AFC is <3 [64]. Adult childhood cancer survivors with normal menstrual cycles have been noted to have fewer small antral follicles and total number of follicles per ovary [41].
In children, AFC and TOV could be performed transabdominally. However, this has not been well studied. No normative values exist and there are very few radiologists skilled in this technique.
25.2.1.3 Endocrine Hormones (FSH, LH, Estradiol [E2])
During the menopausal transition and menopause, decreased ovarian function results in increase in FSH levels, decrease in E2 and inhibin B, and a marked decline in primordial follicle numbers in the ovaries [65–68]. Several studies demonstrate the same findings in select groups of female childhood cancer survivors.
However, the use of FSH, LH, and estradiol in assessing fertility potential of cancer patients is limited by variation with the menstrual cycles and low to undetectable levels in prepubertal children, making it difficult to detect changes with or after cancer therapy.
25.2.1.4 Endocrine Hormone: Inhibin B
Inhibin B is a hormone secreted by granulosa cells of the preantral and early antral follicles in females, which has been investigated as a possible surrogate marker of ovarian reserve in childhood cancer survivors. Crofton et al. [69] showed inhibin B to be suppressed with chemotherapy, though usually transient, in nine prepubertal females. Lower inhibin B levels have also been noted in childhood cancer survivors compared to controls (median, 94 vs. 111 pg/ml; P = 0.03) [70]. Although inhibin B levels decrease with age and during premature ovarian failure, it does not predict the onset of ovarian failure and is a fairly late marker of a reduced follicle pool [71–74]. Its use as a marker is also hampered by variation during the menstrual cycle [75].
25.2.1.5 Endocrine Hormone: Anti-Mullerian Hormone (AMH)
AMH is a hormone produced by the granulosa cells of the secondary, preantral, and early antral follicles. It acts as a follicular gatekeeper, limiting follicle growth and estradiol production from small antral follicles prior to selection [76, 77]. At birth, the serum AMH level is barely detectable and then rises in childhood and adolescence, peaking in a woman’s early 1920s before declining to menopause, correlating positively with nongrowing follicle recruitment [72, 78, 79].
AMH has been increasingly used as a measure of ovarian reserve to assess the gonadotoxic effect of chemotherapy/radiotherapy, especially in children in whom FSH and inhibin B are not useful [80–84]. Compared with other ovarian reserve markers, AMH appears to be the best hormonal marker for ovarian reserve as it has several advantages. AMH levels reflect changes in ovarian function earlier, there is no significant fluctuation during the menstrual cycle, and it is highly predictive for timing of menopause [73, 85, 86]. Serum AMH levels are detectable in healthy females from birth to menopause [87, 88], making it suitable as a marker even in prepubertal girls. However, AMH assays continually evolve and there are no international standards established. In addition, there is limited data correlating AMH and natural fertility at different stages of reproductive life, especially in children and adolescents.
Several studies looking at AMH as a measure of ovarian reserve in cancer survivors have shown promise. In women treated with mechlorethamine, vincristine, procarbazine, and prednisone (MOPP) chemotherapy for Hodgkin lymphoma during childhood, AMH was noted to be lower compared with healthy women and women treated without MOPP [89]. Although the mean AMH concentration in a cohort of childhood cancer survivors was no different from controls, the AMH levels were lower than the 10th percentile of normal values in 27 %, identifying subgroups at risk for decreased fertility or premature ovarian failure [90]. AMH declined in adult women with cancer during treatment followed by recovery in some patients, with the rate of recovery determined by the pretreatment AMH level [83]. Similarly, in two small cohorts of female childhood cancer patients 0–18 years old, AMH was detectable across the age range with note of a progressive decline during chemotherapy, regardless of AMH at diagnosis, age, menarche, or treatment given. The degree of recovery depended on the gonadotoxicity of the treatment given [91, 92]. Although promising, more long-term data is needed to ascertain the use of AMH to predict long-term ovarian function after cancer therapy as well as evaluate fertility preservation strategies.
25.2.2 Assessing Testicular Reserve
25.2.2.1 Semen Analysis
Semen analysis is the gold standard for assessing fertility status in the male. It is an easy, cost-effective, and noninvasive test to determine fertility potential in the male at any stage following puberty. Although useful, it is an imperfect tool. It cannot be used in prepubertal males. Controversy remains on what constitutes as “normal” spermatozoa in semen. Current analysis is guided by the 5th edition of the World Health Organization manual [93]. Routine semen analysis includes semen volume and viscosity, sperm concentration, sperm motility, and morphology.
Varying chemotherapeutic regimens can cause temporary (with recovery within 24 months) or permanent oligo- or azoospermia [20, 22, 23, 27, 33, 94]. Of the chemotherapeutic agents, the effect of alkylators (especially cyclophosphamide) on semen has been the most extensively studied. Similarly, radiation effects may also recover within 24 months, depending on dose and site of radiation.
Other sperm function tests (such as sperm-cervical mucus interaction, tests of sperm capacitation, tests of hemizona and zona pellucida binding, sperm penetration assay, tests of sperm DNA damage, assessment of reactive oxygen species, and sperm proteomics) are available but not routinely used clinically [95–97].
25.2.2.2 Endocrine Markers: FSH, LH, Testosterone, and Inhibin B
Levels of FSH have been shown to be inversely correlated to testicular spermatogenic function. Azoospermic patients are found to have an elevated basal FSH [98]. Male childhood cancer survivors have higher FSH and LH and lower testosterone levels than controls [19, 21, 27]. Testosterone can be normal prepubertally and drop postpubertally [13, 14]. Although an elevated basal FSH level gives a good indication of testicular damage, normal levels do not rule out azoospermia [15].
Inhibin B is a hormone secreted by the Sertoli cells. More recently, inhibin B has been used and found to be low in male childhood cancer survivors compared to sibling controls [18]. Lower levels of inhibin B have been noted in males after chemotherapy and radiotherapy, such as after treatment with MOPP (mechlorethamine, vincristine, procarbazine, prednisone) chemotherapy for Hodgkin lymphoma [99], with inhibin B showing an independent correlation with sperm concentration. However, others show that inhibin B alone does not reflect spermatogenesis as well as inhibin B in combination with FSH in childhood cancer survivors [100]. More conflicting results have recently surfaced. In the St. Jude Lifetime Cohort Study, it was noted that although serum inhibin B is directly correlated with sperm concentration, neither inhibin B nor FSH nor their ratio is adequate for distinguishing between azoospermic and nonazoospermic long-term survivors of childhood cancer [101].
25.2.2.3 Guidelines for Assessing Ovarian and Testicular Reserve
The Children’s Oncology Group (COG) has put together the only available guideline regarding the assessment of ovarian and testicular reserve in long-term survivors of childhood, adolescent, and young adult cancer (http://www.survivorshipguidelines.org/).
In females, the recommendations include checking pubertal (onset and tempo), menstrual, and pregnancy history annually as well as Tanner staging annually until a patient is sexually mature. Baseline FSH, LH, and estradiol are checked at age 13 and as clinically indicated in patients with delayed or arrested puberty, irregular menses, primary or secondary amenorrhea, and/or clinical signs and symptoms of estrogen deficiency [Children’s Oncology Group Long-Term Follow-Up Guidelines for Survivors of Childhood, Adolescent and Young Adult Cancers, version 4.0. October 2013]. There are currently no guidelines available on the use of AFC, TOV, and AMH.
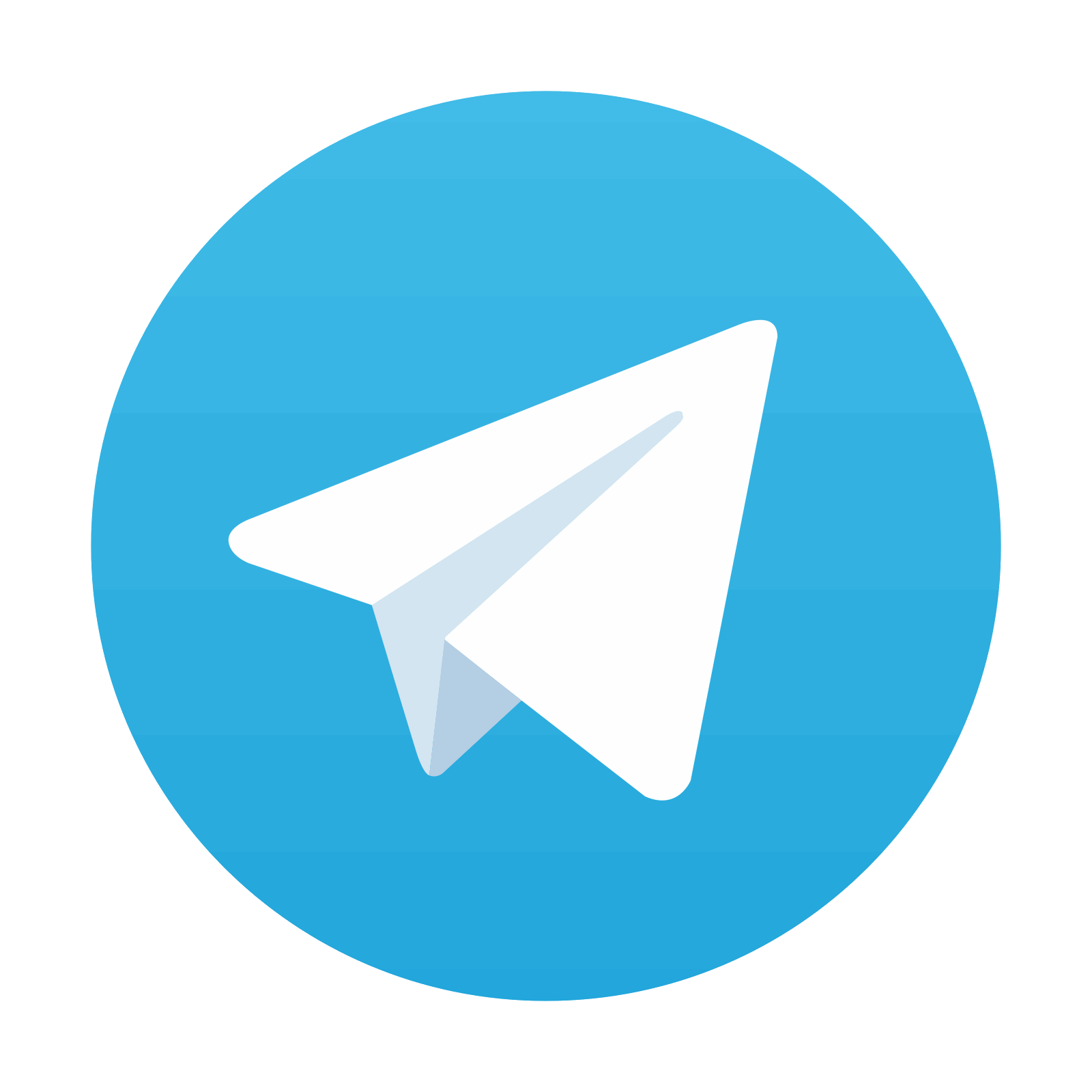
Stay updated, free articles. Join our Telegram channel

Full access? Get Clinical Tree
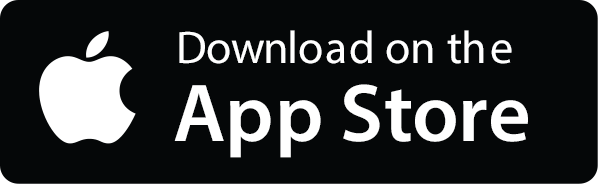
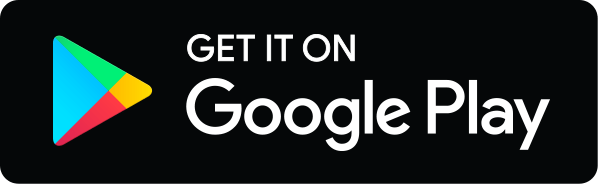