GC-MS
Sensor array
Compounds
Volatile and semi-volatile compounds
Tunable through choice of sensors
Accuracy of compound identification
Very high
No compound identification
Detection limit
~ppm v , can be improved to ~ ppb v through sample pre-concentration
Depends on sensor type; tunable for specific VOC mixtures
Compound quantification
Requires calibration
n/a
Speed
Off-line
High
Required user skill level
High
None
Sample preparation
Pre-concentration of breath VOCs necessary
None
Possibility of direct breath sampling
No
Yes
Breath print/VOC pattern determination
Separate statistical treatment of VOC concentration profiles; requires quantification
Directly through built-in statistical treatment of the collective sensing signals
Size of equipment
Typically very large
Small, portable
Maintenance
High
Low
Consumable costs per sample
$40–$150
$1–$5
Collective breath VOC patterns can, in principle, be derived through additional statistical analysis of the concentration profiles of preselected VOCs, but the entire process is tedious and time-consuming. To summarize, analytical chemical analysis of the exhaled breath may yield highly accurate concentration profiles of separate BC-specific VOCs that provide interesting input for studying biochemical pathways of BC. However, the experimental procedures involved would not be practical for real-world diagnostic or BC screening.
The second approach consists of the direct detection of collective breath VOC patterns (without actually identifying the constituent compounds), using arrays of broadly cross-reactive sensors [66, 67]. These patterns have been termed breath prints. Sensor arrays mimic the mammalian sense of smell and are therefore often called electronic noses. Each sensor in the array responds to all or part of the VOCs in the breath sample. Breath prints are then derived from the collective numerical output of the sensors that interact with the breath VOCs, using methods of statistical data analysis. The breath print BC markers are dimensionless parameters. This approach avoids expensive equipment and, therefore, has realistic potential for future fast, cost-effective, and high-throughput breast cancer diagnostics. Pre-concentration is generally not necessary, because additive signals are monitored that stem from a wide range of breath VOCs, at total concentrations of at least ppmv or above. In principle, this approach can be adapted for direct sampling of patients’ breath, but until now, indirect sampling of pre-collected breath samples has been more feasible, since the sensor arrays are usually operated in a research laboratory. Sensor arrays are therefore ideally suited for direct BC marker breath printing [12, 14]. Types of sensors that have been used for breath printing will be described in section “Sensor Arrays for BC Marker Breath Printing.” However, breath printing is essentially a black box approach to chemical sensing, which bears the risk of over-fitting small data sets during the statistical analysis [30]. Therefore, careful validation of the study results, preferably with a blinded validation sample set, should be an integral part both of limited proof-of-concept studies and of large-scale clinical trials.
Challenges on the Way to Clinical Practice
Breath VOCs that might indicate cancer have attracted much research interest during the past decades; however, limited preliminary results on BC have been achieved both in the chemical analysis of exhaled breath and in the sensor-based breath printing (see Tables 23.2, 23.3, and 23.4). Due to the complexity of the breath collection process and analysis and the insufficient attention to confounding factors, no viable and generally accepted BC markers have yet been established, and BC breath markers are still entirely confined to research. The lack of standardization pertains to each step of the multistep process toward the establishment of reliable BC markers from exhaled VOCs. Figure 23.1 provides an overview of the breath BC marker development—from bench to bedside. Inconsistent findings of different study groups in limited pilot trials could be attributed to one or more of the following problematic aspects of current breath marker research:
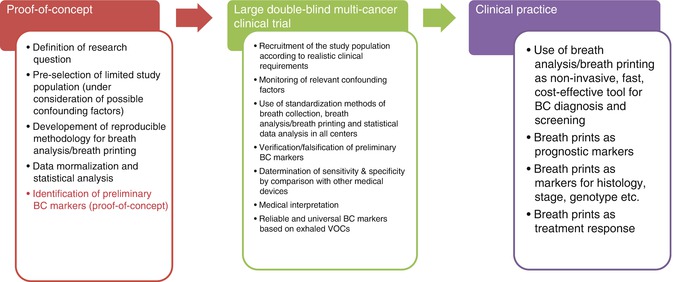
Table 23.2
Examples for experimental studies of BC-specific VOC patterns, using different analytical techniques
Determination of VOC pattern | Study population | Obtained results | Future potential as marker for | Principal investigator | |||||||||
---|---|---|---|---|---|---|---|---|---|---|---|---|---|
Analytical method | VOC as input for algorithm | Statistical algorithm | Validation | Target | Control 1 (confirmed by mammography/biopsy) | Control 2 (healthy volunteers) | Control 1 | Control 2 | Breast malignancy | Histology | |||
Sensitivity (%) | Specificity (%) | Sensitivity (%) | Specificity (%) | ||||||||||
TD/GC-MS | 8 VOCs | Forward stepwise discriminant analysis | Cross-validation | 51 BC | – | 42 | 88.2 | 73.8 | – | Phillips et al. [16], #144 | |||
10 VOCs | Forward stepwise discriminant analysis | Cross-validation | 52 BC | 50 | – | 60.8 | 82 | – | Phillips et al. [16], #144 | ||||
5 VOCs | Fuzzy logic | Prediction set | 51 BC | 42 | 93.8 | 84.6 | – | Phillips et al. [17], #143 | |||||
10 VOCs | Weighted digital analysis (WDA) | Prediction set | 54 BC | 204 | – | 75.3 | 84.8 | – | – | – | Phillips et al. [15], #150 | ||
5VOCs | – | t test and Mann-Whitney U test | 10 BC | – | 10 | – | – | n/a | n/a | x | – | Mangler et al. [19], #159 | |
SPME/GC-MS | 5 VOCs | Forward stepwise discriminant analysis | 14 BC | – | 22 | – | – | n/a | n/a | x | – | Peng et al. [14] |
Table 23.3
Breath VOCs as potential BC markers
Study | Analytical method | VOC | Chemical class | Possible source | Principal investigator |
---|---|---|---|---|---|
1 | TD/GC-MS | Nonane | Alkane | Markers of oxidative stress named: breath methylated alkane contour (BMAC) | Phillips et al. [16] |
Tridecane, 5-methyl | Methylated alkane | ||||
Undecane, 3-methyl | Methylated alkane | ||||
Pentadecane, 6-methyl | Methylated alkane | ||||
Propane, 2-methyl | Methylated alkane | ||||
Nonadecane, 3-methyl | Methylated alkane | ||||
Dodecane, 4-methyl | Methylated alkane | ||||
Octane, 2-methyl | Methylated alkane | ||||
2 | 2-Propanol | Alcohol | n/a | Phillips et al. [17] | |
2,3-Dihydro-1-phenyl-4(1H)-quinazolinone | Ketone | Antitumor activity | |||
1-Pentyl-ethanone | Ketone | Anti-invasive activity against human MCF-7/6 mammary carcinoma cells | |||
Heptanal | Aldehyde | Cancer biomarker | |||
Isopropyl myristate | Ester | n/a | |||
3 | Cyclopropane, ethylidenea | Diene | n/a | Phillips et al. [15] | |
Cyclotetrasiloxane, octamethyla | Siloxane | Exogenous | |||
D-Limonene 5989-27-5a | Liquid alkane | Ingested from foodstuffs | |||
Benzene, 1,2,4,5-tetramethyla | Benzene derivative | Environmental pollutant | |||
Tridacanea | Alkane | Oxidative stress | |||
Dodecane, 2,7,10-trimethyla | Methylated alkane | Oxidative stress | |||
Tetradecanea | Alkane | Oxidative stress | |||
(+)-Longifolenea | Oily liquid alkane | n/a | |||
2-Hexyl-1-octanola | Alcohol | n/a | |||
2,5-Cyclohexadiene-1,4-dione, 2,6-bis(1,1-dimethylethyl)-a | Ketone | n/a | |||
4 | 3-Methylhexane | Methylated alkane | Altered activity of cytochrome p450 | Mangler et al. [19], #159 | |
Decane | Alkane | Altered activity of cytochrome p450 | |||
Caryophyllene | Terpenes | Altered activity of cytochrome p450 | |||
Naphthalene | Polycyclic aromatic hydrocarbon | Altered activity of cytochrome p450 | |||
Trichlorethylene | Halogenated hydrocarbon | Tumor cell metabolites | |||
5 | SPME/GC-MS | 3,3-Dimethyl pentane | Methylated alkane | Oxidative stress | Peng et al. [14] |
2-Amino-5-isopropyl-8-methyl-1-azulenecarbonitrile | Nitrile | n/a | |||
5-(2-Methylpropyl) nonane | Methylated alkane | Oxidative stress | |||
2,3,4-Trimethyl decane | Methylated alkane | Oxidative stress | |||
6-Ethyl-3-octyl ester 2-trifluoromethyl benzoic acid | Ester | n/a |
Table 23.4
Examples for experimental studies of BC breath prints, using sensor arrays
Sensor array | Study population | Sensitivity (%) | Specificity (%) | Future potential as marker for | Principal investigator | |||||||||||
---|---|---|---|---|---|---|---|---|---|---|---|---|---|---|---|---|
Sensor type | Sensing material | Statistical algorithm | Target | Control 1 (benign breast condition) | Control 2 (healthy volunteers) | Control 3 (clear mammography) | Breast malignancy | Distinction from other breast deficiency | Distinction from other malignancy | Staging | Histology | Treatment response | Genotype | |||
Chemiresistors | GNP | PCA | 13 | – | 22 | – | n/a | n/a | x | n/a | x | x | x | n/a | n/a | Peng et al. [14] |
GNP + cubic Pt NP | PCA + SVM | 11 | 14 | – | 7 | 94 | 80 | x | x | – | x | x | n/a | n/a | Shuster et al. [12] | |
GNP + cubic Pt NP | PCA + SVM | 11 | – | 21 | – | n/a | n/a | x | – | – | x | x | n/a | n/a |
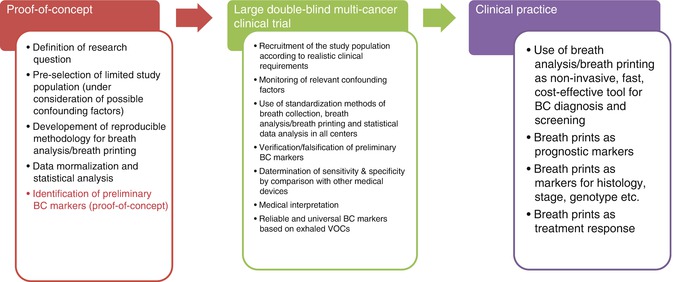
Fig. 23.1
Steps toward the development of reliable VOC-based breath BC markers for clinical practice—from bench to bedside. BC breast cancer, VOC volatile organic compound
1.
Inconsistencies in the preselection of the rather small control groups used in the proof-of-concept clinical studies. For example, control groups might consist of healthy biopsy-proven, benign breast tumors, age-matched groups, hospital personnel, relatives and spouses of the patients, etc. Clinical studies investigating exhaled VOCs of BC, especially proof-of-concept studies of limited size, should be carefully designed to avoid biased results, by using well-matched study populations.
2.
Inconsistent breath sampling and VOC pre-concentration procedures used in various studies (see section “Breath Collection, Sample Storage, and Possible Sources of Contamination”). Breath collection should be standardized, and either one universally accepted method should be adapted by all researchers in the field or a small number of well-defined techniques should be established for use. In addition, a breath collection protocol should be followed which minimizes the effect of nutrition, smoking habits, and medication.
3.
Use of different analytical methods including different equipment (e.g., GC-MS (42, 48), PTR-MS (25, 37), etc.). See Tables 23.1 and 23.2 and section “Chemical Analysis: Identification of Specific BC Marker VOCs.” Note that the identification of the VOCs by GC-MS or PTR-MS is not 100 % certain, even if the identification by spectral library match and retention time for GC-MS is quite reliable [22, 24, 48].
4.
Use of different sensor arrays for breath print detection. Special attention must be paid to confounding factors when using sensor arrays. The response of the sensor array to the relevant confounding factors should be studied in order to exclude biased results.
5.
Inconsistencies in the normalization procedures of the raw data. While part of the studies normalized the data according to the concentration of a specific VOC in the exhaled breath [14], other studies have normalized the data according to the difference between the concentrations in the exhaled and the inhaled air [15–17]. Non-normalized data were reported as well. Normalization for sensor arrays is even more challenging, because long-term and short-term sensor drifts have to be considered [68].
6.
Inconsistent data analysis. For instance, the analysis of the GC-MS raw data includes identification, separation, and area integration of the peaks in the chromatograms for each sample, as well as quantitative comparisons of the chromatogram peak areas or compound concentrations between different study groups, using statistical algorithms. Patterns distinguishing the study groups may be obtained from the collective GC-MS results through a variety of supervised or non-supervised statistical pattern recognition algorithms. For example, forward stepwise multi-linear regression, a supervised method, was used by Phillips et al. [16] in order to establish BC patterns based on (unidentified) chromatogram peaks. VOC patterns of BC were studied using non-supervised methods such as principal component analysis [12]. Also, the data analysis of the collective sensor array output involves multivariate statistical analysis of the raw data.
7.
No in vitro studies have been performed. In vitro studies may serve as a way to eliminate confounding factors and, thus, will allow immediate testing to predict the clinical benefit from targeted therapy straight from the tissue. This approach could help guide treatments by tracking genetic alterations from the frozen tissue with no time or money limitations.
The following sections are designed to provide the reader with a detailed understanding of the key components of the experimental process in state-of-the-art breath BC marker research that may affect the results of experimental studies: Different methods of breath sample collection with their strength and limitations are discussed below; methods of analytical breath VOC analysis, together with selected studies, are presented in section “Chemical Analysis: Identification of Specific BC Marker VOCs;” and sensor arrays that were developed for and/or have been applied to BC marker breath printing will be described in section “Sensor Arrays for BC Marker Breath Printing.”
Breath Collection, Sample Storage, and Possible Sources of Contamination
Exhaled breath samples are rather delicate. Special attention should be paid during sample collection and storage:(1) to preserve the highly volatile disease markers and (2) to avoid contamination with confounding or environmental VOCs from external sources. The study of biomarkers in exhaled breath still suffers from a lack of standardization of the breath collection and analysis. Amann and coworkers have recently proposed a standardization of the breath collection process that might be generally accepted in the future [69]. Several different procedures of direct and indirect breath collection are currently being used. Sampling procedures include, but are not limited to, mixed expiratory breath collection, end-tidal breath collected with CO2-controlled sampling [36], sampling with Tedlar or Mylar bags [41], and portable breath collection apparatus (BCA), which was developed and used by Phillips et al. [70].
Direct and Indirect Breath Sampling
During direct breath sampling, air exhaled by the subject is introduced into the measuring system without any intermediate steps [47]. This approach would be most convenient for a future clinical device. Breath print analyzers based on sensor arrays could, in principle, be adapted to direct sampling. In contrast, direct sampling cannot be used in combination with the most important method of chemical analysis (GC-MS). During indirect sampling, the exhaled air is stored on an adequate medium and analyzed later. Indirect sampling is still by far more widely used method in research settings, both for chemical analysis of breath and sensor array studies.
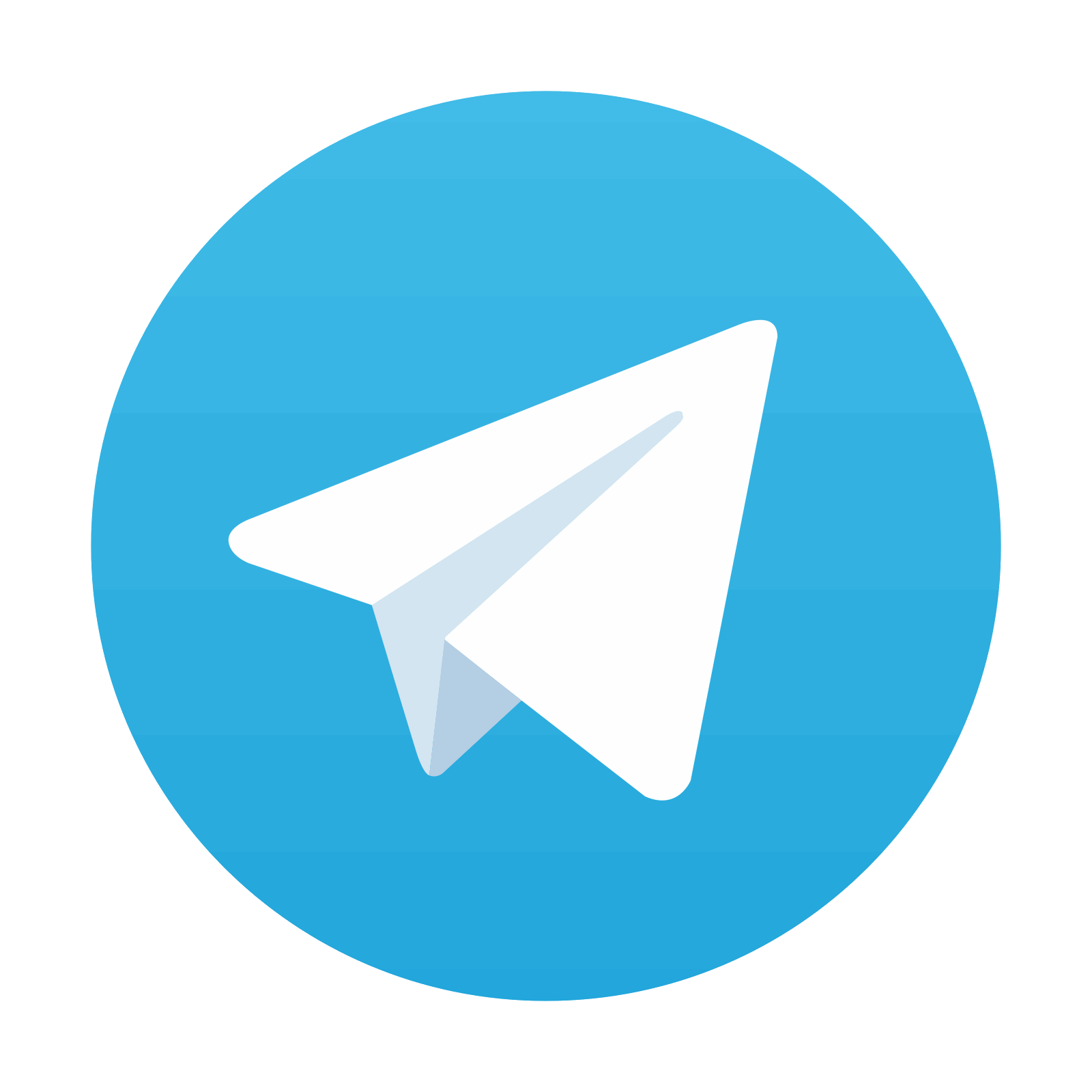
Stay updated, free articles. Join our Telegram channel

Full access? Get Clinical Tree
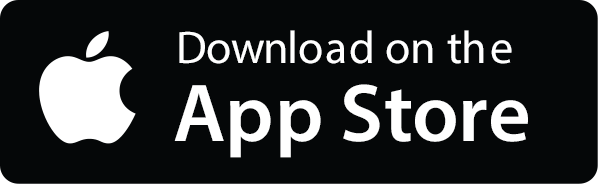
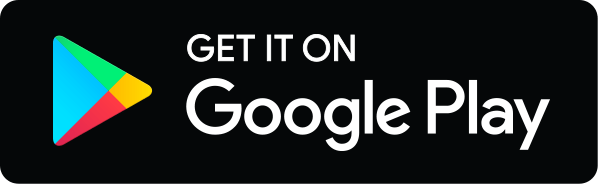