where Hn is the venous haematocrit of the recipient’s own red cells after n operations. The total PCV after n operations is calculated by:
The first term represents the patient’s residual red cells and the second term the donor’s red cells (Veall and Mollison 1950).
When the method of intermittent substitution is used for plasma exchange, a similar formula can be derived for calculating the rate of exchange (Veall and Mollison 1950; Mollison et al. 1950) (see also ‘Therapeutic plasma exchange’, below).
Sophisticated equations are not necessary in practice. Removal of most blood components follows a logarithmic curve (Figure 17.1) (McCullough and Chopek 1981). This model assumes that the component removed is neither synthesized nor degraded substantially during the procedure, remains within the intravascular compartment and mixes instantaneously and completely with any replacement solution. When the goal of plasmapheresis is to supply a deficient substance, for example plasma factors in the treatment of thrombotic thrombocytopenic purpura, replacement follows logarithmic kinetics similar to those developed for solute removal. From Figure 17.1, it should be evident that removal of 1.5–2 volumes will reduce an intravascular substance by about 60% and that processing larger volumes results in little additional gain.
Figure 17.1 Relation between volumes removed by apheresis and percentage of the target component remaining. The relation is valid for blood volumes during red cell exchange or for plasma volumes during plasmapheresis if the target solute remains primarily within the intravascular space.
(Source: Adapted from Chopek and McCullough (1981) Lab Med 12: 745. Reproduced with permission of The American Society for Clinical Pathology.)
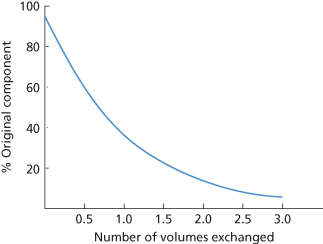
Indications for Red Cell Exchange (Erythrocytapheresis)
Sickle Cell Disease
Red cell exchange (erythrocytapheresis) is used most often to manage or prevent the acute vaso-occlusive complications of sickle cell disease. Automated blood cell separators are faster and easier than manual exchange transfusion (Klein et al. 1980; Klein 1982). Most new instruments calculate the exchange conditions after the patient’s laboratory and clinical data are entered into a pre-programmed exchange protocol. A single volume exchange will remove about two-thirds of the circulating cells.
Sickle cell anaemia occurs in individuals who are homozygous for a single mutation in codon 6 of the beta globin gene, resulting in substitution of a single amino acid. Although the defect appears simple, the genetics may be further complicated by other polymorphisms in the haemoglobin molecule or mutations in control of chain synthesis. The pathophysiology of the vaso-occlusive crises is even more complex, involving haemoglobin polymerization, change in cell shape, adhesion to endothelial cells, release of inflammatory cytokines and nitric oxide binding by free haemoglobin (Kaul et al. 1996, 1998, 2000; Kato et al. 2007). Clinical manifestations vary from patient to patient and there may be distinct clinical phenotypes (Alexander et al. 2004). The rationale behind exchange transfusion involves improving tissue oxygenation, suppressing erythropoiesis and consequent haemolysis of sickling cells, and preventing microvascular sickling by diluting the patient’s abnormal red cells, while simultaneously correcting anaemia and favourably altering whole blood viscosity and rheology. No clinical data support a single optimal level of haemoglobin A (HbA); however, as few as 30% of transfused cells markedly decrease blood viscosity; at mixtures of > 50%, resistance to membrane filterability approaches normal (Anderson et al. 1963; Lessin et al. 1978; Kurantsin-Mills et al. 1988). In non-emergency situations, such levels can often be achieved with a simple transfusion regimen. For simple and exchange transfusions, raising the level of HbA to 60–70% while lowering the level of HbS to 30% is generally efficacious, although even higher levels of HbA may be required to treat an ongoing crisis. The percentage of HbA in a sample of patient’s blood may continue to rise after transfusion as the short survival of the sickle cells results in a survival advantage for the transfused cells.
Clinical indications for exchange transfusion in patients with sickle cell anaemia remain controversial. Limited controlled study data are available. Simple transfusion has been shown to improve renal concentrating ability and splenic function in young sickle cell patients; exchange transfusion improves exercise tolerance and reverses the periodic oscillations in cutaneous blood flow associated with this disease (Keitel et al. 1956; Pearson et al. 1970; Miller et al. 1980; Rodgers et al. 1984). Such observations have encouraged the use of exchange transfusion for acute complications of sickle cell disease such as chest syndrome, priapism, cerebrovascular accident, and hepatic and retinal infarction. Exchange transfusion for sickle cell patients has also been used for prophylaxis during pregnancy and before surgery, although prophylactic transfusion in these settings is controversial. The only randomized trial of transfusion during pregnancy has shown that prophylactic transfusion sufficient to reduce the incidence of painful crises did not reduce other maternal morbidity or perinatal mortality (Koshy et al. 1988). In a randomized study of sickle cell disease patients undergoing surgery, a conservative simple transfusion regimen to increase the haemoglobin level to 10 g/dl was as effective as an aggressive regimen (to lower the HbS level to < 30%) with respect to perioperative non-transfusion-related complications (Vichinsky et al. 1995). The patients in the aggressive regimen group received twice as many units of blood, had a proportionally increased red blood cell alloimmunization rate and suffered more haemolytic transfusion reactions. A subsequent trial has confirmed that preoperative transfusion is associated with decreased perioperative complications in patients with sickle-cell disease who are scheduled to undergo low-risk and medium-risk surgeries (Howard et al. 2013).
Transfusion prophylaxis is now clearly indicated for children at high risk for stroke. In addition to symptomatic stroke, silent infarcts (SCI) occur in 27% of children prior to the sixth birthday, and 37% by the fourteenth (Kwiatkowski et al. 2009; Bernaudin et al. 2011).
In adults, the frequency and consequences of SCI are less well defined. A randomized controlled study demonstrated a risk reduction of stroke by 90% in patients who were maintained at levels of HbS < 30% by simple or exchange transfusion (Adams et al. 1998). This result confirms earlier experience (Russell et al. 1984) and indicates that in this group of children with sickle cell anaemia, transfusion therapy should begin before the first event and continue indefinitely. Cessation of transfusion therapy results in recurrence of stroke and progression of central nervous system disease, although SCI continue at a reduced frequency despite regular transfusion (Abboud et al. 2011). Treatment with hydroxyurea is not a suitable substitute. Long-term erythrocytapheresis may be preferable for patients at high risk for stroke who have developed iron overload to levels associated with organ damage (Kim et al. 1994). However, patients remain in positive iron balance, although iron accumulation is slow and chelation is rarely required to prevent transfusional haemosiderosis. Use of exchange transfusion for adults is extrapolated from the paediatric experience, as no equivalent systematic data have been collected.
Exchange transfusion, although relatively safe, carries all the complications of red cell transfusion. Patients are exposed to a large number of donors and are at a small but significant risk of contracting blood-borne infections (see Chapter 16). As many as 33% of all patients develop alloantibodies, and life-threatening delayed haemolytic transfusion reactions have been reported (Diamond et al. 1980; Coles et al. 1981). In addition, a study of multiply transfused sickle cell patients found that 85% of heavily transfused patients had been alloimmunized to HLA and/or platelet-specific antigens (Friedman et al. 1996). Extended red blood cell phenotyping at diagnosis and provision of phenotypically matched blood can reduce the risk of red cell alloimmunization and associated haemolytic transfusion reactions (Rosse et al. 1990; Vichinsky et al. 1990; Castro et al. 2002). However, providing extended matching may be difficult for chronic transfusion programmes, and a reasonable compromise concentrates on matching for the most immunogenic, clinically important antigens, D, E, K and C.
Haemolytic Disease of the Newborn
See Chapter 12 and Appendix 13.
Exchange Transfusion in Other Conditions
Other indications for red cell exchange are rare. The procedure has been used for patients with overwhelming red cell parasitic infections, such as severe and complicated malaria and babesiosis (White 1996). In these situations, red cell exchange decreases the concentration of circulating parasites and may help sustain life until conventional therapy and natural immunity take effect. Although the efficacy of this therapy has not been evaluated by controlled trials, prospective studies and review of published cases consider erythrocytapheresis prudent when parasitaemia exceeds 10–15%, and even at lower levels in selected patients (Powell and Grima 2002; Griffith et al. 2007). Automated red cell removal with volume replacement (isovolaemic haemodilution) can be performed rapidly and safely in polycythaemic subjects. This manoeuvre should be reserved for polycythaemic patients with an urgent clinical indication to lower the haematocrit (e.g. evolving thrombotic stroke) for which standard single unit manual phlebotomy might be inadvisably slow. Automated double red cell apheresis technology has been used more recently to treat individuals with hereditary haemochromatosis (Leitman et al. 2003a) (see Chapter 1). This procedure removes excess iron more rapidly than does manual phlebotomy, and may be more tolerable to patients due to the lower frequency of maintenance procedures required. Red cell exchange is occasionally indicated when a patient is found to have been recently transfused with incompatible red cells or as an adjunct to Rh-Ig therapy when a D-negative woman of child-bearing age who is not already immunized to D has been inadvertently transfused with a large volume of D-positive cells.
Plasmapheresis
A Short History of Plasmapheresis
John Jacob Abel in 1914 coined the term plasmapheresis, from a Greek verb meaning ‘to take away or withdraw’, to describe removal of plasma and return of the remaining blood components to the donor. Abel and colleagues (1914) described the technique of plasma removal as an investigational treatment for toxaemia in nephrectomized dogs, but foresaw a variety of additional potential applications. Their studies, like those of contemporaries in Germany and Russia, relied upon cumbersome phlebotomy, centrifugation and resuspension techniques, as well as anticoagulation with the leech-derived protein, hirudin. The results of Abel and colleagues were clouded by technique-related deaths of several experimental animals.
The first manual plasmapheresis procedures in humans were likewise conducted for experimental purposes to determine the normal rate of protein regeneration in six volunteer donors (Tui et al. 1944). The procedure proved safe, although one volunteer experienced a pyrogenic reaction, most likely related to contamination of the reusable glass bottle system that was employed for blood donation in the 1940s. A similar manual technique was put into practice by Grifols-Lucas (1952), the first use of an apheresis procedure to provide components for transfusion. Whole blood was removed from the donor, and red cells were either allowed to sediment by gravity or packed by centrifugation, removed and returned to the donor, often as long as a week after the initial phlebotomy. In the same report, Grifols-Lucas described the use of manual plasmapheresis to treat a few patients with hypertension and recorded what is arguably the first placebo effect with therapeutic apheresis, ‘striking subjective improvement, which, however, is not matched by a corresponding betterment of the objective symptoms’. A further refinement of manual plasmapheresis relied upon a closed-system vacuum bottle technique to remove plasma from a patient with macroglobulinaemia (Adams et al. 1952).
Although the early manual methods demonstrated the feasibility of plasmapheresis for collecting components and for therapy, glass bottles proved awkward, and reusable equipment raised concern about contamination with bacteria and pyrogens. With the introduction of sterile, disposable, interconnected plastic blood bags, plasmapheresis became relatively safe and easy (Skoog and Adams 1959). The integral-bag procedure required only minor modifications in centrifuge technique for single-donor platelet collections (Kliman et al. 1961). However, manual apheresis proved too slow, inefficient and labour intensive for large component volumes and engendered concerns that the separated units of red cells might be returned accidentally to the wrong donor or patient, with potentially fatal consequences (Cumberland et al. 1991). The introduction of automated on-line blood cell separators solved these problems.
Automated on-line blood processing arose from the seminal studies of Edwin J Cohn and collaborators, who developed an ex vivo refrigerated, sealed-bowl system for centrifugal separation of blood components (Tullis et al. 1956). This system evolved into a single-arm instrument capable of separating plasma or cellular blood elements in a plastic, disposable bowl and returning the unwanted components to the donor in a batch-processing fashion (Latham 1986). Almost concurrently, a scientific team from the IBM Corporation and the National Cancer Institute was developing a continuous-flow instrument, modelled after the first mechanical heart–lung oxygenator, for collecting granulocytes and platelets from blood donors (Freireich et al. 1965; Jones 1988). These early instruments had reusable parts, rotating seals on the centrifuge bowls and numerous rings and external connections. A system that eliminates seals for continuous-flow centrifuges has been designed and is now used by several manufacturers (Ito et al. 1975).
Although centrifugal cell separation was the earliest and most widely used apheresis technique, automated equipment was also designed to collect granulocytes by filtration or, more accurately, by granulocyte adhesion to nylon filters (Djerassi and Kim 1977). Problems with haemocompatibility and donor reactions led to the abandonment of this technique. However, the success of haemodialysis and the desire to effect more selective separation of plasma proteins spurred further development of filtration and column technology.
Plasmapheresis Vs. Plasma Exchange
When relatively small amounts of plasma, for example 500–650 ml, are removed, without infusing any fluid as a replacement, or infusing only saline, plasmapheresis is the appropriate term for the whole operation. When more than this amount of plasma is removed it becomes advisable to infuse a crystalloid or colloid such as albumin or, occasionally, plasma to replace part or all of the lost plasma protein. This operation constitutes plasma exchange.
The need for plasma for fractionation is still greater than the need for red cells. As a consequence, plasmapheresis is used in some countries, such as the USA, as a method of collecting fresh plasma from normal donors for fractionation into plasma derivatives. Plasma from particular donors, for example donors hyperimmunized to Rh D, tetanus or HBsAg, needed to obtain specific immunoglobulins, is also collected by apheresis. As described in a later section, plasma exchange is carried out for therapeutic purposes with the object of removing some particular constituent from a patient’s plasma.
Plasmapheresis: from Plastic Packs to Blood Cell Separators
In early practice, whole blood was drawn into a primary pack with anticoagulant and a series of attached satellite packs. The blood was centrifuged while the intravenous line was maintained with a saline drip. The plasma or platelet-rich plasma (PRP) was extracted and retained, and the red cells were returned to the donor. The whole procedure was repeated (‘double plasmapheresis’), yielding a total of approximately 500 ml of citrated plasma. With modern refrigerated centrifuges, double plasmapheresis can be completed in less than 1 h., however manual plasmapheresis is cumbersome and carries additional hazards, the most serious of which is that red cell units may be interchanged so that a donor suffers a haemolytic transfusion reaction after receiving the incorrect unit (Cumberland et al. 1991). The development of automated closed-system instrumentation has rendered the manual procedure obsolete.
The advantages of automated apheresis are that (1) donors are never disconnected from their own blood, thus eliminating the risk of introducing the red cells or other blood components from another donor, (2) the speed of collection is considerably faster than with manual apheresis, (3) the procedure is preferred to manual apheresis by donors and (4) the total extracorporeal volume at any one time is less than in double (manual) plasmapheresis. A major advantage is the use of totally disposable harnesses, the majority of which are available prepacked as sterile, closed systems.
Several machines have been designed exclusively for plasma removal based on the principle of either separation of blood components by centrifugation or filtration through a spinning cylindrical membrane. Cell separators use special sterile tubing preconnected to the centrifugation receptacle, containers with fluids (anticoagulant, saline) and collection bags, all known as the harness or ‘kit’. With modern instruments, the anticoagulant is metered in an automated way, often controlled by a microprocessor.
Centrifugal separation involves either continuous or discontinuous flow. Both systems allow centrifugation of the blood without twisting or kinking of the external tubing connected to the moving centrifuge parts either by the use of a rotary seal with one face stationary and the other moving, or by using a flexible jump-rope type of seal-less connector based on the Adams principle (Ito et al. 1975; Stong 1975; Suaudeau et al. 1978). In the discontinuous system, following the separation and removal of the plasma, centrifugation stops while the cells are returned to the donor (Figure 17.2). Depending on the size of the bowl, approximately 250–500 ml of blood is processed with each cycle. The cycles are repeated as often as necessary to obtain the required volume of platelet-poor plasma (PPP). The disadvantages of the discontinuous system are slower performance and greater extracorporeal volume; the main advantages, which make the system ideal for plasmapheresis, are simplicity and the need for a single venous access.
Figure 17.2 Cross-section of an intermittent-flow cell separator bowl (Haemonetics Model A-30 Latham bowl), redrawn from a diagram supplied by the Haemonetics Corporation. Blood is fed into the bottom of a disposable, polycarbonate, rotating bowl and, after separation of the components of blood into layers, different fractions may be harvested sequentially into separate plastic bags. The required fraction, for example that containing the platelets, is retained for transfusion to a patient and the remaining components are returned to the donor. The whole operation may then be repeated.
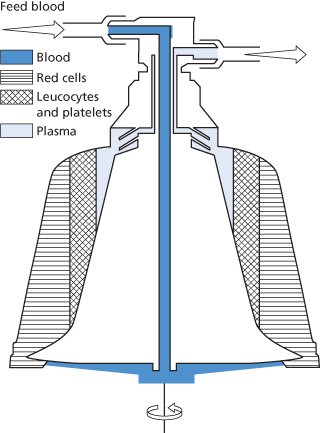
Effects of Intensive or Long-Continued Plasmapheresis
Plasmapheresis of donors may be performed at three levels (WHO 1989b): (1) The donor gives plasma at the same frequency as that allowed for whole blood; (2) the volume of plasma taken and the frequency of donations are two or three times that allowed for routine donations; and (3) 1000–1200 ml of plasma are collected weekly, reaching an annual volume of 50–60 litres of plasma per donor, depending on whether the weight of the donor is below or above 80 kg. With this programme, protein levels do not return to initial values although, in most healthy well-nourished donors, levels remain within the normal range.
Intensive plasmapheresis equivalent to levels 2 and 3, and long-term plasmapheresis, appear to be well tolerated in healthy subjects (Kliman et al. 1964; Cohen and Oberman 1970; Friedman et al. 1975). However, when 1000 ml of plasma is removed weekly for long periods, if equilibrium is to be maintained, an extra 6 g of albumin and 2 g of immunoglobulin G (IgG) must be synthesized daily. These amounts are considerable in relation to the normal production of these proteins, estimated at approximately 12 g of albumin and 2 g of IgG per day (Schultze 1966).
In the US, plasma donors, depending on weight, may donate up to 800 ml twice a week for as many as 104 donations annually. The maximum amount of plasma that may be donated is 50–60 litres a year according to regulations in the US, but only 15 litres per year in Europe. As discussed above, when 1 litre is donated each week, albumin synthesis must increase by 50% if equilibrium is to be maintained. This figure seems uncomfortably close to the maximum possible increase in albumin synthesis which, in both humans and experimental animals, seldom exceeds 100%. Subjects who donate 1 litre of plasma per week lose as much albumin as does a patient with nephrotic syndrome and severe proteinuria (Lundsgaard-Hansen 1977a). However, long-term intensive donor plasmapheresis appears to be safe. Twenty-one plasma centres recruited 3783 donors who were switched from a moderate to an intensive plasmapheresis programme and observed over a 3-year period (Schulzki et al. 2006). Individuals donated up to 850 ml of plasma per session as many as 60 times per year. Severe clinical adverse events related to plasmapheresis; 4 four associated with venipuncture and a fracture associated with a fall, were observed in five subjects. Cardiovascular events were less frequent in the plasmapheresis group than in the corresponding general population. Other cross sectional and longitudinal studies of frequent plasma donors support the safety of the programmes (Rodell and Lee, 1999; Tran-Mi et al. 2004). In view of the relatively sparse scientific information about the long-term effects of the considerable extra load on protein synthesis and of a depleted total albumin pool, continued careful monitoring of donors is warranted.
During plasmapheresis, most of the platelets are returned to the donor. When PRP is harvested, the donor’s platelet count is well maintained until about 5 litres of plasma is collected per week; even then, platelet counts return to normal within 3 days of the last donation (Kliman et al. 1964). Concern has been raised regarding changes in bone metabolism that result from frequent exposure to citrate based anticoagulants that bind calcium, elevate parathormone levels and create metabolic alkalosis (Amrein et al. 2010). Studies at the National Institutes of Health indicate that whereas bone remodeling occurs, no evidence of osteopenia was observed when longtime apheresis donors were compared with a matched cohort of whole blood donors.
Care of Plasmapheresis Donors
The criteria for the acceptability of plasmapheresis donors are slightly stricter than those for routine blood donors. In the US, annual examination of frequent plasmapheresis donors is required (Solomon 1985). Laboratory tests are carried out periodically and include a full blood count, quarterly determination of total serum proteins, of serum albumin and of immunoglobulins as well as screening for urinary protein and glucose.
No donor should be subjected to plasmapheresis without detailed informed consent about the possible hazards (WHO 1989b). If donors are to undergo immunization with vaccines, red cells or blood group substances for the provision of specific immunoglobulins, guidelines to safeguard the donor should be followed (WHO 1975, 1989b).
If plasma donors give whole blood or do not have their red cells returned during apheresis, a period of 8 weeks should elapse before the next plasma donation, unless the physician in charge and the donor agree on the need for and safety of the procedure (WHO 1989a; AABB 2011).
Harvesting of Platelets, Leucocytes, Red Cells and Progenitor Cells
For the collection of platelets, leucocytes or peripheral blood stem cells (PBSCs), automated blood cell separators use microprocessor technology to draw and anticoagulate blood, separate components either by centrifugation or by filtration, collect the desired component and recombine the remaining components for return to the donor (Hester et al. 1979; Burgstaler 2010). The equipment contains disposable plastic software in the blood path and uses anticoagulants containing citrate or combinations of citrate and heparin that do not result in clinical anticoagulation of the patient or donor. Most instruments function well at blood flow rates of 30–80 ml/min and can operate from peripheral venous access or from a variety of multilumen central venous catheters. Centrifugal separation involves either a discontinuous or a continuous flow.
Intermittent (Discontinuous)-Flow Cell Separators
There are several varieties of instruments, all of which have in common a stationary tube, a rotating bowl and a rotating seal (Burgstaler 2010). These seals are now designed to maintain sterility, so that the system is considered ‘closed’ and the components can be stored for more than 24 h.
PRP can be collected in 30 min. From the PRP, 450 ml of plasma for fractionation and 150 ml of platelet concentrate, containing 2–3 × 1011 platelets, can be obtained depending on the donor’s platelet count. Platelet concentrates in a volume of approximately 300 ml and containing 3–6 × 1011 platelets require twice this time. In-line filters can effect acceptable levels of leucoreduction of the platelet concentrate (Seghatchian et al. 2001). An intermittent-flow cell separator has been designed with the flexibility to collect red cells, platelets or plasma (for clinical use or for fractionation), or any combination of these options depending on the programme chosen (Bandarenko et al. 2001). Further modifications of disposables and microprocessor software permit collection of double units of red cells or of red cells and plasma.
Continuous-Flow Cell Separators
Continuous-flow devices were developed initially for the collection of granulocytes (Freireich et al. 1965). Anticoagulated blood is fed continuously into a rapidly rotating chamber or channel in which red cells, leucocytes, platelets and plasma separate into layers. Any layer or layers can be removed through special ports, depending on the harness and programme, and the remainder, together with a replacement fluid, if plasma or red cells are being removed, returned to the patient.
The Amicus (Fenwal Inc. Lake Zurich IL) has a top panel onto which is placed a disposable tray which aligns a disposable harness set with the 6 pumps, 10 valves and 4 sensors that direct blood using a sealless rotating module into two chambers designed like a belt wrapped around a spool; citrated blood enters into the first chamber, where red cells are separated from PRP. The PRP is pumped into the second chamber, where the platelets or white cells are removed from plasma depending on the computerized programme and the collection chamber selected. The red cells are returned to the donor and most of the processing is carried out through computer-assisted devices. The donor’s extracorporeal volume is much lower, about 300 ml, than with intermittent-flow machines. The absence of a seal in the Amicus permits collection of platelets in a closed system, making it safe to store them for more than 24 h. High platelet yields are obtained with the standard platelet separation chamber which permits separation into 2 and even 3 therapeutic doses (Burgstaler 2010). The instrument is fully automated and has a relatively short processing time that yields a predictable, leucoreduced platelet concentrate. This instrument has single- and dual-needle capability, although the dual-needle procedure is used almost exclusively. A modification of this technology, the ALYX, is capable of collecting red cells or red cells and plasma.
The Spectra models (TerumoBCT, Lakewood, CO, USA) separate the different components of anticoagulated blood on the basis of density; they have a hollow, loop-like, rotating channel in which the blood travels circumferentially and in which centrifugal forces act radially. The centrifugal force and flow rate can be varied by the operator to adjust the composition of the buffy coat, which is then extracted via a tubing manifold into a collection chamber. The outlet port of each component is controlled by a separate pump. There are two types of channel designs, with different positionings and sizes of the exit ports, which are used to collect white cells or perform plasma and white cell exchange by one channel, or plasma exchange alone by another. A third type is configured for red cell exchange. A two-stage channel is available to collect a purer preparation of platelets; in the first stage PRP is separated from the red cells, and in the second stage the PRP enters a spiral and narrow channel in which the concentrated platelets and PPP are separated (Figure 17.3). The Spectra cell separator can use any of the three channel designs; the platelets collected with the dual-stage separation channel have little white cell contamination (less than 0.5 × 107 per concentrate) with a yield, after 90-min collection, often above 6 × 1011, sufficient to enable partitioning of the concentrate into two full adult therapeutic doses. The use of a sterile disposable harness and micropore filters for the anticoagulant and saline makes extended platelet storage safe. A leucoreduction enhancement to the software and harness (LRS in Figure 17.3) has reduced white cell contamination to less than 1 × 106 in more than 99% of uneventful platelet procedures. The instrument has a small extracorporeal volume and, depending on the programme chosen, is adaptable to different processes, such as platelet collection, plasma exchange, PBSC collection, etc. A programme is available which is only slightly slower than when two arms are used. Continuous-flow cell separators such as the Spectra tend to use more citrate per procedure giving rise to a greater frequency of hypocalcaemic symptoms.
Figure 17.3 Diagram of the COBE® Spectra Apheresis System LRS Continuous flow separation channel: whole blood entering the channel is separated in the first stage into PRP flowing clockwise and red cells flowing anticlockwise, leaving the channel through the ‘RBC out’ line. Transfer of all fluids to and from the pumps and the donor is through a closed loop system. The PRP enters the second stage by passing over a dam and is separated into a platelet concentrate and plasma, which is further processed in the LeukoReduction System (LRS) chamber into a leucocyte-poor platelet concentrate. The plasma leaves the channel partly through the ‘plasma out’ line and partly through the control tube, where it sustains the interface at the desired level in the second stage. Advanced fluid dynamics and fluidized particle bed technology separate out virtually all of the few remaining passenger leucocytes from the platelet concentrate, resulting in a final product with very few leucocytes (diagram supplied by Terumo BCT, Lakewood, CO, USA).
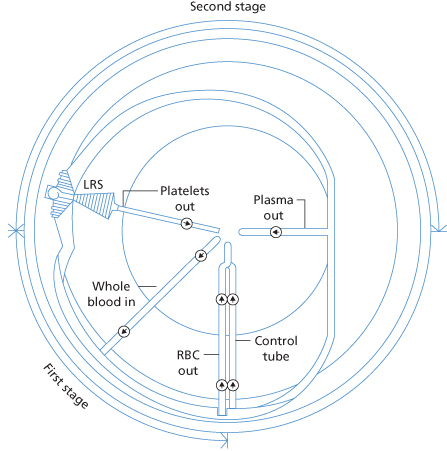
Several continuous-flow blood cell separators have been designed as on-site component processing systems capable of collecting red cells and other blood components on-line so that donor collections can be matched to blood inventory needs (Leitner et al. 2003). These instruments are intended for blood donation only. The intermittent-flow MCS plus has been described above. The TerumoTrima and Trima Accel are continuous-flow devices that use single needle access and either single-stage or dual-stage chambers similar to those of the Spectra (Rock et al. 2003). The Fenwal ALYX system is a single-needle, continuous-flow instrument that uses a reservoir system to achieve this match. All of these systems emphasize ease of use, programmed protocols, standardized component collection and flexible programming.
Continuous-flow cell separators have the advantages of speed and, because of the small extracorporeal volume involved, minimal blood volume fluctuations. The disadvantages of these separators are that, in general they are relatively immobile and require two sites of vascular access, although the Spectra is an exception in that it is lighter and more mobile and can be used with one vascular site of access. Detailed technical descriptions of centrifugal systems of cell separation have been compiled by Burgstaler (2010).
Collection of Platelets by Cell Separators
If platelet concentrates are to be stored, gas exchange through an appropriate plastic container is vital to avoid hypoxic conditions that lead to accelerated production of lactic acid and fall in pH. The availability of more permeable or thinner plastics has prolonged the shelf-life of platelet concentrates from 3 to 5 days, and containers suitable for 7-day storage have been produced (see Chapter 14).
Large quantities – at least 3 × 1011 platelets (a ‘full dose’) – can be collected from single donors using intermittent-flow centrifugation or by continuous-flow centrifugation, which not infrequently yields 6–10 × 1011 platelets (a double dose) per collection. The number, 3 × 1011, is an arbitrary one arrived at by consensus, rather than by some scientific or medical principle, at a time when instrumentation could not reliably collect greater numbers. Platelets appear to be mobilized from the splenic pool during the course of the procedure. The procedure normally requires 1–2 h and a relatively large volume of citrate anticoagulant is returned to the donor. Regardless of the system used, the platelet yield will depend mainly on the donor’s platelet count and blood volume before thrombapheresis and on the volume of blood processed. On the other hand, the volume of the platelet concentrate and the degree of white cell contamination will depend on the instrument used. Several studies have shown that platelets collected by apheresis have in vitro and in vivo functions similar or superior to platelets obtained from units of whole blood, although different collection protocols, containers, preservatives and processing complicate comparisons (Seghatchian and Krailadsiri 2000). Platelets for extended storage should be kept in the appropriate large plastic containers at a concentration suitable to maintain pH between 6.4 and 7.4. In the case of high platelet yields, with double or triple adult doses, i.e. >6 × 1011 platelets, the concentrate may be split into multiple storage containers.
The percentage of platelets collected by apheresis in different countries varies and is influenced by historical practice, cost, regulatory hurdles and perhaps by litigation as clinicians strive to limit the number of exposures to allogeneic donors. Some North American centres supply 100% of the platelets as apheresis donations. In the USA approximately 80% of platelet units derive from apheresis collections and more than 40% of collections are split into multiple doses, thus making more efficient use of the platelet donor (Report of the US Department of Health and Human Services 2011). When platelets from only one or two donors are needed, as when transfusing infants or using HLA-matched platelets collected by apheresis, donors should avoid aspirin-containing medication within the previous week, as aspirin impairs platelet function. On the other hand, when transfusing pooled concentrates obtained from many different donors, it is unnecessary to reject platelets from donors taking aspirin, as it is unlikely that all donors contributing to a pool will be taking the drug (Slichter 1985).
Yields of platelets with different modern cell separators are similar. Most continuous-flow systems meet the criteria of the Council of Europe for leucodepletion, 75% of products tested have white cell counts below 5 × 106 per 3 × 1011 platelets (equivalent to 1 × 106 per 0.6 × 1011 platelets). However, to achieve a white cell count below 1 × 106 per adult dose of platelets consistently, the platelets in the concentrate need to be counted and, if needed, platelet leucodepletion filters designed for this purpose can be used. Filtration results in a loss of about 15% of the platelets collected.
Donor Reactions
Thrombapheresis is generally safe and well tolerated (see also Chapter 15). However, rapid infusion of citrate anticoagulant solutions induces a fall in total and ionized calcium (30% decrease at 90 min) and a sharp rise in parathormone levels, both of which are blunted by oral calcium taken 30 min before the procedure (Bolan et al. 2001). A rise in osteocalcin levels as well as a striking increase in urinary calcium and magnesium excretion occur and persist for at least 24 h. Repeated procedures over years may result in long-term loss of total body calcium, however studies at NIH (S. Leitman, personal communication) suggest that bone remodeling occurs and bone density may actually increase. Clinical impressions notwithstanding, the common practice of administering oral calcium prior to the procedure, at least in a commercially available tablet preparation, has at best a minimal affect on relieving citrate-related symptoms in thrombapheresis donors (Bolan et al. 2003c) (see below). Reducing flow rate is more effective.
Transient but significant decreases in platelet counts have been documented to occur in donors undergoing single and serial short-term plateletpheresis collections (Glowitz and Slichter 1980; Rock et al. 1992). Regular plateletpheresis donors develop sustained decreases in platelet count of up to 50 000 per μl, which correlate directly with donation frequency in all but the most frequent donors. However, clinically significant thrombocytopenia is unusual (Lazarus et al. 2001).
Vasovagal reactions are common at the first donation, but appear to occur less frequently in apheresis donors than in whole blood donors (McLeod et al. 1998). Venepuncture pain, haematoma, nausea and vomiting occur in only about 1% of donors, and no life-threatening adverse events were encountered in a survey of 19 611 procedures at seven centres (McLeod et al. 1998).
Collection of Granulocytes by Leucapheresis
A unit of fresh blood contains 1.5–2 × 109 leucocytes. As only one-half can be retrieved by centrifugation, at least 10–12 and preferably double this number of units of blood would be required to provide a sufficient daily dose for an adult (2 units for a newborn infant) (see Chapter 14). The provision of leucocyte concentrates from routine donations entails exposing the recipients to large numbers of donors as well as sacrificing the red cells from those units because of the additives and manipulation needed in the processing. Granulocytes for adult transfusions are therefore not prepared routinely from whole blood units, but rather collected by leucapheresis from a single donor.
Three methods have been widely used, separately or more often in combination, to increase the yield of granulocytes: the administration of steroids to the donor to increase the peripheral granulocyte count, the introduction into the cell separator of a red cell sedimenting agent such as hydroxyethyl starch (HES) and stimulation of the donor with a haematopoietic cytokine such as granulocyte colony-stimulating factor (filgrastim, G-CSF). Stimulating donors with G-CSF or G-CSF with dexamethasone increases the yield of granulocytes compared to the yield from the traditional collection regimen of dexamethasone alone (Dale et al. 1998; Liles et al. 2000; Stroncek et al. 2001). The need for agents that cause rouleaux formation and thus help to separate red cells from granulocytes arises from the fact that the specific gravity of red cells and granulocytes is very similar (1.093–1.096 for red cells; 1.087–1.092 for granulocytes), so that centrifugation alone is a very inefficient means of separation (Mishler 1975).
Use of Donor Bone Marrow Stimulation
The yield of granulocytes correlates directly with the donor’s circulating granulocyte count. Granulocyte collection from normal volunteer donors by continuous-flow centrifugal separation yields insufficient numbers of cells unless the donor can be stimulated or ‘mobilized’ prior to the collection. The original stimulant was etiocholanalone, which releases marginating granulocytes from the peripheral pool (McCredie et al. 1974). The effect was transient and donor side-effects unacceptable.
The administration of corticosteroids increases the granulocyte count by stimulating the release of neutrophils from bone marrow reserves and by inhibiting the escape of granulocytes from the circulation. A single dose of oral steroids may be given at least 2 h and preferably >4 h before collection. For practical purposes, dexamethasone (6–12 mg) or prednisone (40–60 mg) is routinely administered the evening (8–12 h) before donation. For optimal results, 2–3 oral doses of dexamethasone can be given 12–18 h before leucapheresis. The pretreatment of donors within a 10- to 12-h period prior to leucapheresis with a double dose of dexamethasone significantly increased the total quantity and efficiency of granulocyte collected compared with a donor group receiving a single dose of dexamethasone (Winton and Vogler 1978). A mean of 25.5 × 109 total granulocytes were collected in addition to an efficiency of 2.11 × 109 granulocytes harvested per litre of blood processed in the double dose-treated donors, in contrast with 19.6 × 109 total granulocytes collected and an efficiency of 1.82 × 109 granulocytes harvested per litre of blood processed in the single-dose donor group (Higby et al. 1975).
Donor mobilization with G-CSF results in a 10-fold increase in the circulating granulocyte count and a sixfold increase in the number of cells collected compared with historical collections (Bensinger et al. 1993). A single subcutaneous dose of G-CSF administered 12–16 h before collection resulted in a five-fold increase in granulocyte yields and normal function of the collected cells (Caspar et al. 1993). Dexamethasone administration potentiates this effect and results in no immediate increased donor toxicity (Liles et al. 2000; Stroncek et al. 2001). Granulocyte mobilization response to subcutaneous G-CSF plus dexamethasone is sustained at peak levels for 8–24 h after coadministration of the two drugs, and even although the cytokine can be administered intravenously, no advantage accrues from doing so (Stroncek et al. 2002). Apheresis donors with higher baseline platelet counts and absolute neutrophil counts (ANCs) have higher ANCs after G-CSF/dex stimulation; donor age, weight, and sex do not have a significant impact (Quillen et al. 2009a). A retrospective study of 83 granulocyte donors who contributed to 1120 granulocyte concentrates after G-CSF stimulation, or a mean of 13.5 granulocytapheresis procedures per donor, did not differ in measures of health status from a control group of platelet donors with a mean of 87.5 plateletpheresis procedures per donor (Quillen et al. 2009b).
Use of Erythrocyte Sedimenting Agents
The yield of granulocytes can be increased by adding an erythrocyte sedimenting (rouleaux-forming) agent with the citrate anticoagulant to the input line of the cell separator. HES has been used extensively in the USA. The increased granulocyte yield with HES administration may not be due solely to its sedimenting properties, but may have a specific effect independent of an increase in erythrocyte sedimentation rate (ESR) (Mishler 1982). Both ESR and granulocyte collection efficiency (GCE) vary widely among donors, yet may remain relatively constant for a given donor. Baseline ESR correlates with hydroxyethyl starch-modified ESR and with GCE (Lee and Klein 1995). The HES commonly used is pentastarch, which has a lower molecular weight and is more rapidly excreted than hetastarch; it is used as a 10% solution in a 500-ml dose (Strauss et al. 1986). However, HES results in superior granulocyte collection and almost double the efficiency and has become the standard agent despite the longer in vivo retention time (Lee et al. 1995).
Plasmagel (a modified fluid gelatin) has been used widely in France as a red cell sedimenting agent. Plasmagel is eliminated more rapidly from the body than is HES, although allergic reactions are more frequent (Huestis et al. 1985), and plasma volume is also expanded (Rock et al. 1984).
Donor Complications
Donors who receive a single 5-µg/kg dose of G-CSF experience headache, bone pain, myalgia, arthralgia, insomnia, dizziness, nausea and fatigue (Dale et al. 1998; Liles et al. 2000). When donors were given dexamethasone, 58% reported one or more symptoms on at least 1 day, compared with 85% when they were given G-CSF alone and 75% when they were given G-CSF plus dexamethasone (Stroncek et al. 2001). The largest proportion of donors reported symptoms on day 2. When donors were given G-CSF, they were more likely to report two or more symptoms on day 2 than when they were given dexamethasone. Some donors continued to experience symptoms 3 and 4 days later. Donors given G-CSF or G-CSF plus dexamethas-one are more likely to experience arthralgias than when they are given dexamethasone alone, and donors given G-CSF are more likely to report headaches than when they receive dexamethasone alone (Stroncek et al. 2001).
Because corticosteroids are known to induce posterior subcapsular cataracts, concern has been expressed that repeated leucapheresis might increase the risk of cataract formation. Case control studies support this concern (Ghodsi and Strauss 2001; Burch et al. 2005, Clayton et al. 2011). Donors who have undergone repeated collections of granulocytes may benefit from an annual ocular examination and indication of cataract formation should preclude further donation. No other long-term risks of mobilization regimens have been reported, but few studies have been performed.
Solutions of hetastarch used for volume expansion produce significant abnormalities in some laboratory measurements of haemostasis (Strauss et al. 2002). It is unlikely that the modest haemostatic abnormalities produced at these doses would contribute to a risk of bleeding in volunteer granulocyte donors, even when infused on consecutive days. Hetastarch causes more haemostatic abnormalities than pentastarch.
Collection of Red Cells by Apheresis
An estimated 1.96 million red cell units were collected by apheresis in the USA in 2008, an increase of 11% compared with collections in 2006 (Report of the US Department of Health and Human Services 2011). The growth in the number of instruments capable of performing red cell collection and increasing acceptance by collectors and donors indicates that the trend is likely to continue worldwide. The several advantages include fewer donor visits per year, decreased testing costs and decreased donor exposures when both units are given to one recipient (Shi and Ness 1999). The procedure is particularly applicable to donors with haemochromatosis, as depletion of iron stores develops rapidly with repeated procedures (Leitman et al. 2003b). Other donors may benefit from iron supplementation. At present donor suitability is limited to larger (by weight) donors and the donation interval is extended to 112 days. The incidence of immediate adverse effects related to 2-unit RBC apheresis is about 3%, similar to that of other apheresis procedures. The majority of reactions involve discomfort from venepuncture, citrate intolerance and vasovagal reactions. Donor tolerance of 2-unit red cell donations (400 ml), a volume of red cells twice that in a standard 450-ml blood donation, does not seem to differ substantially from donor tolerance of standard or sham donations (Smith et al. 1996).
Collection of Progenitor (Stem) Cells from Peripheral Blood
Collection of Peripheral Blood Haematopoietic Stem (Progenitor) Cells
Optimal outcomes in hematopoietic stem cell transplantation (HSCT) depend on the successful procurement of progenitor cells from patients (autografts) or donors (allografts). Small numbers of haematopoietic progenitors circulate and large numbers can be mobilized and collected with apheresis technology. True pluripotent progenitor (stem) cells cannot be identified by microscopy, cell surface markers or cell culture techniques. However, good correlation exists between the dose of mononuclear cells expressing the CD34 surface marker, the presence of long-term culture initiating cells (LT-CIC) and haematopoietic engraftment in animal models and in human haematopoietic reconstitution. Most centres rely on CD34+ cells because they are readily quantified and the kinetics of CD34+ cell mobilization has been well studied.
Historically, mobilization of stem cells into peripheral blood for autografting was done using myelosuppressive chemotherapy such as cyclophosphamide following the observation that increased numbers of progenitor cells enter the circulation during the recovery phase of the bone marrow. In the late 1980s, the recombinant cytokines G-CSF and GM-CSF became available and were used either alone or in combination with chemotherapy as mobilizing agents. G-CSF is now the standard for this indication. The cells seem to be qualitatively similar to those found in bone marrow (Korbling et al. 1995; Prosper et al. 1997). Most available centrifugal instruments can achieve adequate doses for transplantation, although the efficiency of each collection varies with donor characteristics, operator experience and the particular instrument and protocol used; 10–20 litres are routinely processed per procedure. Collections of PBSC contain large numbers of platelets, leucocytes and red cells. The mean number of T lymphocytes, CD3+ cells, is about 10 times that of a bone marrow collection. Red cell volume ranges up to 22 ml, but ordinarily does not exceed 1.5 ml/l (Leitman and Read 1996). Intravenous electrolyte (primarily calcium) supplementation has permitted longer, more efficient collection procedures (Bolan et al. 2003b, 2004).
PBSC mobilization requires multiple daily injections of cytokine and the increase in circulating cells is temporary (Stroncek et al. 1996a). After the first 48 h of daily injections, no increase in circulating CD34+ cells can be detected. By the third day, cell counts begin to increase and reach a peak on the fifth or sixth day. The peak number varies among individuals (Stroncek et al. 1997). When 5- to 10-µg/kg per day G-CSF is administered, circulating CD3+ cells on day 6 range from 8 × 106 cells/l to 155 × 106 cells/l (Stroncek et al. 1997). After the sixth day, CD34+ cell counts fall even if injections are continued, achieve only one-third of peak by day 10 and one-quarter by day 11. Doses of up to 16 μg/kg per day have been administered. Some centres have found that doses injected twice a day appear more effective than a single dose (Waller et al. 1996), whereas other centres have not confirmed this split dose effect (Anderlini et al. 2000). Cytokine mobilization also increases the colony-forming units in peripheral blood, and LTC-IC in particular appear to mobilize well (Prosper et al. 1996). No increase in these progenitors or in CD34+ cells is found in the marrow, suggesting that mobilization does not result from expansion of cells in the marrow cavity, but from release from the marrow or expansion from peripheral blood. The marrow itself may be depleted at least transiently of progenitors responsible for long-term engraftment, as late graft failures have been reported when patients receive marrow grafts from donors stimulated with G-CSF (Mavroudis et al. 1998). The cytokine GM-CSF has also been studied as a mobilizing agent but does not appear to have any advantage over G-CSF and may result in more severe side-effects (Fritsch et al. 1994; Lane et al. 1995).
A significant proportion of patients do not mobilize with G-CSF with or without chemotherapeutic agents. Factors that have been shown to predict for poor mobilization include increasing numbers of cycles of prior chemotherapy, prior radiation therapy and the presence of marrow metastasis (Koumakis et al. 1996; Noach et al. 2003). Fludarabine is particularly toxic to stem cells and this should be avoided in patients for whom a stem cell collection for autograft is planned. Until recently poorly mobilizing patients had few options. However, an agent originally developed to treat HIV, Plerixafor (AMD3100), has been shown to have significant activity in these patients. In contrast to G-CSF which needs multiple injections over several days a single dose of Plerixafor mobilizes stem cells into the periphery beginning at 1 hour and peaking at 10 hours (Devine et al. 2004). In addition the quality of the stem cell graft mobilized by Plerixafor may be superior. Compared to G-CSF mobilized cells, Plerixafor mobilized cells appear more primitive and therefore more quiescent and produce superior engraftment in both NOD/SCID mice and human recipients. The combination of G-CSF and Plerixifor produces greater increases in CD34+ cells than does either agent alone (Flomenberg et al. 2005; Liles 2005). Donor demographic and laboratory predictors such as platelet count and CD34+ mononuclear cell count can be used to customize the length of the collection procedure (Vasu et al. 2008).
The mechanism(s) involved in the process of mobilization are incompletely understood (Kessinger and Sharp 2003). The G-CSF effect appears to be mediated by cytokine release and enzymatic action on CD34+ cell ligands. Dividing the daily dose may increase yields, at least in healthy volunteers (Kroger et al. 2004). Plerixifor (160 µg/kg × 1 on day 5) is a selective antagonist of the CXCR4 molecule that also appears to attach progenitors to their marrow niche. Plerixifor-mobilized leucapheresis products contained significantly greater numbers of T and B cells compared with G-CSF-stimulated leucapheresis products (Liles et al. 2005).
Whereas G-CSF is still the standard agent used, complications seen with stem cell mobilization are often related to this cytokine. Bone pain, headache, fatigue, insomnia, and gastrointestinal disturbances occur in 40–80% of donors, are usually mild and respond to administration of paracetamol or ibuprofen. Cutaneous reactions including folliculitis, urticaria, pyoderma gangrenosum and exacerbations of psoriasis have been reported (Brumit et al. 2003). Transient laboratory abnormalities include increases in sodium, lactate dehydrogenase (LDH), alkaline phosphatase, serum glutamate pyruvate transaminase and uric acid levels and decreases in potassium, bilirubin, blood urea nitrogen and magnesium levels. Platelet counts drop during the administration of G-CSF and following collection of PBPC. WBC counts rise during the administration of G-CSF, but then fall below baseline levels 2–3 weeks after the collection (Stroncek et al. 1996b, 1997). Spleen size increases in almost all individuals on G-CSF and this has been associated with splenic rupture (Falzetti et al. 1999). Donors should be advised to refrain from contact sports for a few weeks after the last mobilization (Stroncek et al. 2003). Vascular complications and citrate toxicity are not unlike those experienced in other long apheresis procedures.
Adequate cell dose for engraftment depends on whether the procedure is an autograft, a related, or an unrelated allograft. Cell dose, cell source, and patient characteristics are all important variables. The dose of stem cells for unrelated donors (Haynes et al. 1995; National Marrow Donor Program) is 2 to 4 × 108 nucleated cells per kilogram of recipient weight, with 2 to 4 × 106 CD34+ cells per kilogram of recipient weight deemed as an adequate dose for transplantation and doses of greater than 5 × 106 CD34+ cells per kilogram of recipient weight associated with more rapid engraftment. Lower doses may be adequate in related donor settings, but engraftment of both leukocytes and platelets correlates with CD34+ cell content.
Effects of Repeated Cytapheresis
Volunteer subjects undergoing repeated apheresis should be monitored to prevent levels of red cells, platelets and lymphocytes from falling below normal limits. Neutrophils are so rapidly produced and released that their number in the circulation remains unchanged.
Platelet Loss: Inadequate Components But No Donor Purpura
When platelets or granulocytes are collected with blood cell separators, the donor’s platelet count may not return to pre-donation levels for 6–7 days. Low platelet count limits the frequency of platelet donations by relatives of patients requiring HLA-compatible platelets. On occasion the platelet count can fall below 100 × 109/l and, although this poses no risk to the donor, thrombocytopenia to this degree makes further platelet collection inefficient (Strauss et al. 1983). Platelet donation is ordinarily deferred if the pre-apheresis platelet count falls below 150 × 109/l. Regular plateletpheresis donors develop sustained decreases in platelet count that correlate directly with donation frequency (see above) (Lazarus et al. 2001).
Lymphocyte Losses: Does Immune Compromise Result?
Large losses of lymphocytes may occur during intensive thrombapheresis owing to the similar density of lymphocytes and platelets. Broadly similar losses of lymphocytes were found in three different studies: 3.5 × 109 in donors from whom platelets or granulocytes were harvested by intermittent-flow cytapheresis, using a six-cycle procedure (Koepke et al. 1981); 6 × 109 per plateletpheresis donation (Fratantoni and French 1980) and 3 × 109 (T lymphocytes) in donors given prednisone and a sedimenting agent (Dwyer et al. 1981). The long-term effects of this loss of lymphocytes might seem potentially serious, however no adverse health problems have been reported. After 10 weekly donations, a fall in the lymphocyte count of 20%, with a decrease in B cells in one-half of the donors, has been observed (Koepke et al. 1981).
In a study of 21 healthy blood donors subjected to 9–17 cytaphereses over a period of 12 months, absolute lymphocyte count decreased 23%, T cells decreased 25% and B cells fell by 46%, with a reduction of 14% in serum IgG concentration (Senhauser et al. 1982). Similar results were obtained in 21 out of 113 donors who gave platelets regularly by apheresis, with a mean number of 13.1 procedures a year over a mean period of 162.7 weeks (Robbins et al. 1985). The lymphocyte count was inversely related to the total number of thrombaphereses and the CD4+ and CD8+ lymphocyte subsets were reduced in all 13 lymphocytopenic donors tested. However, CD4/CD8 ratios were found to be normal and no adverse effects were noted in any of the donors. Donors who develop lymphocytopenia (lymphocyte count < 1 × 109/l) are ordinarily excluded from donation until the lymphocyte count is > 1.5 × 109/l. Donors who fail to revert to normal within 8 weeks are often referred for further investigations (Strauss et al. 1983). On the other hand, short-term removal of lymphocytes has little measurable effect. Following a total of six lymphapheresis procedures over a 12-day period in 12 blood donors with a mean removal of 41.6 × 109 lymphocytes, no significant changes in absolute lymphocyte counts, immunoglobulin levels or in vitro tests of the immune response were measured (Blanchette et al. 1985).
Depletion of Haematopoietic Progenitors
Few donors have been mobilized repeatedly for progenitor cell collection. As yet there is no evidence that depletion of the stem cell compartment poses a risk to donors.
Symptoms and Signs in Donors Giving Blood Via Cell Separators
Apheresis donation is a safe undertaking, suitable for voluntary blood donors, with a very low risk of serious adverse effects. Many of the symptoms and signs such as vasovagal reactions are similar to those experienced by donors giving a routine blood donation or by patients during plasma exchange. Factors that may cause symptoms or signs include changes in the donor’s blood volume, chilling of returned blood and the administration of pharmaceutical agents such as citrate or HES. Of a total 195 372 donor apheresis procedures, complications were reported in 9.6% of cases, including 5.2% donor-related problems (e.g. fainting, citrate toxicity), 4% machine and harness problems and 0.4% operator errors (Robinson 1990). When transient paraesthesia and mild vasovagal events are excluded, a survey of 17 USA centres reporting on 19 611 apheresis donations recorded 600 adverse events in 428 donations (2.18% of donations and 4.84% of the 2295 first donations). Only 203 donations (1.04%) sustained non-venepuncture adverse effects. No life-threatening adverse effects were reported. Procedure-specific non-venepuncture rates were 1.05% of 17 584 platelet donations, 0.67% of 594 white cell donations and 0.37% of 1354 plasma donations. Centre-specific rates varied from 0.32% to 6.81% of donations for total adverse effects and from 0.11% to 2.92% of donations for non-venepuncture events (McLeod et al. 1998). Most of the immediate complications can be dealt with by the operator and apheresis need not be halted. Reactions can usually be controlled by adjusting the return flow of blood or the rate of saline infusion into the receiving arm.
Syncope, seizures and vasovagal effects occur more frequently when using intermittent-flow devices as the extracorporeal blood volume is larger than with continuous-flow blood cell separators especially during the last cycles. Extracorporeal volume should not exceed 15% of the donor’s own volume at any time (Huestis 1989). Severe vasovagal episodes were reported in 32 donors undergoing leucapheresis; 10 donors lost consciousness and had seizures (Sandler and Nusbacher 1982). However, if experienced blood donors are used for plasmapheresis and thrombapheresis, syncope occurs in only 0.2% of procedures although fainting and nausea are 10 times more likely when cells rather than plasma components are collected. From the UK apheresis registry, the chance of severe syncope requiring resuscitation has been estimated at 1 in 100 000 procedures (Robinson 1990). In the USA, vasovagal nausea and/or vomiting occurred in 0.87% of first-time and 0.13% of repeat donors, whereas the experience for syncope and/or seizure was 0.39% and 0.04% respectively (McLeod et al. 1998).
Chills are relatively common and may be due partly to extracorporeal chilling of blood and partly to undetermined factors. In the USA, chills and/or rigors were noted in 0.31% of first-time and 0.01% of repeat donors (McLeod et al. 1998). In most cases, a blanket or a blood warmer will prevent the chills.
Vascular effects such as venous spasm, collapse, infiltration and haematoma formation are more frequent in apheresis than in routine donations because one or two needles have to be in place for 60–90 min and the needles are usually larger because of the pumped red cell return (Robinson 1990; Simon 1994). Pain or haematoma at a venepuncture site was the most common complaint in the USA survey complicating 1.15% of donations (McLeod et al. 1998).
Citrate toxicity is related to the concentration of citrate used, the rate of re-infusion, the duration of the procedure, the volumes processed and whether a continuous or discontinuous procedure is used. Citrate ‘effect’, mild tingling, is common as contrasted with citrate toxicity. The distinction is a matter of degree, but toxicity can be aborted by early recognition of donor or patient discomfort. Common manifestations include circumoral paraesthesia, vibratory sensations, nausea, vomiting and overt tetany that may be exacerbated by the alkalosis that follows hyperventilation.
Citrate-induced nausea and/or vomiting occur in less than 1% of donors and tetany is less frequent by an order of magnitude (McLeod et al. 1998). In the collection of plasma components, the return of citrated plasma is small and the incidence of toxicity is 10 times lower than when leucocytes or platelet concentrates are collected (Robinson 1990). Signs of citrate toxicity closely parallel the fall in ionized calcium when standard concentrations of ACD-A (Olson et al. 1977; Bolan et al. 2001) or sodium citrate (Mishler et al. 1977) are used (see above). Signs of toxicity may be avoided by slowing the rate of infusion or by using a lower concentration of citrate, combined if necessary with a small amount of heparin (Huestis 1986a). In the treatment of citrate toxicity during voluntary donation, the administration of intravenous calcium is not recommended routinely as it can cause clotting in the extracorporeal circuit or arrhythmia in the donor (Westphal 1984). However, in the USA, where the recommended citrate load (ACD-A) should not exceed 1 ml/l of the total blood volume, i.v. calcium has been administered to donors to prevent symptoms of citrate toxicity (Bolan et al. 2002). Oral tablets of calcium are not particularly effective in preventing either hypocalcaemia or donor symptoms (Bolan et al. 2003a,c). In the UK, there are recommendations for maximum flow rates for the different anticoagulant ratios in different instruments to avoid the need to use calcium supplementation (Robinson 1990).
Adverse effects of hydroxyethyl starch – febrile and allergic reactions due to HES are discussed in Chapter 2. Frequent infusions of HES over a short period are potentially hazardous. Four healthy donors were given infusions of 500 ml of HES (molecular weight 450 000) daily for 4 days. After the fourth infusion, the average increase in plasma volume was 0.85 litre; 24 h later, in all but one of the donors, plasma volume was still 0.5 litre above the donor’s initial value (Rock and Wise 1979). In subjects who donate granulocytes regularly by apheresis and receive repeated infusions of HES, plasma HES levels are still raised 3 months after the last infusion (Ring et al. 1980). In such donors, headache, mild hypertension, swollen fingers and peripheral or periorbital oedema may be present (Westphal 1984). Abnormalities of coagulation assays occur, but bleeding does not appear to be a concern (Strauss et al. 2002) (see above).
Transitory side-effects due to steroid administration such as headache, insomnia, flushing, palpitations and euphoria occur in some donors and are dose related.
Allergic reactions consisting of sneezing, urticaria or even angioedema are seen in occasional donors and are five times more likely to occur in donors giving cells than in donors giving plasma components (Robinson 1990). Reactions are more common in donors who are taking ACE inhibitor medication. Severe reactions due to sensitization to ethylene oxide are discussed in Chapter 15.
Mild haemolysis is seen occasionally and is caused by kinks in the plastic tubing (Huestis 1986a, 1989; McLeod et al. 1998).
Infections at the site of venesection are more likely to occur in cytapheresis donors who have two needles in their veins for a period of 1–3 h. There is also the more remote, although more serious, danger of infection from contaminated bottles of solutions used in cytapheresis or from leaking harnesses (Kosmin 1980).
Mortality
Fatalities attributed to the donation of plasma or cells by apheresis are rare. Nine deaths were reported to the FDA for the 10 years between 1976 and 1985; eight of these were attributed to plasma donations and one to leucapheresis (Sazama 1990). No deaths attributed to apheresis have occurred either in 289 385 procedures analysed in the UK registry from mid-1985 to April 1989 or in the USA survey.
Therapeutic Plasma Exchange
The exchange of large volumes of plasma and their replacement with other colloids such as albumin or allogeneic plasma became possible with the introduction of automated plasmapheresis performed with centrifugal blood cell separators or with hollow fibre or membrane filters. Filtration can occur at a high flow rate through continuous-flow systems, and with a pore diameter between 0.2 and 0.5 µm, immunoglobulins and most immune complexes can be removed while the cells pass through the separator and return to the patient with the replacement fluid. However, large immune complexes and IgM may not be removed effectively and cryoglobulins may gel in the extracorporeal circuit, impairing their clearance. Several variations of filtration using combinations of filters or filters and columns have been devised to remove plasma components of a specific molecular weight or a particular physical characteristic (‘cascade filtration, cryofiltration’), but with the exception of columns for removal of low-density lipoproteins (see below), these procedures are not widely used (Pineda 1991; Griffin 1996). Virtually all therapeutic exchanges are performed with centrifugal blood cell separators.
The main criteria for the application of therapeutic plasma exchange and more particularly for immunoadsorption are: (1) the existence of a known pathogenic substance in the plasma; and (2) the possibility of removing this substance more rapidly than it can be renewed in the body. However, clinical improvement does not necessarily follow the removal of a pathogenic substance. On the other hand, some conditions improve after plasma exchange even when the concentration of the substance in the plasma does not fall. Moreover, in uncommon conditions such as thrombotic thrombocytopenic purpura (TTP), there appears to be, in addition to unwanted substances that need to be removed, a missing plasma factor that is supplied in sufficient quantities by replacement with fresh-frozen plasma (FFP) or cryoprecipitate-poor plasma (Levy et al. 2005) (see below). In general, plasma exchange is used to treat severely ill patients not responding to conventional therapy. As plasma exchange has short-lived benefits and because it is a complex and expensive form of therapy which is not without risk, it should be used in only two circumstances: in well-controlled clinical trials, and in treating patients with one of the few conditions in which there is good evidence of its efficacy (Szczepiorkowski et al. 2010; Griffin et al. 1996).
Volumes of Fluid Exchanged
The volume of fluid exchanged at each therapeutic plasma exchange (TPE) is usually equivalent to the patient’s plasma volume. The usual practice consists of a course of four or five procedures carried out within 7–10 days, although the volume and frequency of plasma exchanges vary depending on the individual patient, the clinical condition and the protocols of each centre.
In TPE, the amount of a specific pathogenic substance removed depends on the volume of plasma exchanged and on the frequency of the procedure. The concentration Xt of a pathogenic substance X after a time t (in h) of plasma exchange is given by the equation:
where X0 represents the initial concentration of the pathogenic component and r the fractional rate of exchange or volume of plasma exchanged per hour divided by the plasma volume (Calabrese et al. 1980). The equation is valid only if the following conditions are met: complete mixing, no change in the patient’s blood volume and no influx of the component from the extravascular space. In these circumstances, each plasma exchange equivalent to one plasma volume will remove approximately 60% of a (pathogenic) intravascular component. The removal of 40 ml of plasma per kilogram of body weight gives roughly the same results as predicted by the equation (Huestis 1986c). In practice, the conditions outlined above are rarely met. For molecules that are mainly intravascular, such as immune complexes or IgM, the final concentration is nearer the calculated value than for smaller molecules such as IgG, which diffuse freely to the extravascular space. Other factors that need to be taken into consideration are the catabolism, half-life and rate of renewal of the substance being removed. If resynthesis is very rapid, greater numbers of procedures will be required. Rebound phenomena tend to occur after plasma exchange when antibodies or immune complexes need to be removed. In these cases the concurrent use of immunosuppressive therapy has proved the treatment of choice, as plasma exchange reduces the required dose of immunosuppression with a consequent reduction in hazards (Huestis 1986c).
Vascular Access
An antecubital vein is used whenever possible. For children, and when more effective blood flow is needed, a catheter can be passed into a femoral or subclavian vein, or arteriovenous shunts, arteriovenous fistulae or prosthetic grafts can be established. Temporary rigid polyethylene and Teflon catheters and semi-rigid polyurethane ones are used for emergency and short-term access. A semi-permanent catheter, made of soft silicone rubber with a subcutaneous anchoring system inserted through a subcutaneous tunnel is indicated for medium- and long-term use. Although catheter design has benefited greatly from technical advances and material haemocompatability, catheter-related morbidity still remains high and is associated with significant rates of infection and thrombosis that can extend proximally and distally. The morbidity includes gangrene, aneurysm formation, cardiac arrhythmia, pneumothorax, perforation of great vessels and even of the heart (Huestis 1986b; Couriel and Weinstein 1994; Stegmayr and Wikdahl 2003).
Replacement Fluid
In general, in TPE, replacement fluids are given in a volume equal to that of plasma removed, although patients with fluid overload may be given smaller volumes of replacement fluids and those with contracted plasma volumes may be given additional fluids to avoid hypotension. Colloid osmotic pressure and electrolyte balance are generally maintained. Except for TTP, for which plasma is the treatment of choice (see below), the composition of the replacement fluid is rarely important. If the patient’s protein levels are normal, 1 litre of crystalloids may be used at the beginning of the procedure; the replacement fluid given first will be partially removed at the end of the exchange (Urbaniak 1983). A large number of centres routinely use equal volumes of 0.9% saline and 4–5% albumin as a replacement fluid. Much has been made of the removal of coagulation factors, but clinically important bleeding does not often occur. Immediately after exchange, significant prolongation of the prothrombin, partial thromboplastin and thrombin times occurs, with reduction of the fibrinogen and antithrombin III levels. Within 4 h of exchange, almost all factors, including platelets, return to pre-exchange values, and at 24 h all are corrected (Flaum et al. 1979). Plasma exchange with solutions devoid of coagulation factors results in a coagulation defect that may be of clinical significance in a haemostatically compromised patient. Partial replacement with FFP may be needed for patients with a risk of bleeding such as those with liver disease or disseminated intravascular coagulation (DIC). Patients with hypoalbuminaemia or contracted plasma volume may require total replacement with 5% albumin. Patients undergoing multiple, intensive exchanges may become hypofibrinogenaemic; this is rarely of clinical significance. The plasma Ig levels may also fall considerably in such patients, but it is not clear that replacement IVIG is needed. The levels of certain complement components such as C3 may drop precipitously and, similarly, cholinesterase levels may become so low as to lead to apnoea in patients injected with suxamethonium prior to surgery (Evans et al. 1980).
The disadvantages of the use of plasma as replacement include: increased incidence of citrate toxicity and of urticarial and anaphylactic reactions, transmission of viral infections and passive transfer of antibodies such as leucoagglutinins, thought to be responsible for the severe and even fatal pulmonary complications (TRALI, see Chapter 15) of plasma exchange. If plasma needs to be used in the UK, it is obtained from male volunteers and subjected to viral inactivation. However, the ideal replacement fluid is the patient’s own plasma from which the pathogenic component had been removed. Unfortunately, methods for the selective removal of plasma constituents are not without risk and are, at present, applicable only to a small number of diseases (see below).
Indications for Plasma Exchange
Plasma exchange is indicated for conditions in which well-controlled trials have shown the treatment to be of value and in others in which the pathogenic plasma component can be removed or replaced. Although reports claim benefit for plasma exchange in more than 100 disorders, scientific studies and well-controlled clinical trials are sparse (Szczepiorkowski et al. 2010; Griffin et al. 1996; Shehata et al. 2002). There are only a few conditions in which plasma exchange has been shown to be of definite benefit: the hyperviscosity syndrome, cryoglobulinaemia, myasthenia gravis, Guillain–Barré syndrome, chronic inflammatory demyelinating polyneuropathy, homozygous familial hypercholesterolaemia, Goodpasture syndrome, Refsum disease, post-transfusion purpura and TTP. In many other conditions, suggestive evidence of the value of plasma exchange has been obtained, but the absence of control subjects makes it impossible to reach definite conclusions; plasma exchange in D-immunized pregnant women is one example. Reviews of the effects of plasma exchange in various other conditions abound (Szczepiorkowski et al. 2010; McLeod 2002; Shehata et al. 2002; Smith et al. 2003). Increasingly, IVIG has become the preferred initial form of therapy for autoantibody-mediated immune disorders such as PTP, refractory immune thrombocytopenia (ITP), acute Guillain–Barré syndrome, myasthenia gravis and systemic vasculitis in patients with Wegener granulomatosis (Jayne 1990; Kasprisin 1991; Knezevic-Maramica and Kruskall 2003).
Several professional societies have published evidence-based consensus guidelines for therapeutic plasmapheresis. The current evidence-based recommendations of The American Society for Apheresis (ASFA) have been published by category and further divided according to strength of evidence (Szczepiorkowski 2010). A modification of these recommendations can be found in Table 17.1. Category I lists disorders for which apheresis is accepted as first-line therapy, either as a primary standalone treatment or in conjunction with other modes of treatment. Category II lists disorders for which apheresis is accepted as second-line therapy. In category III, the role of apheresis therapy has not been established and therapeutic decisions are generally individualized. Category IV lists disorders in which published evidence suggests that apheresis is ineffective or even harmful.
Table 17.1 Recommendations for therapeutic plasma exchange.
Removal of Antibodies
Alloantibodies
Plasmapheresis to remove anti-HPA-1a in PTP is standard therapy (see Chapter 15). Removal of anti-D and anti-P during pregnancy reportedly salvages some fetuses and allows some women with recurrent spontaneous abortion to carry the pregnancy to term (Robinson and Tovey 1980; Rock et al. 1985) (see Chapter 12). Removal of major blood group and HLA antibodies has been undertaken before renal transplantation and for hyperacute rejection (Akalin et al. 2008). The removal of anti-A and anti-B, now seldom necessary in ABO-incompatible bone marrow transplants (see Chapter 14), has proved useful prior to ABO-incompatible renal grafts (Griffin et al. 1996; Gloor et al. 2003). The data on graft survival are impressive, although uncontrolled (Sonnenday et al. 2002; Montgomery et al. 2011). The role of humoral immunity in causing antibody-mediated rejection of organ allografts has been extensively documented. Thus, negative complement-dependent cytotoxicity (CDC) cross-matches between recipient sera and donor T and B lymphocytes have become a mandatory requirement for solid organ transplantation. 32% of patients on transplant waiting list are HLA alloimmunized (13% of these with >80% PRA). Treatments are designed to lower the antibody titer to an acceptable level that will allow successful transplantation and decrease risk of acute rejection. Long-term renal transplant outcomes vary according to different pre- and post-transplant regimens (Jordan and Pescovitz 2006). A similar rationale supports treatment with plasmapheresis for rejection of cardiac allografts.
Autoantibodies
For example, anti-acetylcholine receptor (AChR) in myasthenia gravis; anti-basement membrane in Goodpasture syndrome; factor VIII inhibitor in unresponsive haemophiliacs with severe haemorrhage and in the rare antibody-mediated, pure red cell aplasia; autoantibodies to epidermal cell membrane glycoproteins in pemphigus; platelet autoantibodies in refractory immune thrombocytopenia, although IVIG is now the treatment of choice; and peripheral nerve myelin autoantibodies in Guillain–Barré syndrome, where TPE has been shown to be of benefit in two large clinical trials (McKhann et al. 1988). IVIG appears to be equally effective (van der Meche and Schmitz 1992).
In myasthenia gravis, removal of AChR autoantibodies by plasma exchange is associated with clinical improvement, relieving weakness of respiration and swallowing after about 2 days (Dau et al. 1977; Richman and Agius 2003). However, there is little role for TPE in the long-term management of this condition. Patients with the Lambert–Eaton myasthenic syndrome, caused by autoantibodies to the calcium channel on the pre-synaptic nerve terminal, also benefit from TPE (Griffin et al. 1996; Newsom-Davis 2001). IVIG affords equal benefit in the acute phase and immunosuppressive therapy provides long-term control in both conditions.
In Goodpasture syndrome, a rapidly progressive glomerulonephritis accompanied by pulmonary haemorrhage, autoantibodies to type IV collagen in the glomerular and alveolar basement membranes can be removed by plasma exchange; the procedure is particularly effective in the early stages of the disease. Suppression of further antibody synthesis can be achieved with immunosuppressive medication such as cyclophosphamide, azathioprine and corticosteroids (Lockwood et al. 1975). Early treatment is essential, as renal impairment is irreversible (Levy et al. 2001). A small controlled trial confirmed that the addition of plasmapheresis not only results in the expected rapid decline in antibody to glomerular basement membrane (anti-GBM), but in improvement in renal function, renal histology and disease outcome (Johnson et al. 1985). Combination of TPE and immunosuppression proved similarly successful in a small controlled study of rapidly progressive glomerulonephritis (excluding Goodpasture syndrome), even when patients had become dependent on dialysis (Pusey et al. 1983). Experience with dialysis-independent patients in a large multicentre trial failed to confirm these results (Glockner et al. 1988). In severe systemic vasculitis (Wegener granulomatosis, Churg–Strauss syndrome) with pulmonary haemorrhage, even in the absence of renal involvement, plasma exchange combined with immunosuppression has a chequered history, but seems to provide reasonable adjunctive therapy in patients with rapidly progressing disease, especially when a patient’s organ or life is threatened (Guillevin et al. 1995; Gaskin and Pusey 2001). Plasma exchange removes anti-neutrophil cytoplasmic autoantibodies (ANCAs) and immune complexes as well as inflammatory mediators such as cytokines, complement factors and coagulation components, but improvements in clinical outcome have been difficult to document.
Removal of Immune Complexes and Macromolecules (E.g. Rapidly Progressive Glomerulonephritis (See Above), Systemic Lupus Erythematosus and Cryoglobulinaemia)
Symptomatic treatment of cryoglobulinaemia with plasma exchange has proved successful on a short-term basis. The cold-precipitating properties of cryoglobulins make it possible to deplete them selectively, although cold ultrafiltration has not gained many supporters (Pineda 1991; Siami and Siami 1998, 2004). Removal of the cryoprotein does not always improve the clinical syndrome. Plasma exchange has enjoyed some success in treating patients with recurrent focal segmental glomerulosclerosis (FSGS) after renal transplantation and less commonly in the native kidney. A circulating plasma factor that increases glomerular permeability to albumin has been isolated from these patients, but this may not be the pathogenic agent. TPE is often used, but appears to be less efficacious in adults than in children with this disease (Matalon et al. 2000). TPE is usually used in combination with steroids and immunosuppression and the effects are most likely transient (Moroni et al. 2010).
Plasma exchange effectively removes antibodies, immune complexes and inflammatory mediators from the blood of patients with systemic lupus erythematosis. Despite a wealth of anecedotal reports that point to efficacy, controlled trials have failed to define a treatment strategy that would benefit patients with lupus nephritis or other manifestations of the disease , with the possible exception of life-threatening cerebritis (Wei et al. 1983; Lewis et al. 1992; Doria et al. 1994; Wallace et al. 1998).
Removal of Monoclonal Proteins (Hyperviscosity Syndrome)
The hyperviscosity syndrome (HVS), most commonly due to an increase of serum IgM, was first described by Jan Waldenström in 1944 and occurs in 10–30% of patients with Waldenström macroglobulinaemia (Waldenström 1944). Much less often patients with multiple myeloma or rheumatoid arthritis produce high-molecular-weight polymers of IgG or IgA that result in (in patients with multiple myeloma). Patients may present with headache, vertigo, blurred vision as a result of hyperviscosity, or purpura as a result of interaction of the elevated immunoglobulins with circulating platelets. Progression of HVS can lead to confusion, stroke, or coma. Treatment is ordinarily instituted based on symptoms and fundoscopic findings of retinal venous engorgement, exudates, or papilledema. Plasma viscosity measurements are usually unavailable acutely and vary significantly among patients (MacKenzie and Babcock 1975).
One of the most effective applications of plasma exchange is the treatment of the acute cardiovascular, neurological and haemorrhagic complications of this disease before there has been time for chemotherapy to become effective (Solomon and Fahey 1963). Removal of a small volume of plasma may result in dramatic clinical improvement as the expanded plasma volume is returned toward normal. In most cases, only two or three plasma exchanges are needed; the replacement fluid should be plasma free and, if the volume replaced is moderate, protein free as well. There is no need to achieve a normal plasma viscosity. Patients who are refractory to, or intolerant of, chemotherapy can be maintained on plasma exchange at regular (4- to 6-weekly) intervals. Comprehensive reviews describing the rationale and treatment schedules for plasmapheresis of patients with a variety of paraproteinaemias, including cryoglobulinaemia and Waldenstrom’s macroglobulinaemia have been published (Drew 2002).
Removal of Excess Plasma Constituent (E.g. Phytanic Acid as an Adjunct to Restrictive Diet in Refsum Disease)
Simple plasma exchange may be used in patients with other inherited metabolic diseases, such as Refsum disease (Gibberd et al. 1979). The frequency of exchange depends primarily on total body burden, rate of synthesis and plasma concentration of the solute to be removed (Figure 17.4). Less evidence exists to support a role for repeated treatments in these diseases.
Figure 17.4 Plasma exchange to remove plasma neutral glycolipids in a patient with Fabry disease. The plasma lipid recovery kinetics appear biphasic, reflecting initial re-equilibration from tissue stores and subsequent new synthesis of that glycolipid.
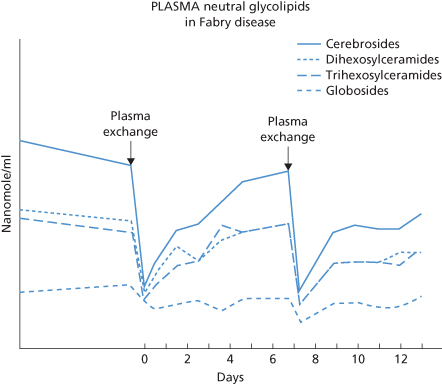
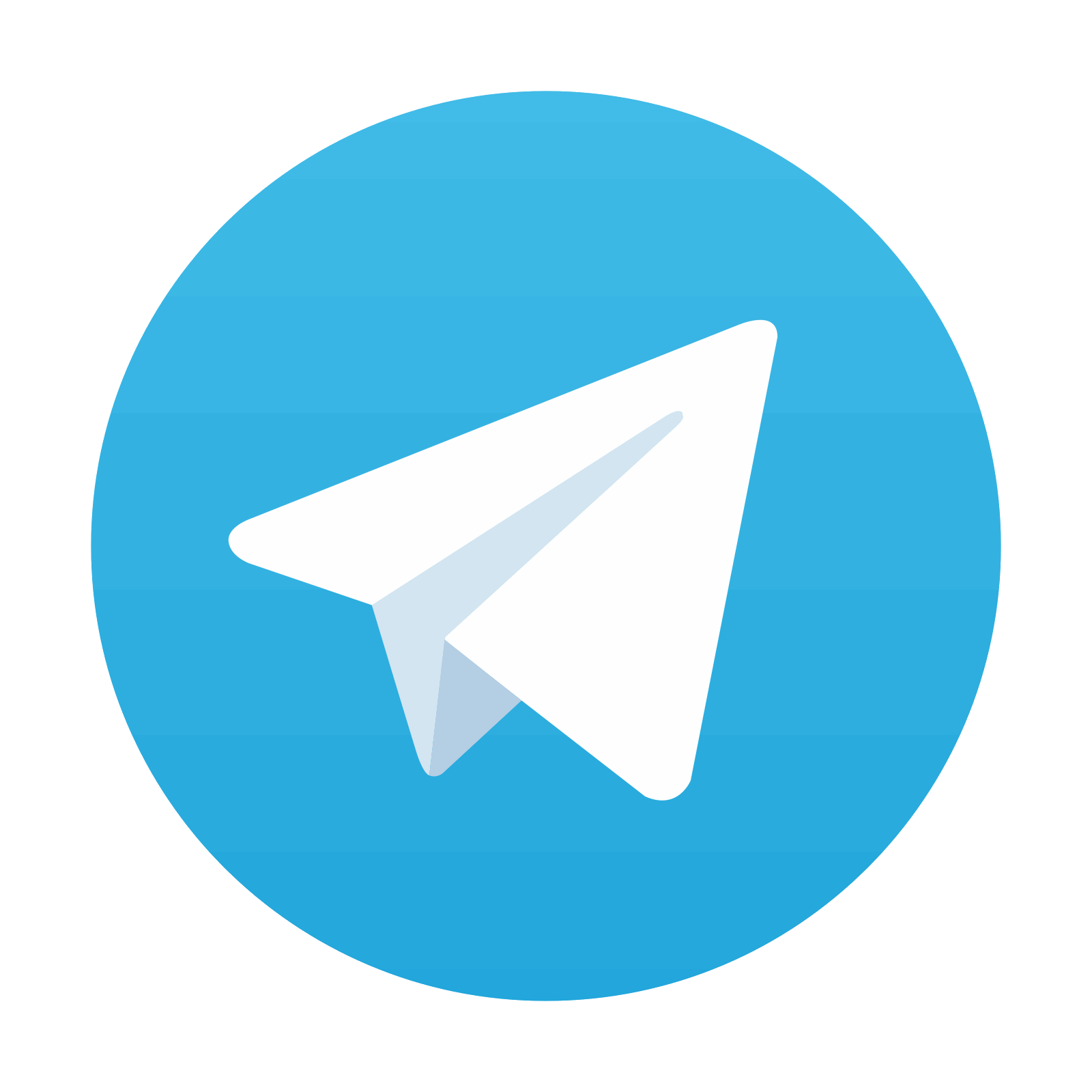
Stay updated, free articles. Join our Telegram channel

Full access? Get Clinical Tree
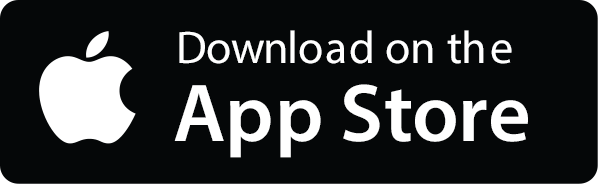
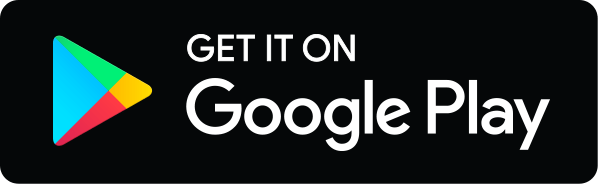