Fig. 4.1
Source of estrogens in lean premenopausal and obese postmenopausal women. In premenopausal women, the main source of estrogens is the ovaries. Estrogens then act in an endocrine fashion to regulate function of tissues including the brain (blue), bone (green) and uterus (black). After menopause, extragonadal tissues produce low levels of estrogens which then act in an autocrine and paracrine fashion. In obese postmenopausal women, the adipose becomes the predominant site of estrogen biosynthesis contributing to an increase in the risk of developing estrogen-dependent breast tumors
4.2 The Aromatase Enzyme
The aromatization of C19-androgens into C18-estrogens is the key and final step in estrogen biosynthesis and this reaction is uniquely catalyzed by the aromatase enzyme. Aromatase is a member of the cytochrome P450 superfamily of hemeproteins which contains over 6,000 members. Aromatase belongs to gene family CYP19 and is the family member CYP19A1. Most members of this superfamily have molecular weights in the range of 50 kDa, and in keeping with this, aromatase has 503 amino acids. The sequence has regions of marked homology with other members of the superfamily, notably a putative N-terminal membrane-spanning region, I-helix, Ozols and heme-binding region. These enzymes require molecular oxygen for their catalytic activity as well as a source of reducing equivalents. In the case of the mammalian members, these are derived from NADPH. The NADPH interacts with and passes electrons to the flavoprotein NADPH-cytochrome P450 reductase which in turn transfers the electrons to the aromatase cytochrome P450. These proteins are located at the endoplasmic reticulum [87]. The aromatase protein, expressed from a full-length cDNA insert, was shown to catalyse the aromatization of androstenedione and testosterone, and in the case of the human placenta, 16α-hydroxyandrostenedione. The reaction was also shown to be inhibited by known aromatase inhibitors. Conversion of C19-steroids into C18-estrogens occurs in a complex three-step process catalysed by this single polypeptide chain and requires three moles of cofactor NADPH and three moles of oxygen for every mole of C19 androgen converted (Fig. 4.2). Light has recently been shed on the precise molecular symphony that gives rise to the formation of estrogens as a consequence of the elucidation of the crystal structure of aromatase by the group of Ghosh and colleagues in Buffalo, NY [88]. The proposed reaction mechanism highlights the importance of residues such as D309 in the catalytic reaction mechanism as well as that of C437. This cysteine is present in the heme-binding region and occupies the 5th coordination position of the heme iron, and is uniquely common to all P450 enzymes. Specific to aromatase, in the third step of the reaction sequence, the A ring aromatization step, D309 is shown to be involved in enolization of the 3-oxo group, and removal of the 2β-hydrogen is facilitated by the carbonyl group of A306. The reaction mechanism of aromatase is very specific essentially limited to C19 steroids due to a highly specific binding cleft at the catalytic site.
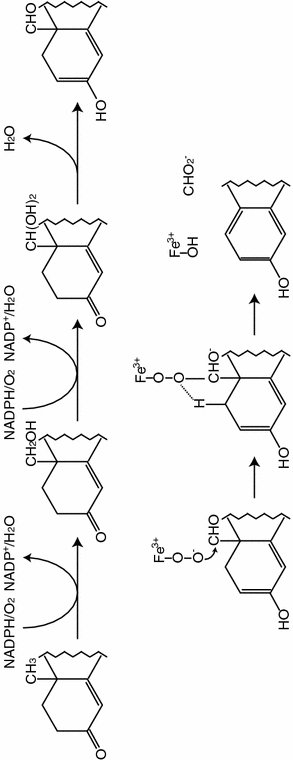
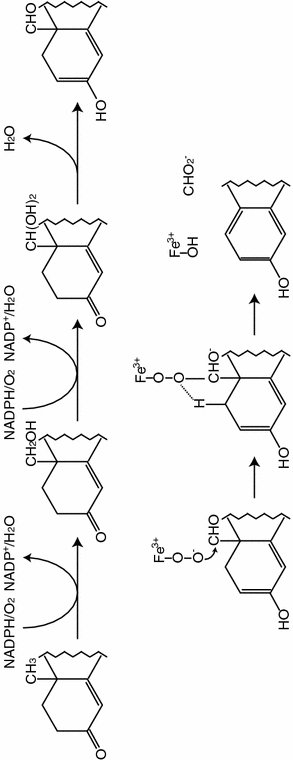
Fig. 4.2
Mechanism of aromatization of estrogens into androgens by the aromatase enzyme
4.3 Local Aromatase Expression in Breast Cancer
The source of estrogens driving tumor growth in postmenopausal women remains an area of contention. Two schools of thought exist. On the one hand, it is believed that estrogens produced locally within the breast serve to stimulate breast cancer cell growth whereas the second contends that circulating estrogens are taken up by the breast and drive tumor growth. Both arguments have merit and the strength of each argument depends on adipose-derived estrogens as a driver of tumor cell growth.
Evidence to support circulating estrogens as drivers of tumor growth are largely epidemiological and rely on findings demonstrating that postmenopausal breast cancer incidence is positively correlated with body fat content and serum estrogen levels [89, 90]. This argument is also supported by findings demonstrating that intratumoral estrogens are positively associated with tumor ERα expression, suggesting that uptake of estrogens contributes to tumor growth [91]. These two studies are not inconsistent, however, with circulating estrogens arising as a consequence of adipose tissue estrogen biosynthesis or originating from the adjacent breast adipose.
There is substantial evidence to support local breast estrogen production as a major driver of breast tumor growth. Of note, O’Neill et al. examined mastectomy tissue from breast cancer surgery and demonstrated that aromatase activity is highest in the breast quadrant which contains the tumor [92]. These findings were later supported by studies examining aromatase transcript, protein and activity in similar tissue [93–97]. This would suggest one of two scenarios, either that tumors tend to originate in areas which have high estrogen biosynthesis or that factors produced by tumors stimulate aromatase expression and tumor growth. Reports to date would suggest that both scenarios exist. As support for the hypothesis that breast tumors originate in a region of the breast with high estrogen levels, a recent study has demonstrated that mammographically dense breasts have high aromatase expression [38]. Using immunohistochemistry performed on breast core biopsies, the authors demonstrate that areas from dense areas of the breast had the highest immunoreactivity for aromatase with highest levels being seen in the stroma compared to other cell types and non-dense areas of the breast. The hypothesis that this elevated aromatase expression may lead to an increased risk of breast cancer is not only supported by epidemiological data demonstrating a clear link between mammographic density and breast cancer incidence, but also in animal models whereby overexpression of aromatase in the mammary gland of ovariectomized mice leads to breast hyperplasia, a phenomenon that is reversible in the presence of the aromatase inhibitor letrozole [98].
The relative contribution of estrogen biosynthesis within various cell types within the breast has also been explored. Adipose stromal cells express aromatase and have measurable aromatase activity [99], and much of the work pertaining to aromatase regulation within the breast has been performed using adipose stromal cells in monolayer culture. Nevertheless, aromatase immunoreactivity has been detected in both the adipose stroma and tumorous epithelium of the breast [100–102] and factors that contribute to the regulation of aromatase expression in both cell types is discussed below.
4.4 The CYP19A1 Gene and Tissue-Specific Expression
Unlike other steroidogenic enzymes, including 17β-hydroxysteroid dehydrogenases, which undergo tissue-specific regulation due to being encoded by different genes, aromatase is encoded by a single gene with a number of tissue-specific promoters. These promoters are under the control of a tissue-specific set of regulatory factors and direct the expression of a number of untranslated 1st exons which are spliced into the transcript at a common site upstream of the translational start-site such that the protein product is identical in each tissue site of expression (Fig. 4.3). Thus in the placenta, a unique promoter, I.1 is employed which is some 90 kb upstream of the translation start site. This promoter is regulated by factors such as hypoxia factor 1α (HIF1α) and estrogen-related receptor γ (ERRγ) [103]. In adipose tissue, another distal promoter is utilized, I.4, which is regulated by class 1 cytokines and TNFα in the presence of glucocorticoids. However, proximal to a breast tumor, a promoter adjacent to the translation start site is employed, namely promoter PII. This promoter is regulated by cAMP and in the case of adipose tissue, its expression is driven by PGE2. In the ovary, promoter PII is also involved and in this case expression is driven by FSH. The complex nature of the aromatase gene therefore allows the biosynthesis of estrogens at extra-gonadal sites to be finely tuned and responsive to changes in a woman’s physiology. Since the initial discovery of human aromatase transcripts in 1986 [104], much work has been done to characterize the structure of the gene that encodes it, CYP19A1, and its regulatory regions. In 1988, the full length cDNA from human placental libraries was isolated [105, 106] and the CYP19A1 gene was mapped to band 15q21.1 of the human genome [107]. In 1989, some of the first regulatory regions of the CYP19A1 gene were identified by structural analysis, namely a putative TATA sequence located 23 bp upstream of the transcription start site and an AP1 site and cAMP and glucocorticoid regulatory elements [108]. In 1990, the CYP19A1 gene was found to be greater than 52 kb in size and to consist of 10 exons and 9 introns [109] (Fig. 4.3). The entire gene is now known to encompass some 123 kb, of which, 93 kb is an extended 5′ regulatory region.
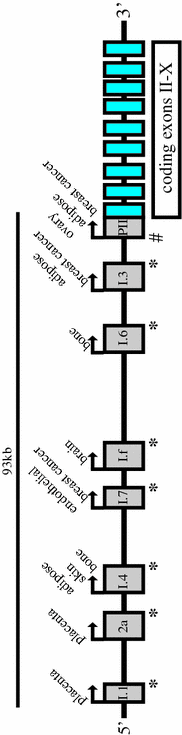
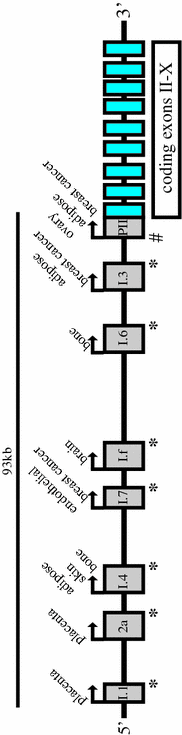
Fig. 4.3
CYP19A1 gene structure. The human aromatase gene, CYP19A1, is composed of nine coding exons (blue) and a number of untranslated first exons (grey) which are regulated in a tissue-specific manner. Each first exon has a splice donor site (*) which is spliced into a common splice acceptor site (#) such that coding region is identical irrespective of promoter used to drive expression
4.5 Promoter-Specific Regulation of Aromatase in Obesity and Breast Cancer
It is plausible that the increase in aromatase expression found in obese breast adipose results in part from the decreased differentiation of stromal cells to adipocytes in response to factors including PGE2 [110], TNFα [111] and IL-11 [112]. This would lead to the increased ratio of adipose stromal cells to fat cells which express little to no aromatase [113, 114]. However, it is clear that factors produced in obesity, including those which inhibit differentiation, alter the expression of aromatase in breast adipose stromal cells (Fig. 4.4) independent of effects on adipogenesis and this, via molecular mechanisms involving complex signaling pathways.
Much of the initial work pertaining to aromatase regulation in the adipose was undertaken before the CYP19A1 gene structure was even elucidated. In the early 1980s, a number of factors, including glucocorticoids and cAMP analogues, were found to stimulate aromatase activity in isolated human adipose stromal cells [115, 116]. These studies also examined the effect of serum on the glucocorticoid- and cAMP-mediated regulation of aromatase and interestingly, demonstrated that while serum potentiated the effects of dexamethasone, it inhibited the effects of cAMP. This was one of the first indications that glucocorticoids and cAMP must be acting via different pathways to regulate aromatase. Following on from these studies and once the cDNA for aromatase had been isolated, much information was gathered using Northern blot analysis. These studies added a new level of complexity when phorbol esters were found to potentiate the effects of cAMP to increase aromatase expression, and growth factors and inflammatory cytokines, including TNFα and IL-1β, inhibited the cAMP-mediated expression of aromatase [117]. At this stage, interest grew to elucidate the signaling pathways involved. The characterization of the CYP19A1 gene and the 5′-end of aromatase transcripts suggested that alternative promoters must be used to drive aromatase expression in different tissues, hence the term tissue-specific promoters. It was demonstrated, using 5′ rapid amplification of cDNA ends from an adipose-derived cDNA library, that promoter I.3-specific sequences were expressed in adipose tissue as well as in adipose stromal cells maintained under all tissue culture conditions, whereas the newly identified promoter I.4-specific transcripts were present only in breast adipose tissue and adipose stromal cells treated with glucocorticoids [118]. It was later shown that the majority of transcripts found in normal adipose, be it from the buttocks, thighs, abdomen and breast were derived from activation of promoter I.4 [119, 120], whereas adipose tissue from tumor-bearing breast had high levels of aromatase transcripts that were derived from the proximal promoters I.3/II, despite promoter I.4-specific transcripts also being increased [120]. The identification of these specific transcripts allowed additional studies into the promoter-specific regulation of aromatase by tumor-derived and obesity-associated inflammatory factors. In isolated human adipose stromal cells, stimulation with class 1 cytokines, including IL-6, IL-11, leukemia inhibitory factor (LIF) and oncostatin M caused an increase in promoter I.4-specific transcripts, whereas PGE2 stimulated promoter II-specific transcripts [121]. These results are corroborated by findings demonstrating that TNFα, IL-6 and COX-2 are positively correlated with aromatase transcript levels in breast cancer tissue [122].
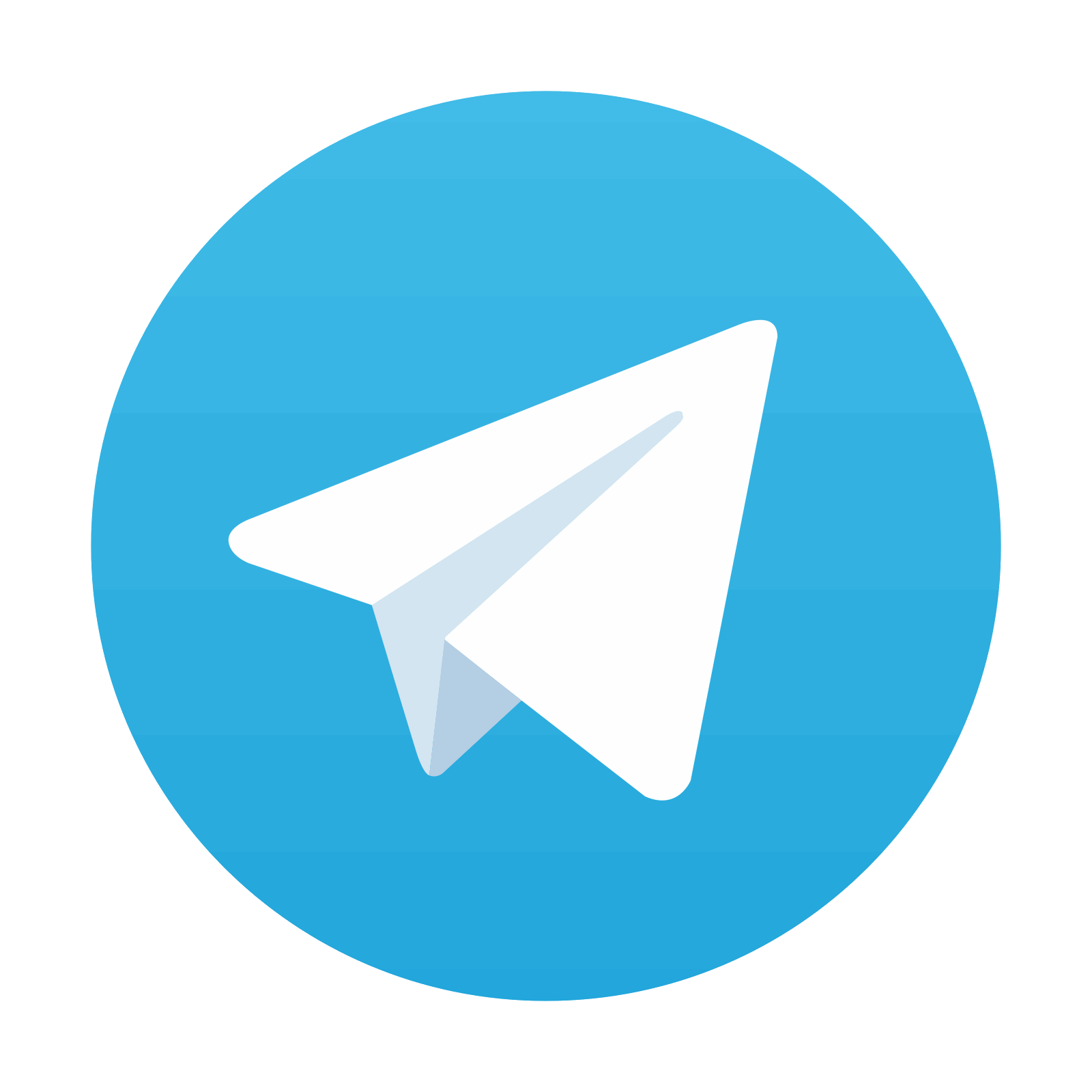
Stay updated, free articles. Join our Telegram channel

Full access? Get Clinical Tree
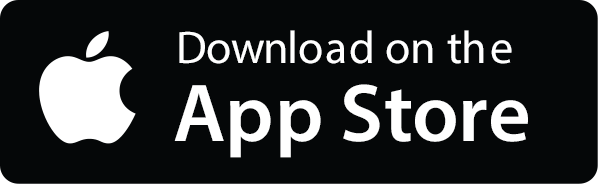
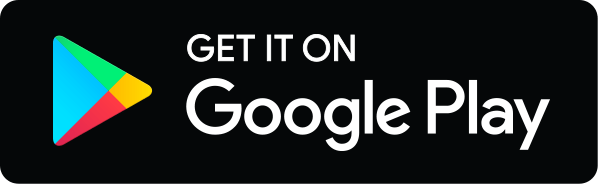