Fig. 15.1
US acute lymphocytic leukemia (ALL) and acute myeloid leukemia (AML) incidence rates (age-adjusted, 2000 US standard) by race and sex, diagnosed among residents of nine cancer registry areas of the Surveillance, Epidemiology and End Results Program, 1975–2007. (a) Age-specific rates (<15, 15–24, 25–34, 35–44, 45–54, 55–64, 65–74, 75+ years); (b) temporal trends (1975–1981, 1982–1988, 1989–1995, 1996–2001, 2002–2007)
Relative survival (RS) rates for individuals diagnosed in SEER-9 during 1975–2006 and followed through 2007 are considerably more favorable for patients with ALL than AML, with approximately 80 % and 60 % 1- and 5-year overall RS, respectively, for ALL, and 35 % and 15 %, respectively, for AML. Overall RS for ALL and AML is somewhat more favorable for females than males; overall ALL survival is slightly more favorable for whites than blacks, whereas the reverse is true for AML. Over the past three decades, overall ALL and AML survival has improved, with the greatest strides apparent among the youngest age groups. During 1975–1982, 5-year RS for ALL was 61 %, 22 %, 11 %, and 8 % for those <20, 20–39, 40–59, and ≥60 years, respectively, and this increased to 86 %, 41 %, 27 %, and 11 % in the corresponding age groups during 1999–2006. During these same two time periods, AML 5-year RS increased prominently from 22 % to 58 % among those <20 years, 13–51 % among those 20–39 years, and 10–34 % among those 40–59 years, with a less striking improvement of 3–7 % among those ≥60 years.
Risk Factors for Acute Leukemia in Children
Chromosomal translocations have been hypothesized to initiate pediatric leukemia in utero based on studies of leukemia arising in identical twins, investigations in newborn cord blood, and the short latency period characterizing pediatric leukemia [10]. Agents causing such translocations have not been identified, and it is suspected that additional molecular changes are required for pediatric leukemia to develop. Data supporting the requirement for additional events in pediatric leukemogenesis include twin concordance rates, results from animal studies, and the notably higher prevalence of leukemic fusion genes in normal newborns than the subsequent incidence rates for pediatric leukemia. Next, we summarize the epidemiologic findings on preconception and prenatal risk factors followed by results for postnatal leukemogenic factors.
Prenatal and Preconception Factors
Reproductive History
Long-standing efforts to identify reproductive factors associated with risk of pediatric ALL and AML have not yielded clearly established etiologic factors. Associations of prior maternal fetal loss with increased risk of acute pediatric leukemias [11, 12] have not been confirmed in recent studies of pediatric [13, 14] or infant [15] leukemia. Inconsistent findings also have been reported for increased risks in firstborns [13, 14, 16, 17], young maternal age at birth [13–15, 18, 19], and advanced maternal age [14, 20, 21], with the latter potentially affected by changing maternal birth cohort patterns [22].
Medical Conditions and Treatments
Decades of efforts to identify infectious agents causing pediatric ALL have been unsuccessful, and more recent studies of maternal medical conditions and/or treatments generally have not been replicated. It has long been postulated that a viral agent infecting a susceptible mother during pregnancy may play an etiologic role in pediatric leukemias [23]. However, reports linking in utero influenza infection [24] and Epstein–Barr virus [25] with pediatric leukemia and ALL, respectively, were not subsequently confirmed [26, 27], and specific leukemogenic infectious agents have not been identified [28–30].
Maternal hypertension has been linked with pediatric ALL [19] and AML [18], but the association has not been replicated, and no relationship has been found for preeclampsia [14] or gestational diabetes [18, 19]. Also requiring replication are findings of excess risks of pediatric common B-cell precursor leukemia in offspring of women with a previous molar pregnancy [14] and those receiving antibiotics [31] or mind-altering drugs during pregnancy [32], pediatric AML in offspring of mothers with polyhydramnios or anemia [14], and pediatric hematopoietic malignancies in offspring of mothers treated previously with infertility drugs [13, 33]. An excess of pediatric leukemia in mothers using marijuana before or during pregnancy [34] was not confirmed [35].
Medical Radiation Exposures
Diagnostic X-ray exposures from the late 1940s through the mid-1970s have been associated consistently with modest elevation in risk of pediatric leukemia, although risks have declined over time as radiation doses have decreased [36, 37]. Increased risk of pediatric leukemia was first linked with fetal exposure to abdominal or pelvic diagnostic X-ray of the pregnant mother more than 50 years ago [16]. Subsequently, additional data from the large Oxford Survey of Childhood Cancers, a medical record-based study in hospitals in the northeast United Kingdom (UK), and close to 30 other case–control studies support an overall RR of 1.4 (i.e., 40 % increased risk) for pediatric leukemia associated with diagnostic ionizing radiation exposure in utero, but the interpretation of the statistical association has been debated [37]. Data are insufficient to determine risks of pediatric leukemia in offspring of women undergoing radiotherapy for cancer, and there is no evidence linking ultrasonography during pregnancy with risk of pediatric leukemia [36].
Environmental Ionizing Radiation Exposures
Data evaluating leukemia risk with in utero exposure to environmental sources of ionizing radiation are limited. The cohort study of survivors who were in utero at the time of the atomic bombings of Hiroshima and Nagasaki “…cannot provide information on the effect of radiation on the incidence of childhood cancers” in the absence of complete information for the first 5 years of follow-up and insufficient size to assess such rare outcomes [38]. Similar limitations characterize a cohort of persons who were in utero at the time of the Chernobyl accident [39]. Existing data are not informative about pediatric leukemia risks in offspring of female airline crew, women living in proximity to nuclear testing or nuclear installations, or women exposed to residential radon, gamma, or other natural background radiation [40].
Parental Occupational Radiation Exposures
A small study linking paternal preconception occupational exposure of nuclear industry workers with excess leukemia and lymphoma risks in offspring [41] was not confirmed in a study of 39,557 children of male nuclear workers [42]. Furthermore, there was no excess of pediatric leukemia in offspring of female or male medical radiation workers in the UK [43] or the USA [44]. A meta-analysis of all studies of parental preconception occupational exposure to extremely low-frequency magnetic fields (ELF-MF) revealed a borderline significant increased risk of childhood leukemia in offspring (RR = 1.35, 95 % CI = 0.95–1.91) [45]. However, the studies in the meta-analysis included substantial heterogeneity, lacked detailed exposure data, and did not consider occupational chemical or other confounding exposures. In addition, the largest and most detailed epidemiologic study showed no association [45].
Parental Occupational Pesticide Exposures
Risks of leukemia in offspring of parents occupationally exposed to pesticides have been extensively investigated, but few investigations have identified the specific pesticides to which the fetus has been exposed. Meta-analysis of more than 30 case–control and five cohort studies revealed similarly increased risks for both ALL and AML (RR = 2.64, 95 % CI = 1.00–5.00; RR = 2.64, 95 % CI = 1.48–4.71, respectively) with maternal occupational exposure, with higher risks in studies of farm-related exposures compared with studies of mixed or unknown place of exposure [46–49]. No consistent association was observed for childhood leukemia with paternal occupational exposure to pesticides [48].
Parental Occupational Chemical Exposures
Findings from epidemiological studies of pediatric leukemia in offspring of parents exposed to heavy metals (including arsenic), polychlorinated biphenyls, dioxin, indices for outdoor air pollution, traffic density, drinking water disinfection by-products, and specified and unspecified solvents are inadequate to estimate risks [50] using definitions proposed by the US National Academy of Sciences [51]. Limited evidence has linked pediatric leukemia in offspring with paternal preconception exposure to motor vehicle emissions, driving or inhaled particulate hydrocarbons [52] or with maternal exposure to unspecified solvents [50].
Parental Cigarette Smoking
The role of maternal smoking in the development of pediatric leukemia has been evaluated for several decades, with a few studies reporting an increased risk. However, a meta-analysis [53] and subsequent studies concluded that maternal smoking during pregnancy is not a major risk factor for childhood acute leukemia [54]. Paternal preconception smoking has been investigated less extensively, but a recent meta-analysis of nine studies showed a modest association with childhood ALL (RR = 1.17, 95 % CI = 1.04–1.30) [55].
Parental Alcohol Consumption
A comprehensive assessment of pediatric leukemia risk and parental alcohol consumption in 33 case–control studies did not strongly support an association [56]. Paternal preconception consumption of alcohol also has not been linked with elevated risk [57]. However, a meta-analysis of 21 case–control studies concluded that maternal consumption of alcohol during pregnancy was associated with increased risk of pediatric AML (RR = 1.56, 95 % CI = 1.13–2.15), but not ALL [58]. Reasons why in utero exposure to alcohol increases AML but not ALL risk are unknown.
Maternal Diet and Vitamin Supplements
The possible role of maternal diet or vitamin supplements in risk of acute leukemia has only begun to be investigated systematically, and to date only a few studies have reported results. Three investigations found reduced risks of pediatric leukemia in offspring of mothers who consumed higher levels of fruits and vegetables during pregnancy [4, 59, 60]. Spector et al. found that maternal consumption of foods that inhibit DNA topoisomerase II was linked with increased risk of infant AML [4]. A recent meta-analysis [61] showed weak evidence of a reduced risk of developing childhood ALL associated with maternal folate use before pregnancy, and other studies also found reduced risk with maternal use of iron supplements [32, 62].
Postnatal Factors
Birth Weight
High birth weight has been linked consistently with increased risk of ALL, and recent studies suggest a similar relationship for AML. A meta-analysis of 31 studies demonstrated significantly increased risks of ALL (RR = 1.23, 95 % CI = 1.15–1.32) associated with birth weight of ≥4 kg, and seven of these studies showed a similar relationship for AML (RR = 1.40, 95 % CI = 1.11–1.76) [63]. It has been suggested that accelerated growth rather than high birth weight per se is etiologically linked with ALL [64], but further epidemiologic investigations are needed. Low birth weight (<1.5 kg) has been associated with increased risk of AML (RR = 1.49, 95 % CI = 1.03–2.15) but not ALL [63].
Breast-feeding
Large case–control studies examining the association of breast-feeding and risk of childhood acute leukemias have reported 9–20 % decreased risk for ALL, but a less consistent picture for AML, ranging from no reduction to 23 % reduction in risk [65, 66]. A meta-analysis found that ever having been breast-fed was associated with a 9 % reduced risk of ALL based on 17 studies and no reduction in risk of AML based on nine studies [67]. Although few studies have examined risks for combined breast-feeding and milk supplementation, one reported a moderately increased risk of childhood leukemia among those infants who received milk supplementation with breast-feeding more than 50 % of the time [68].
Medical Conditions and Treatments: Infections, Vaccinations, and Atopy
Clusters (a group of cases representing an excess intensity within the population-at-risk which is unlikely to be due to chance) of pediatric leukemia have been described for decades, but no infectious or other causal agent(s) have been linked with these clusters [69, 70]. A comprehensive, broad-based epidemiologic and laboratory investigation of the largest pediatric acute leukemia cluster to date, diagnosed in residents of Churchill County, Nevada, was unable to identify a causal agent [71].
Two mechanisms have been suggested by which infectious agents might be associated with the age peak from 2 to 5 years in the common form of pediatric ALL. Greaves postulated a two-hit model of leukemogenesis, with the first event or mutation occurring in rapidly dividing immature B cells in utero and the second event arising in early childhood as a result of delayed exposure to infectious agents [72]. Epidemiologic assessment of surrogate measures related to the timing of exposure to infectious agents, such as daycare attendance, birth order and interval between births, and the timing, frequency, and severity of infections in the first 2 years of life generally supports a reduced risk of ALL associated with early and substantial daycare attendance [73] and social activity in the first year of life [74], but findings for other measures are not as consistent [75]. However, findings of significantly more clinically diagnosed infectious episodes during infancy, particularly in the neonatal period, and earlier age of onset in children who subsequently developed ALL compared with controls suggest that a dysregulated immune response to infection increases risk of developing pediatric ALL [76]. Kinlen proposed that childhood ALL occurs as a rare response to a specific, albeit currently unknown viral agent(s), particularly when there is mixing of rural (or other low-density) populations with urban (or other high-density) populations [77]. Summarizing multiple extreme examples of population mixing in Britain, Kinlen observed significant short-term excesses of pediatric leukemia [78], although others reviewing the population mixing studies have disagreed with the conclusions drawn by Kinlen [79].
A limited number of studies investigating an infectious etiology for pediatric acute leukemia have reported a protective effect from vaccination in infancy or early childhood, with risks varying according to the type of vaccine and age at immunization [80]. A meta-analysis of epidemiologic studies examining atopy and risk of childhood leukemia revealed a 31 % significantly reduced risk of ALL based on six studies and no significant association for AML based on two studies [81]. Inverse associations were seen for ALL with asthma, eczema, and hay fever, but the authors caution about overinterpretation of the results in light of the limited number of studies, substantial heterogeneity, and potential misclassification.
Medical Radiation and Chemotherapy
In general, studies of low-dose postnatal diagnostic radiographic exposures (estimated organ doses range from 0.01 to 6 mGy) have not observed increased risks of pediatric leukemia; the results were based on questionnaire responses and estimated doses were not evaluated [82]. To date, there have been no reports assessing pediatric cancer risks in children who have undergone one or more higher-dose diagnostic imaging procedures, such as computed tomographic (CT) examinations (estimated organ doses from CT scans of the chest, abdomen, brain, spine, and face range from 10 to 80 mGy) or repeated radiologic examinations during the neonatal period, although studies of the former are underway.
Earlier cohort studies of infants or children irradiated for benign conditions were generally too small to estimate risk of pediatric leukemia accurately [83], but radiotherapy during childhood for tinea capitis was associated with a subsequent moderately increased risk of leukemia mortality (RR mortality = 2.3, estimated bone marrow dose = 30 mGy) [84]. Two of three earlier small studies found a dose–response relationship between estimated radiation dose to the bone marrow from radiotherapy for treatment of pediatric cancer and subsequent risk of secondary leukemia. A larger more recent investigation did not confirm this relationship; however, excess secondary leukemia was evident—likely due to chemotherapy treatment [85], a strong and well-established risk factor for acute leukemia. More information on the relationship between chemotherapeutic agents and acute leukemia risk is provided in the section of this chapter on adult leukemia.
Environmental Exposures: Ionizing Radiation
The follow-up investigation of Japanese atomic bomb survivors, the most important epidemiological study of radiation-exposed populations for quantitative risk assessment, demonstrated that survivors who were less than 10 years old at exposure had moderate-to-high relative risks for both ALL and AML [86]. Relative and absolute risks were higher among children than adults, and these risks peaked within 10 years. The absolute risk for incidence was estimated to decrease by about 5 % for each year’s increase in age, and risk for ALL among females was about 40 % of that among males.
Results from other studies of postnatal environmental ionizing radiation and risk of pediatric acute leukemias are less clear. Leukemia and lymphoma risks were found to be increased in persons under age 25 among populations living near nuclear fuel reprocessing or weapons production plants in the UK (specifically Sellafield and Dounreay), but not among populations residing close to nuclear plants generating electricity; however, environmental radiation levels measured in proximity to these facilities were considered too low to ascribe to the radiation exposures from these plants [87]. Residential exposure to radon was linked with elevated risk of pediatric ALL in an ecological study but was not associated with an increased risk in studies in the USA [88], Germany [89], or the UK [90]. Although there was no overall association of residential radon with pediatric AML, a borderline increase was observed in children ages 2 or older [91].
Environmental Exposures: Nonionizing Radiation
Initial reports of two- to threefold excess risks of pediatric leukemia in children residing in homes with high levels of 60-Hz magnetic field exposures from residentially proximate power lines were assessed further in large studies with more extensive and direct measurements. Results from pooled analyses of these high-quality studies revealed that pediatric leukemia risks were not increased among children residing in homes with power frequency magnetic field exposures under 0.4 μT (which included more than 99 % of residences internationally), but were twofold increased among the <1 % of children living in homes with exposures ≥0.4 μT [92]. Reasons for the increased risk are unclear, particularly since experimental studies have not linked power frequency magnetic fields with carcinogenesis [93].
Environmental Exposures: Chemicals
Residential use of insecticides demonstrated a dose–response relationship with total childhood leukemia [94] and ALL [95], but exposures to insecticides in agricultural settings were not associated with risk, nor was use of herbicides or fungicides in any setting [50]. Limited evidence supports a relationship of pediatric leukemia with childhood residential exposure to nearby high traffic density, car repair garages or gasoline stations [50], and with frequency of household use of petroleum products, solvents, and paint [5, 50, 96, 97]. No clear association was observed with pediatric leukemia risk and exposure to drinking water disinfection by-products, drinking water nitrate, residence near hazardous waste disposal sites, or exposure to environmental tobacco smoke [50].
Diet and Vitamin Supplements
Only a few small studies have assessed risk of pediatric leukemia with dietary components, and replication of the results is needed. Findings were mixed for consumption of cured meats [98, 99], and reduced risks were associated with intake of bean curd and vegetables in a study in Taiwan [99] and with consumption of foods containing vitamin C and/or potassium in a study in California, particularly during the earliest years of life [98]. Concern about a report linking intramuscular vitamin K with risk of pediatric leukemia [100] was dispelled following a pooled analysis showing no association [101].
Risk Factors for Acute Leukemia in Adults
Medical Radiation
Some data have linked childhood or adult diagnostic radiography with increased risk of adult-onset AML, but there is debate about whether the relationship is causal or due to underlying nonmalignant medical conditions requiring radiography or early manifestations of leukemia [83]. Use of the radiographic contrast medium, Thorotrast (an alpha-emitting contrast medium with a half-life of 400 years that was used in earlier time periods for cerebral angiography), has been consistently linked with an increased risk of myelodysplastic syndrome (MDS)/AML [102], with a cumulative estimated risk of 173 cases/10 [6] person-years [103]. Increased risk of AML has been associated with radiation treatment for ankylosing spondylitis (excess RR/Gy = 7.0 during 1–25 years after exposure) [104], and RRs ranging from 1.2 to 3.0 have been linked with radiation treatments for benign gynecologic disorders [105], tinea capitis [84], and peptic ulcer disease [106]. Individuals treated with radiotherapy for non-Hodgkin lymphoma, Ewing sarcoma, or cancer of the breast, uterine cervix, or uterine corpus have moderately increased risks of secondary AML, although the absolute risk is small [83]. Similar to the pattern for primary AML occurring among the Japanese atomic bomb survivors, initial cases of secondary AML following radiotherapy for another primary cancer often appear within 5 years of treatment (with most cases occurring within 10–15 years) and is associated with estimated bone marrow doses ranging from 1 to 15 Gy [107].
Chemotherapy
The development of therapy-related myeloid neoplasms, typically AML (t-AML), is a rare but highly fatal complication of cytotoxic treatments for both malignant and nonmalignant disease. Exposure to such treatments is associated with RRs ≥3 and lifetime cumulative risks ranging from <1 to 10 % for t-AML [108].
Specific cytotoxic agents differ in their leukemogenic potential and are associated with distinct morphologic and clinical characteristics as well as cytogenetic abnormalities, with similar findings among both children and adults [108–111]. Patients treated with alkylating agents are more prone to t-AML that is preceded by MDS, develops 5–7 years after exposure, and is characterized by loss or deletion of chromosome 5 and/or 7 [−5/del(5q),−7/del(7q)] and the presence of somatically acquired loss-of-function mutations in p53. In contrast, patients treated with topoisomerase II inhibitors, including the epipodophyllotoxins, are more prone to t-AML that is rarely preceded by MDS, arises after a latency of 2–5 years, and is characterized by balanced translocations involving the MLL gene at 11q23.
Antimetabolites used for treatment of malignancies (e.g., fludarabine) and for immunosuppressive therapy for autoimmune diseases and transplantation (e.g., azothioprine) also have been shown to increase risk of t-AML. Abnormalities in chromosomes 5 and/or 7, similar to t-AML associated with alkylating agents, have been described [108, 112]. In addition, children with aplastic anemia who were treated with recombinant human granulocyte colony-stimulating factor (rhG-CSF) combined with immunosuppressive therapy (e.g., cyclosporine) were at increased risk of transformation to acute myelomonocytic leukemia with monosomy 7 [113].
Although the vast majority of therapy-related leukemias are of myeloid lineage, increased risk of ALL following exposure to topoisomerase II inhibitors has been reported, also with 11q23 abnormalities [114]. With the introduction and increasing use of a number of new chemotherapeutic agents in recent years, future studies are needed to evaluate the acute leukemia risks and identify cytogenetic abnormalities associated with current treatments. In addition, more research is needed to evaluate the potential interaction between radiotherapy and chemotherapy.
Environmental Exposures: Ionizing Radiation
Compared with Japanese atomic bomb survivors exposed during childhood and adolescence, those exposed in adulthood had lower estimated relative risks of ALL, but the pattern was similar to that following childhood exposure, with risk peaking at less than 10 years since exposure, a declining risk for each year’s increase in age at exposure, and risks for women about half the level of risks for men [86]. For AML, relative risks were similar for males and females, but males had twofold higher absolute risks. A recent analysis suggests that excess risk of acute leukemia mortality continues to persist decades after the bombings [115]. The population living on the banks of the Techa River in the Southern Urals region in Russia, who received chronic low-dose-rate internal and external radiation exposures from releases of radionuclides into the river from the Mayak nuclear weapons plant plutonium production facility, experienced excess risk of leukemias other than chronic lymphocytic leukemia (i.e., non-CLL leukemias) and a significant dose–response relationship was observed (median bone marrow dose was 0.2 Gy, but doses ranged up to 2 Gy) [116].
Environmental Exposures: Nonionizing Radiation
Adult AML has not been significantly associated with residential exposure to ELF-MF [117].
Occupational Exposures: Radiation
Studies of radiation-exposed workers are important for clarifying effects of protracted radiation doses, since animal studies suggest that protracted low doses may allow for DNA repair and thus lower risks of leukemia or other cancers compared with risks associated with an acute, single radiation dose [83]. Medical radiation workers were the first population observed to develop radiation-related leukemia within a few years of the discovery of X-rays due to the very high radiation exposures of early workers [118]. Excess risks of leukemia have been the most consistently observed cancer-related cause of mortality in medical radiation workers, with incidence studies also confirming excess risks of non-CLL leukemias in those first working as radiologists or radiologic technologists before 1940 or 1950, but little evidence of increased risk in those first working after 1960 [118, 119]. Epidemiologic studies in nuclear workers who experience low, protracted radiation exposures have shown modest increases in risk for non-CLL leukemias, with a statistically significant dose–response [120, 121]. Moderate-to-high risks and significant dose–response relationships also were observed for non-CLL leukemias as well as CLL in Ukrainian Chernobyl cleanup workers (based on 71 total histologically confirmed leukemia cases diagnosed during 1986–2000 in a population of about 100,000 workers) [122]. Leukemia mortality risks were significantly elevated and risk rose significantly with increasing external radiation dose among workers at the Mayak nuclear complex in Russia where effects of plutonium exposures have been under investigation for years [123].
Occupational Exposures: Benzene
For more than 100 years, there has been recognition that occupational benzene exposure is related to risk of leukemia. A literature review identified nine cohort and 13 case–control studies that included estimates of benzene exposure, excluded ecologic or proportionate mortality methods, included a comparison group, and assessed risks for one or more subtypes of leukemia [124]. High RRs and a positive dose–response relationship were seen across study designs for AML, especially in more highly exposed workers in rubber, shoe, and paint industries. Data on ALL were judged to be sparse and inconclusive. A subsequent systematic review and meta-analysis of four studies focusing on cumulative exposure to benzene and AML found a clear dose–response pattern, with RRs of 1.94 (95 % CI = 0.95–3.95), 2.32 (95 % CI = 0.90–5.94), and 3.20 (95 % CI = 1.09–9.45) for low, medium, and high benzene exposure, respectively [125]. A large cohort study of benzene-exposed workers in China showed that risk of AML/MDS increases within 10 years of first exposure [126].
Occupational Exposures: Other Chemicals
Epidemiologic studies in industries with potential benzene and/or other solvent exposures (including chemical manufacturing workers, petroleum refinery workers, painters, automobile mechanics, truck and bus drivers, gasoline service station attendants, dry cleaners, chemists) or formaldehyde exposure (including furniture manufacturing workers, embalmers, and others) have found increased risks of total leukemia and/or AML; however, the number of leukemia cases in most of these studies has been small, exposure assessment generally has been limited, and potential confounders have not been evaluated, making it difficult to draw convincing conclusions about causality [7, 127].
Occupational Exposures: Farming
Increased risks of leukemias and lymphomas have been linked with farming, but relatively few studies have focused on risks of ALL or AML. Some data link occupation as a farmer with increased risk of ALL, but the data are not conclusive [7]. A few studies have examined risks of AML, and results have been mixed. The single study that examined farming practices in relation to AML found modest nonsignificant excesses with exposure to pesticides and weed killers [128]. One study among women linked residence on a farm with a small but significant excess of AML, but data were not available to determine if the women worked as farmers or to assess their exposures [129].
Cigarette Smoking
Cigarette smoking was first associated with risk of adult leukemia in the mid-1980s. A meta-analysis reported modest increases in risks of AML (RR = 1.3–1.4) based on findings from case–control and cohort studies [130]. Similar findings were observed in a recent large cohort study, with higher risks among current cigarette smokers (RR = 2.42, 95 % CI = 1.63–3.57 and RR = 2.29, 95 % CI = 1.38–3.79 for ≤1 and >1 pack per day, respectively) than among former smokers (RR = 1.29, 95 % CI = 0.95–1.75 and RR = 1.79, 95 % CI = 1.32–2.42 for ≤1 and >1 pack per day, respectively) compared with never smokers [131].
Hair Dyes
Although earlier studies had limited statistical power to evaluate the role of personal hair dye use and hematopoietic neoplasms [132], a subsequent investigation reported a modest association for ever use of permanent dyes (RR = 1.5, 95 % CI = 1.0–2.1) [133]. The association for hair dye use was stronger for longer duration of permanent hair dyes, than for nonpermanent hair dyes; the latter were not associated with increased risk of hematopoietic neoplasms [133].
BMI and Diet
In a meta-analysis based on nine cohort studies, high BMI or obesity was associated with increased risks of ALL and AML [134]. A limited number of studies have investigated dietary factors and AML [131, 135–137]. A large prospective cohort study identified higher meat intake and absence of coffee drinking with increased risk of AML [131]. Some investigations have not found an association of AML with fruit and vegetable consumption [131, 137]. Alcohol intake also has not been shown to be an important risk factor for adult acute leukemia [138, 139]. The few studies conducted to date along with methodologic issues related to dietary assessment, particularly in case–control studies, suggest that additional epidemiologic research is needed.
Hereditary and Genetic Aspects of Acute Leukemias
Familial Aggregation and Genetic Syndromes
Familial aggregation of acute leukemias is rare. Studies of the few pure familial AML pedigrees have identified germline mutations in RUNX1 and CEBPA [140]. Even fewer studies have described familial aggregation of ALL, but individuals having a twin with ALL have substantially elevated risk for ALL, particularly among monozygotic twins [141].
A much larger body of evidence regarding the hereditary component of acute leukemia derives from the study of patients with rare genetic syndromes, but these patients also account for only a small proportion of the total population burden of acute leukemia. Among children with rare inherited bone marrow failure syndromes, including Fanconi anemia, dyskeratosis congenita, congenital neutropenia, and Shwachman-Diamond syndrome, risk of AML is strikingly elevated—often more than 100-fold [142, 143]. The molecular events that predispose to AML among these individuals are not completely understood but are thought to differ by syndrome and involve defective DNA repair, shortened telomere length, and abnormal hematopoietic differentiation and proliferation [144]. Other hereditary conditions associated with increased risk of acute leukemia include Li-Fraumeni syndrome, ataxia–telangiectasia, Bloom syndrome, Noonan syndrome, and neurofibromatosis 1 [145–149].
Myeloid proliferations related to Down syndrome (trisomy 21) have unique morphologic, immunophenotypic, clinical, and molecular features [2]. Children with Down syndrome have a 100-fold risk of AML, as well as approximately 15-fold risk of ALL [150, 151]. Individuals with Down syndrome are disproportionately found to have somatic mutations in the hematopoietic transcription factor GATA1, which results in impaired hematopoietic cell differentiation [151].
Genetic Susceptibility
Numerous studies have investigated common genetic variation in germline DNA in relation to leukemia risk. Because of the importance of chemical carcinogens in leukemogenesis, most of the initial research focused on genes related to carcinogen metabolism, folate metabolism, and DNA repair. Overall, studies suggest modest support for the role of genes in these three pathways in the etiology of acute leukemia [152, 153], but the literature is characterized by inconsistent results, limited statistical power for individual studies, and investigation of a small number of genetic variants.
With recent advances in technology, it is now possible to interrogate the entire genome for common genetic variants that confer susceptibility to disease. Four such genome-wide association studies of childhood ALL have identified risk loci in or near several genes that regulate the transcription and differentiation of B-cell progenitors (IKZF1, ARID5B, and CEBPE) and the tumor suppressor gene CDKN2A, which is also frequently somatically altered in hematologic malignancies [154–157]. Although the leukemia risks associated with these loci are low, these inherited genetic susceptibilities can be common in the general population. Additional research is needed to understand the biological basis of these findings, identify other genetic loci that confer acute leukemia risk, and investigate the potential interaction of genetic susceptibility with environmental and individual risk factors [158].
Chromosomal and Genetic Abnormalities
Recurrent chromosomal and genetic abnormalities are a hallmark of acute leukemia, with specific molecular characteristics often reflecting distinct clinical subsets of disease [159–161]. Most patients with ALL demonstrate an abnormal karyotype [161]. The Philadelphia chromosome t(9;22) is the most common rearrangement in adult ALL and has been associated with adverse prognosis. In contrast, high hyperdiploidy (51–65 chromosomes) and the t(12;21) translocation are the most common cytogenetic abnormalities in childhood ALL and have been associated with favorable prognosis. Approximately half of patients with AML demonstrate an abnormal karyotype, with a subset of patients having a complex karyotype with multiple abnormalities [159, 160]. The most common abnormalities include trisomy 8 and 21, monosomy 5 and 7, and various rearrangements such as t(8;21), t(15;17), and inv(16). Patients with a normal karyotype typically harbor recurrent mutations, frequently in NPM1 and FLT3. In contrast to childhood disease, the most common abnormality in infant ALL and AML is 11q23 translocation involving the MLL gene.
The diverse patterns of molecular characteristics may reflect etiologically as well as clinically and biologically distinct subsets of leukemia. The strongest evidence for this theory comes from t-AML, where different cytotoxic agents are associated with distinct molecular patterns, as described previously [109, 162, 163]. Studies also have suggested that chemical exposure may be associated specifically with ras mutation-positive AML [164, 165]. Identification of the timing of occurrence in addition to the specific type of chromosomal and genetic abnormalities also may provide insight into acute leukemia etiology. Recognition that the 11q23 rearrangement, the most common abnormality in infant acute leukemia, is characteristic of exposure to topoisomerase II inhibitors in t-AML has led to the investigation of maternal exposure to dietary and environmental inhibitors of topoisomerase II in relation to infant leukemia risk [4, 166]. A prenatal origin also has been demonstrated for the t(8;21) in patients with childhood AML [167], which may be related to prenatal pesticide exposure [168], whereas the t(1;19) in ALL has been shown to occur postnatally [169]. It will be critical to evaluate risk factors for molecular subtypes of the acute leukemias, but efforts to assemble large numbers of molecularly well-characterized acute leukemia patient populations will likely necessitate establishment of international consortia.
Summary
Causes of the acute leukemias in children and adults have not been fully identified, but recent progress has provided new etiologic insights. The most well-established risk factors for acute leukemias include ionizing radiation, benzene, and cytotoxic chemotherapy, with increasing risks associated with increasing doses. Among children, risks of pediatric leukemia (ALL and AML) are modestly increased with maternal in utero exposures to diagnostic X-rays in earlier decades, moderately increased with radiotherapy treatments, and moderate-to-highly increased with childhood exposure to the atomic bombings. Among persons exposed as adults, acute leukemia risks are moderately increased among atomic bomb survivors, individuals living near the Mayak nuclear weapons plant, and patients receiving radiotherapy treatments, and AML risks are moderate-to-highly increased with occupational benzene exposure. High risks of AML occur among children and adults receiving cytotoxic treatments (e.g., alkylating agent and epipodophyllotoxin chemotherapy) for benign and malignant disease.
Although pediatric ALL has long been thought to have an infectious etiology, no infectious agent has been isolated. However, recent research has identified modest associations with other environmental, lifestyle, and medical factors for all acute leukemias. Pediatric leukemia has been linked with prenatal maternal occupational pesticide exposure (ALL and AML) and postnatal residential use of insecticides (ALL only). Modest associations have been reported for pediatric AML with maternal prenatal alcohol consumption, pediatric ALL with paternal preconception smoking, and adult AML with cigarette smoking. Anthropometric measures also have been associated with both ALL and AML in children (high birth weight) and adults (high body mass index). Dietary factors may play a role in both pediatric and adult leukemia, but more studies are needed to clarify associations. Interestingly, pediatric ALL risk appears modestly reduced among children who were breast-fed or have a history of atopy.
Recent progress has been made in understanding the germline mutations underlying familial AML, the molecular events by which rare genetic syndromes predispose to the acute leukemias, common genetic variants that confer increased susceptibility to the acute leukemias, and agents causing recurrent chromosomal and genetic abnormalities associated with acute leukemia molecular subtypes. Although insights into etiology to date have facilitated some prevention efforts for the acute leukemias, additional epidemiologic work is needed to further impact the disease burden worldwide.
References
1.
2.
Swerdlow SH, Campo E, Harris NL, et al., editors. World Health Organization classification of tumours of haematopoietic and lymphoid tissues. 4th ed. Lyon: International Agency for Research on Cancer; 2008.
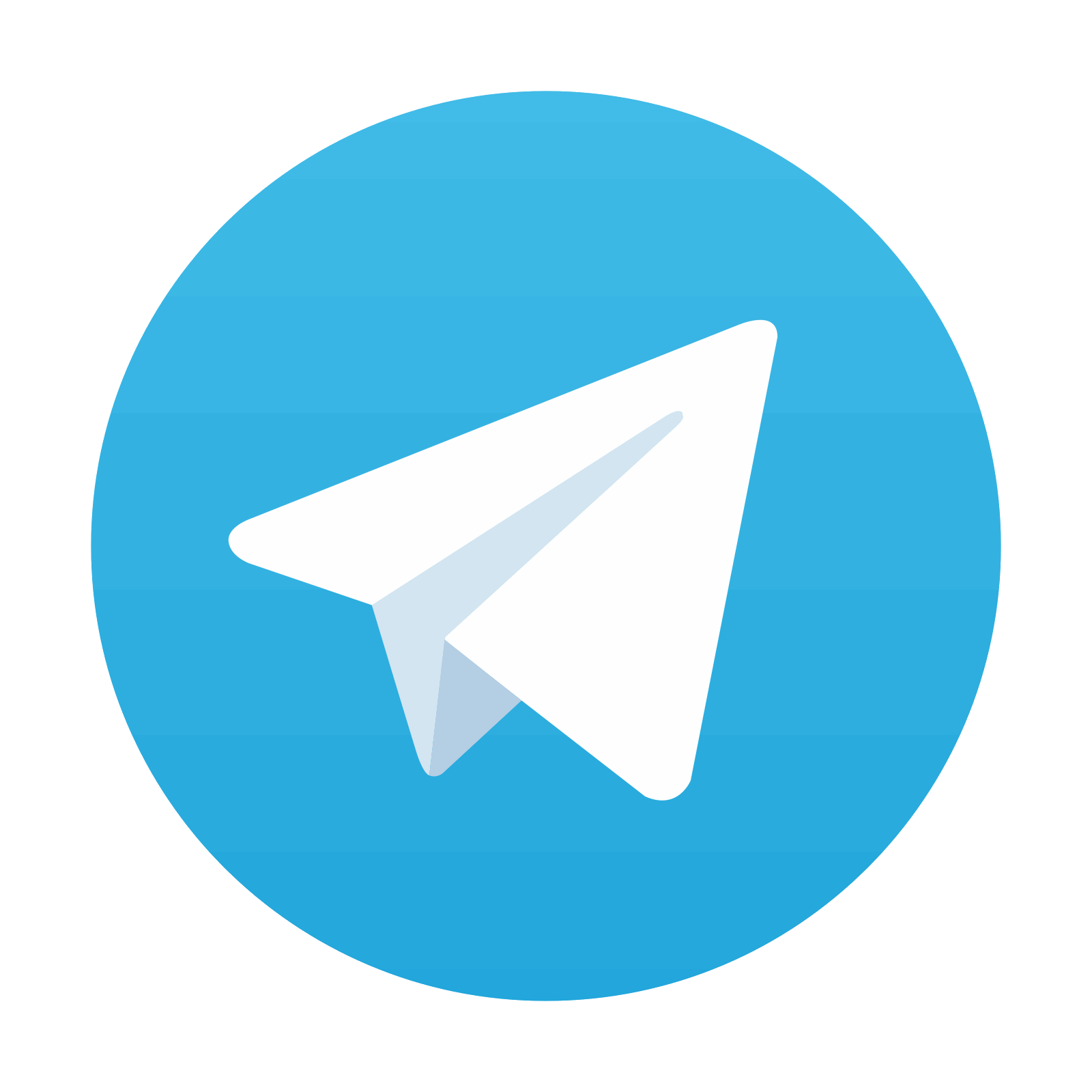
Stay updated, free articles. Join our Telegram channel

Full access? Get Clinical Tree
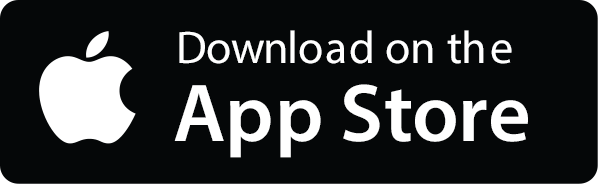
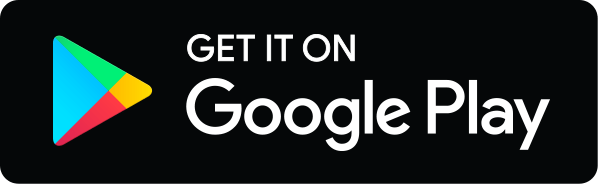