Fig. 9.1
Left cerebellopontine angle ependymoma (upper left), IVth ventricle ependymoma (upper right), spinal cord ependymoma (lower left), and right fronto-parietal ependymoma (lower right)
EPN accounts for 6–10% of brain tumors in children. At presentation, current standard initial treatment for children with EPN consists of maximally safe surgical resection, with the goal of gross total resection (GTR), and postoperative standard fractionated radiation therapy (RT) for microscopic residual tumor (Hoffman et al. 2014). By using this approach, 5-year overall survival (OS) and event-free survival (EFS) of 86% and 55%, respectively, have been achieved (Gajjar et al. 2013). EPN can spread locally into adjacent structures or via the cerebrospinal fluid (CSF) throughout the subarachnoid space (drop metastasis) (Paulino et al. 2002), especially in the case of high-grade tumors. “Sugar coating” of the meninges does not constitute metastatic disease and is likely an inflammatory process. EPN metastases most often appear to be nodular (Fig. 9.2).


Fig. 9.2
Supratentorial ependymoma preoperative (upper row) MR images; postoperative (middle row) MR images showing gross-tumor volume (yellow), clinical target volume (orange), and planning target volume (red); postoperative contrast-enhanced treatment planning CT (lower row) showing target volumes as outlined on MR
The clinical behavior of ependymal tumors is highly variable, and approximately 40% of patients might not be cured because of the paucity of effective and easily available treatment options (Merchant 2009). Surgical resection is the first line of treatment. Several studies have shown the benefits of adjuvant radiotherapy although the ideal volume of irradiation remains controversial. The role of chemotherapy, however, is uncertain, with little evidence supporting its use (Vaidya et al. 2012).
The prognosis for pediatric EPN remains poorer than that for other brain tumors. Definitive prognostic factors include extent of tumor resection, presence of metastases at initial diagnosis, and age at presentation (Reni et al. 2007; Shim et al. 2009). The significance of factors such as tumor location and histopathologic grade and the role of adjuvant therapy remain unclear (Reni et al. 2007; Shim et al. 2009). Research is currently focused on the molecular subtyping of EPN to define diverse subgroups and more accurately predict the expected behavior of each subgroup (Vaidya et al. 2012).
9.2 Prognostic Factors
9.2.1 Age at Presentation
Most studies on pediatric EPN have reported younger age at presentation as an adverse prognostic factor. In three studies, the 5-year OS was 22–42% in children aged 3 years or younger compared with 69–75% for those older than 3 years (Paulino et al. 2002; Perilongo et al. 1997; Pollack et al. 1995). Delay in diagnosis due to nonspecific signs or symptoms (Tamburrini et al. 2009), more aggressive tumor biology (Nazar et al. 1990), delay or avoidance of irradiation (Duffner et al. 1998), and lower radiation doses (Duffner et al. 1998) can affect results in younger children.
9.2.2 Extent of Surgical Resection
The extent of resection is the single most important prognostic factor for EPN (Paulino et al. 2002; Perilongo et al. 1997; Pollack et al. 1995; Duffner et al. 1993; Massimino et al. 2004; Merchant et al. 2009; Robertson et al. 1998; Rousseau et al. 1994). Advances in surgical techniques, such as operating microscopes and image-guided resection, have led to improvement in patient outcomes. A study reported that the 5-year progression-free survival (PFS) was 51–79% for patients who underwent complete resection compared with 9–44% for those who underwent subtotal resection (STR) (Vaidya et al. 2012). The fact that more than 90% of tumor recurrence occurs locally highlights the importance of complete resection (Nazar et al. 1990; Chiu et al. 1992).
9.2.3 Site of Primary Tumor
The ability to resect a tumor is highly dependent on the tumor site within the central nervous system (CNS). In a review of ten studies reporting 307 patients, complete resection was achieved in 53% of 128 patients with ST-EPN (Bouffet et al. 1998). Complete resection was achieved in only 29% of the 179 children with infratentorial lesions. Spinal cord tumors, however, were excised without affecting function in only 27–45% of patients. This disparity arises because surgical resection is more difficult for PF-EPNs because of frequent involvement of the brainstem and multiple cranial nerves (Tamburrini et al. 2009). Higher rates of complete resection for ST-EPNs likely explain the better prognosis and disease-free survival rates for children with these tumors (Vaidya et al. 2012).
The current standard of care for EPN includes maximal safe surgical resection, followed by focal radiotherapy (Merchant 2009; Merchant et al. 2009). Several reports indicate that there was no tumor recurrence in a subset of patients with completely resected ST tumors even in the absence of adjuvant therapy (Venkatramani et al. 2012), which underscores the need for better stratification of patients. Furthermore, although adjuvant chemotherapy continues to be a part of many trial protocols, especially in young children for avoiding or delaying RT, several clinical trials have found no survival benefit of adding chemotherapy at the time of primary diagnosis or at recurrence (Duffner et al. 1993; Bouffet et al. 2009; Bouffet and Foreman 1999).
9.2.4 Histopathologic Grade
Accurate histopathologic diagnosis according to the World Health Organization (WHO) classification for CNS tumors (Ellison et al. 2011; Louis et al. 2007; Pajtler et al. 2015) is challenging for ependymal tumors. Distinction between grade II EPN and grade III anaplastic EPN is often difficult, with poor interobserver reproducibility, even if performed by experienced neuropathologists (Ellison et al. 2011). Grade I EPN, or myxopapillary (occurring in the spine) and subependymomas (SEs; occurring across all compartments), generally have more readily distinguishable histopathologic characteristics. However, the grading of EPNs can be complicated because many tumors show isolated areas, each representing distinct grades, which presents a challenge to predict which tumor component will influence the overall biologic behavior (Pajtler et al. 2015).
The role of histopathologic grade as a prognostic factor remains contradictory. A review reported that the 5-year OS was 10–47% for patients with anaplastic EPN and 55–87% for those with low-grade tumors (Reni et al. 2007). Contrary to this, some studies have reported no differences in survival—or even an opposite survival trend—for patients with anaplastic EPN or low-grade tumors. Two studies reported a 5-year PFS of 78% and 46% for patients with anaplastic tumors and a 5-year PFS of 17% and 57% for those with low-grade tumors (Robertson et al. 1998; Rousseau et al. 1994). Classification of EPN tumors by molecular subtype is likely to obviate the need for conventional histopathologic classification in the coming years.
9.2.5 Molecular Prognostic Factors
Despite the histopathologic similarities among variants of EPN at different anatomic sites, the molecular biology of EPN remains heterogeneous and is associated with distinct genetic and epigenetic alterations as well as diverse transcriptional programs (Carter et al. 2002; Dyer et al. 2002; Korshunov et al. 2010; Mack et al. 2014; Mendrzyk et al. 2006; Parker et al. 2014; Wani et al. 2012; Witt et al. 2011). Functional cross-species studies reveal that these molecular differences reflect regionally discrete cells of origin (Parker et al. 2014; Johnson et al. 2010; Taylor et al. 2005). An association between neurofibromatosis type 2 (i.e., germline mutations in the NF2 gene) and sporadic mutations in NF2 has long been known as a hallmark genetic aberration in spinal EPN (Ebert et al. 1999; Rubio et al. 1994).
Other immunohistochemical markers have not adequately reflected the biologic heterogeneity of EPN and cannot reliably distinguish between histologic grades and subgroups of EPNs. The only molecular marker that has been consistently associated with unfavorable outcome is gain of chromosome arm 1q (Korshunov et al. 2010; Mendrzyk et al. 2006; Godfraind et al. 2012; Kilday et al. 2012; Modena et al. 2012), particularly in childhood PF-EPN. Homozygous deletion of the CDKN2A/B locus is another marker associated with inferior prognosis, mainly in ST-EPN (Korshunov et al. 2010).
Recent large-scale genomic and epigenomic studies have revealed the first driver genes in ST-EPNs. Fusions between RELA, which encodes an NF-kB component, and the poorly characterized gene C11orf95 resulting from chromothripsis (local chromosome shattering) on chromosome 11 occur in more than 70% of patients with ST-EPNs (Parker et al. 2014). Strikingly, the C11orf95–RELA fusion alone can drive tumorigenesis when aberrantly expressed in neural stem cells (Parker et al. 2014).
For PF-EPNs, two distinct molecular subgroups were consistently identified in two independent studies that used different methods and nonoverlapping patient cohorts (Wani et al. 2012; Witt et al. 2011). These subgroups [provisionally termed PF Group A (PFA) and PF Group B (PFB)] are associated with distinct transcriptomic, genetic, epigenetic, and clinical features and are much more informative than WHO grading alone (Archer and Pomeroy 2011).
In 2015, Pajtler et al. used genome-wide DNA methylation patterns to identify nine distinct molecular subgroups of ependymal tumors across all age groups (three subtypes in each anatomic compartment of the CNS) (Pajtler et al. 2015):
Spine (SP), with subtypes SE, MPE, and EPN (with NF2 association), all associated with excellent OS and PFS
PF, with subtypes SE, EPN-A, and EPN-B
ST, with subtypes SE, EPN-YAP1, and EPN-RELA
These molecular subgroups are genetically, epigenetically, transcriptionally, demographically, and clinically distinct. Whether they also have different cells of origin, as suggested by Johnson et al. (2010), remains to be proven in further functional studies. A biologic classification might help researchers and clinicians to better understand the heterogeneity of this disease as compared with the epigenetic subgroups of medulloblastoma (Kool et al. 2012). For example, Pajtler et al. showed that patients in the PF-EPN-A and ST-EPN-RELA (C11orf95–RELA fusion) subgroups had dismal outcomes with current treatment approaches. These patients were in the high-risk category, with a 10-year OS of approximately 50% and a 10-year PFS of approximately 20% (Pajtler et al. 2015).
9.3 Radiation Therapy
9.3.1 Radiation Dose
There is sufficient evidence to show that adjuvant postoperative RT, when compared to surgery alone, improves local control and is associated with a more favorable prognosis. Although data from prospective randomized trials are scarce largely due to rarity of the tumor, multiple retrospective studies have demonstrated that adjuvant RT improves local control as well as OS in patients with EPN. Thus, RT is currently considered to be standard adjuvant therapy after the resection of intracranial EPN (Chan and McMullen 2012; Stuben et al. 1997). The total dose varies from 45 to 54 Gy to the tumor bed in 1.5–1.8 Gy/fraction. Boost doses of approximately 10Gy have been recommended for macroscopic disease in some studies (Reni et al. 2007; Stuben et al. 1997). Two studies showed that higher radiation doses can improve outcomes in intracranial EPN: the 5-year OS for patients given a dose of >50 Gy was 58% and 51%, compared with 33% and 18% for those given a dose of ≤50 Gy (Chiu et al. 1992; Goldwein et al. 1990). Dose escalation to 66Gy by using hyper-fractionated RT was safe but was not associated with an improvement in outcome (Conter et al. 2009).
A total dose of 54 Gy is widely considered as the minimum dose required for local tumor control with gross residual and tumor bed concentrations of microscopic disease. Higher doses are considered to be more efficacious based on the first principles of RT and our understanding that local failure is a dominant component of first failure. The standard RT dose for patients without residual disease is 54–59.4 Gy in 1.8 Gy/fraction, and a more recent series used 59.4 Gy at 1.8 Gy/day for all patients except those under the age of 18 months who underwent GTR and had been treated with a dose of 54 Gy.
9.3.2 Irradiation Volume
A controversial aspect in the RT management of pediatric EPN is determining the appropriate field size and volume for irradiation [local field or craniospinal irradiation (CSI)]. CSI involves prophylactic irradiation of the entire craniospinal axis, with additional focal boost to tumor sites (Merchant and Fouladi 2005).
Previous studies showed that EPN had a predilection for leptomeningeal failures with high rates of metastatic seeding (32%) (Nazar et al. 1990; Merchant et al. 1997; Salazar et al. 1975). Consequently, CSI was the standard RT approach used for EPN for many years. These earlier studies had flaws and biases, including autopsy findings of seeding in patients who died because of local disease. Another problem was that these studies were largely conducted before the introduction of magnetic resonance imaging (MRI), when the extent of disease was not accurately known. The improvement in imaging techniques to adequately stage the craniospinal axis and modern patterns of failure studies has shown that isolated spinal failures for intracranial EPN are rare even in the absence of CSI (Perilongo et al. 1997; Rezai et al. 1996; Taylor 2004). More recent studies have reported seeding rates of only 3–10% (Pollack et al. 1995; Merchant et al. 1997; Vanuytsel and Brada 1991; Wallner et al. 1986). Therefore, the majority of spinal failures will occur in patients with pre-existing local failure (Rezai et al. 1996; Sutton et al. 1990). Reviews have confirmed that patients with localized disease do not require prophylactic CSI irradiation, because more than 90% of the recurrence occurs locally (Nazar et al. 1990; Chiu et al. 1992) and the additional morbidity associated with CSI cannot be justified. Even for anaplastic and infratentorial EPN, which are associated with a poor prognosis, prophylactic CSI has not resulted in a survival benefit (Timmermann et al. 2005). Thus, local-field RT has become the standard treatment volume for intracranial EPN (Chan and McMullen 2012).
Defining the clinical target volume (CTV) for local-field RT in EPN has been the subject of intense debate. A large study by Merchant et al. established that a 1-cm CTV margin around the tumor cavity to account for the microscopic extent of disease is sufficient to achieve high levels of local control in the setting of GTR (Merchant et al. 1999). This 1-cm margin was the established CTV margin used in the recent Children’s Oncology Group (COG) trial ACNS0121. Interestingly, patterns of failure and treatment effects data from St. Jude Children’s Research Hospital (St. Jude) indicate that a smaller CTV margin can likely allow adequate local control and possibly reduce neurocognitive late effects (Merchant et al. 2002a). The COG trial ACNS0831 is investigating a further reduction in treatment volume to a 5 mm CTV to 54Gy and no CTV expansion for the final boost treatment to 59.4 Gy (Chan and McMullen 2012) (Table 9.1).
Table 9.1
Clinical target volume margins by clinical trial
Clinical trial (sponsor) | CTV margin | Month/year of activation and identifier (clinicaltrials.gov) |
---|---|---|
ACNS0121 (COG) | Post-op tumor bed +1.0 cm | August 2003 |
NCT00027846 | ||
SJYC07 (St. Jude) | Post-op tumor bed +0.5 cm | November 2007 |
NCT00602667 | ||
ACNS0831 (COG) | Post-op tumor bed +0.5 cm | March 2010 |
NCT01096368 |
9.3.3 Imaging and Treatment Planning
EPN can exhibit heterogeneous enhancement (i.e., portions of the gross tumor might not show enhancement). Both T1 and T2 MRI might be required to adequately establish the full extent of the target. Given the significant anatomic distortion after surgery for EPNs, particularly those in the PF, proper definition of the resection cavity must include review of preoperative and postoperative MRI to include the full extent of the initial disease within the gross target volume (Chan and McMullen 2012).
The combination of computed tomography planning and MRI has some limitations in the PF, and some common artifacts in that region can lead to misinterpretation of the target definition. Alignment of the spinal cord can be problematic when diagnostic images are acquired in a position different from that used for treatment. MRI to identify the target should be performed in the same simulation position to improve the quality of image fusion and target delineation.
9.3.4 Very Young Children
The treatment of very young children with primary brain tumors is particularly challenging. For example, in very young patients with medulloblastoma, standard management is chemotherapy to delay CSI until the patient reaches 3 years of age. The same approach has been applied to EPN. However, a delay in RT, even in the presence of chemotherapy, tends to increase the risk of tumor recurrence. The St. Jude RT-1 trial showed that patients who received pre-radiation chemotherapy had a poorer 3-year PFS than those receiving immediate adjuvant irradiation (49% vs. 84%, respectively) (Merchant et al. 2002b). Patients with EPN who are younger than 3 years can be treated with RT at an early age without severe toxicity (Merchant et al. 2005). EPN has a more limited treatment volume and tends to occur in the PF, thus allowing the sparing of most structures involved in higher cognitive function. In the recently completed ACNS0121 trial, patients aged 1 year and older could receive upfront conformal RT (Chan and McMullen 2012).
The need for adjuvant RT in patients with completely resected ST-EPN is also under investigation. Published series of spinal EPNs show that complete resection alone is often sufficient to achieve long-term local control (Ferrante et al. 1992; Hanbali et al. 2002). In contrast to fourth ventricular EPN where GTR challenging due to the proximity of the lower cranial nerves and adjuvant RT likely helps sterilize microscopic residual disease, ST tumors, except for those involving eloquent brain regions, the anatomic constraints of surgery are fewer and GTR rates are much higher. A small series from the Beth Israel Medical Center, New York, suggested that long-term local control can be achieved by GTR alone (Hukin et al. 1998). In the ACNS0121 trial, patients with completely resected low-grade ST-EPN were assigned to the observation arm (no RT). Preliminary results from 11 patients in the observation arm showed that 2 patients had relapses within the first 12 months, both of whom were salvaged with surgery and RT and remain disease free (ACNS0831 Protocol 2011. (https://members.childrensoncologygroup.org/Prot/ACNS0831/ACNS0831DOC.pdf). Accessed 04/22/2016. Children’s Oncology Group 2011). This strategy has been retained in the ACNS0831 trial.
9.3.5 Spinal Ependymoma
The epidemiology, pathologic characteristics, and behavior of spinal EPN are distinct from those for cranial EPN. Myxopapillary ependymoma (MPE) is the predominant histologic variant in spinal EPN. These tumors appear to be less common in children than in adults. In the pediatric population, MPE is usually seen in adolescents. MPE represents 13% of ependymal tumors (Chao et al. 2011).
MPE was first recognized as a distinct histologic variant of EPN by Kernohan (1932). The designation “myxopapillary” is based on the histologic appearance of MPE. MPEs produce mucin and, because of their branching vasculature, form tumor cells arranged in papillae (Pica et al. 2009; Sonneland et al. 1985). MPEs are categorized as grade 1 tumors by the WHO and are considered benign tumors characterized by slow, indolent growth and a long disease course (Al-Halabi et al. 2010). In general, MPEs arise in the lumbosacral spine, specifically in association with the conus medullaris, cauda equina, or filum terminale (Chao et al. 2011; Bagley et al. 2007). MPEs rarely arise at other sites in the spinal cord or outside the neuroaxis (Akyurek et al. 2006). Most patients with MPE are male, and MPEs are diagnosed in the third or fourth decade of life (Bagley et al. 2007).
MPEs are also capable of distant spread. One third of treatment failures occur at distant sites with or without a primary site failure (Akyurek et al. 2006). MPEs are rare but more aggressive in children. They are associated with higher incidences of intracranial and spinal dissemination (Pica et al. 2009; Sonneland et al. 1985; Bagley et al. 2007; Akyurek et al. 2006; Merchant et al. 2000). A study from St. Jude reported a higher-than-expected rate of subarachnoid dissemination at presentation in patients with MPEs, which resulted in the use of craniospinal RT rather than involved-field radiation in three of four patients (Merchant et al. 2000).
Maximal safe resection is considered the standard of care for MPE. Currently, there is no clearly defined role for adjuvant RT although it has been recommended after STR. Some studies recommend adjuvant RT for all patients after surgery, regardless of the extent of resection (Al-Halabi et al. 2010; Akyurek et al. 2006). Other studies advocate adjuvant RT for patients receiving piecemeal GTR as opposed to en-bloc resections, based on the increased rates of local recurrence (Volpp et al. 2007). In general, it is very difficult to determine the extension of resection at this site. Because of the rarity of MPE in children, most studies have been limited to retrospective analysis. The primary treatment is GTR, with no clearly defined role for adjuvant RT. Conservative management without the use of adjuvant RT in pediatric patients with a high risk of craniospinal dissemination can sometimes result in the need to give RT with a more extended field.
In a recent retrospective study by the Johns Hopkins Hospital, all children with spine EPN who underwent either GTR or STR were evaluated. All patients receiving RT were treated at the involved site with a median dose of adjuvant RT of 50.4 Gy (range, 45–54 Gy). After a median follow-up of 7.2 years, local control rates at 5 and 10 years were 62.5% and 30%, respectively, for the group undergoing surgery alone versus 100% at both time points for those undergoing surgery and adjuvant RT. In addition, 50% of patients receiving surgery alone had local failure. Local failure occurred in all patients receiving STR compared with 33% of patients receiving GTR alone. One patient in the surgery and adjuvant RT group had recurrence at a distant site 1 year after diagnosis (Agbahiwe et al. 2013).
9.3.6 New Radiotherapy Modalities
In a phase II trial, 88 pediatric patients receiving three-dimensional conformal RT (77 receiving a dose of 59.4 Gy and 15 patients under the age of 18 months receiving a dose of 54 Gy) showed a higher 10-year PFS (69%) (Merchant et al. 2004) than that reported in older studies for patients receiving conventional RT (31–46%). A potential bias in the phase II study, however, was the high (84%) rate of GTR. The study also reported better preservation of neurocognitive function in those receiving conformal RT than in those treated with conventional radiation therapy (Figs. 9.3 and 9.4). A study evaluating adjuvant fractionated stereotactic RT in 80 patients (mean total dose 52.2 Gy, range 50.4–58 Gy) reported a high 5-year PFS of 87% (Combs et al. 2006). However, radiosurgery has not yet been established as a standard adjuvant therapy for EPN (Chan and McMullen 2012).



Fig. 9.3
Treatment planning CT with dose display (>10 Cobalt-Gray Equivalent) for proton therapy plans using passively scattered (left) and pencil-beam scanning methods (right)

Fig. 9.4
Intensity-modulated photon (left, top, and bottom) and proton (right, top, and bottom) therapy plans displaying dose distributions greater than 40 (top row) or 10 (bottom row) Gray or Cobalt-Gray Equivalent, respectively
9.3.7 Intensity-Modulated Radiation Therapy
Recent studies show that treatment of a local field does not compromise local control and survival in patients with EPN. Intensity-modulated radiation therapy (IMRT) has been used over the last 12 years to treat EPN in an effort to spare surrounding normal tissues from high doses of radiation. Given that the volume adjacent to the target receives less radiation, there are concerns that IMRT might compromise local control. However, the local control and survival rates for patients receiving IMRT are comparable with those for patients who received previous therapies that used larger treatment volumes (Schroeder et al. 2008). All failures have been reported within the high-dose region, suggesting that IMRT does not diminish local control (Merchant et al. 2002a; Schroeder et al. 2008).
9.3.8 Proton Therapy
Approximately 66% of intracranial childhood EPNs occur in the PF, arising along the lining of the fourth ventricle (Smyth et al. 2000). These tumors often extend to the cerebellopontine angle through the foramina of Luschka or dorsally through the foramen of Magendie, thus placing the tumor in close proximity to critical structures such as the brainstem, cranial nerves, cochlea, and temporal lobes. Proton therapy appears to offer a better sparing of surrounding critical structures (e.g., optic chiasm, cochlea, hypothalamus, pituitary gland, and pharynx) than does IMRT (MacDonald and Yock 2010; Timmermann et al. 2007). For ST-EPNs, proton therapy appears to spare the more eloquent and cognitive areas. Proton therapy offers the advantage of lower radiation doses to organs at risk (MacDonald and Yock 2010; Timmermann et al. 2007).
9.3.9 Neurologic, Endocrine, and Cognitive Effects
The potential late effects of irradiation have led to past and present decisions about the indications for RT in the treatment of EPN and the need to question its use in some patients. Recovery of neurologic impairment after aggressive neurosurgery is not impeded by RT although whether the rate of improvement can be higher without the use of RT remains open to question (Merchant et al. 2010; Morris et al. 2009). Hearing loss is uncommon with the use of RT alone and can be prevented by using advanced RT methods (Hua et al. 2008). Given the relatively common PF location of EPN avoiding the hypothalamic pituitary axis in treatment planning has become easier, leading fewer cases of growth, thyroid, adrenal, and gonadotropin deficiency. Nevertheless, collateral irradiation of the hypothalamic pituitary axis, even with very low RT doses, can over many years result in the development of hormone deficiency (Merchant et al. 2011) and therefore, should be monitored.
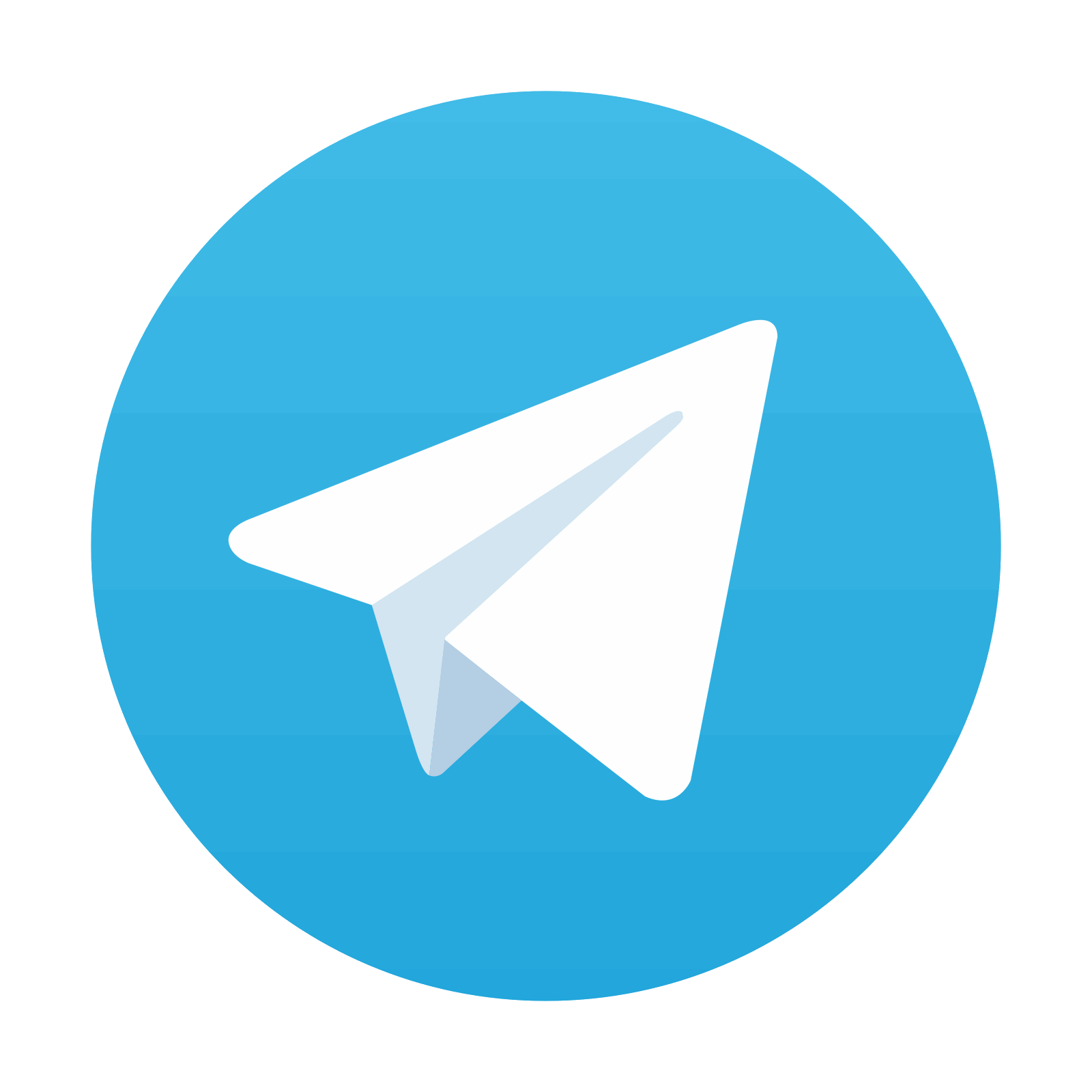
Stay updated, free articles. Join our Telegram channel

Full access? Get Clinical Tree
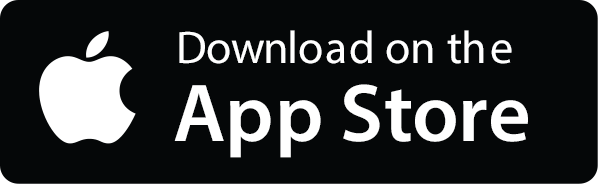
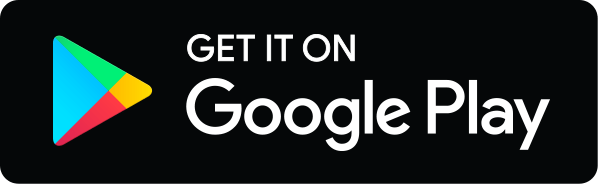