12 Klaas R Westerterp Maastricht University Man is a heterotrophic organism, deriving its energy from organic compounds. Energy is provided by the oxidation of nutrients, being the carbohydrates, proteins and fats consumed, called the macronutrients. A fourth source of food energy that can sometimes make up a significant part of energy intake is alcohol. The oxidation of nutrients provides the energy necessary to synthesise adenosine triphosphate (ATP), which is used for metabolic work. Less than 50% of the potential energy available from the oxidation of nutrients is actually conserved in the phosphoanhydride bonds of ATP; the rest is lost as heat. Additionally, when ATP molecules provide energy for metabolic work by hydrolysis, most of the energy will be transformed into heat as well. Thus, energy expenditure can be assessed by measuring heat loss or, indirectly, by measuring gas exchange in the oxidation of nutrients. The former method is named direct calorimetry, the latter indirect calorimetry. Man is a homeothermic organism, maintaining an almost constant body temperature over a wide range of environmental temperatures. The metabolic requirements of a homeotherm are mainly a function of the body’s surface area. The physiological explanation is that heat is lost via the body surface. The body surface is proportional to body mass to the power of 2/3. Thus, body mass is a determinant of metabolic requirement. Additional determinants are food intake, through the expenditure for food processing, and physical activity. Energy expenditure for maintenance, food processing and physical activity together largely explain the variation in daily energy expenditure within and between individuals. Assessment of daily energy expenditure is the indicated method for the assessment of the energy requirement. Nowadays, energy expenditure can be accurately assessed with a calorimetric technique under daily living conditions over intervals of one or more weeks. As such, it has become the reference for the evaluation of methods to measure energy intake. This chapter describes methods to measure energy expenditure, determinants of energy expenditure and the application of energy expenditure measurements for the evaluation of intake methods. The methods section includes techniques, methods to measure separate components of daily energy expenditure, and measuring substrate utilisation. The determinants section describes the subject characteristics involved, such as body composition, age and gender, and behavioural aspects including food intake and physical activity. Evaluation of intake methods is based on principles of energy requirement and techniques to assess energy homeostasis. The first measurements of energy expenditure, dating back to the early seventeenth century, were based on a balance technique. Sanctorius in 1614 demonstrated how a subject loses weight while seated in a chair suspended from a balance. He distinguished between sensible loss of weight in faeces and urine and insensible loss ascribed to insensible perspiration. Insensible perspiration was assumed to reflect energy expenditure. Now, we know that insensible loss is the sum of the weight of water vapour given off and the difference in weight of oxygen consumed and carbon dioxide produced for energy metabolism. The following calculation shows that insensible perspiration is more a reflection of water lost by evaporation than of energy expenditure. The calculation is performed for a man with an average daily energy expenditure of 12 MJ/day, completely covered by glucose oxidation. The body produces energy from glucose by oxidation: 1 mol glucose (C6H12O6) is oxidised with 6 mol oxygen (O2) to 6 mol carbon dioxide (CO2) and 6 mol water (H2O): In grams, 180 g glucose (1 mol) oxidise with 192 g oxygen (6 mol = 6 × 32) to produce 264 g carbon dioxide (6 mol = 6 × 44), 108 g water (6 mol = 6 × 18) and energy. The heat of combustion of glucose is 2.8 MJ/mol. Thus, the energy expenditure of 12 MJ/day is covered by the oxidation of about 4 mol glucose. The body weight change due to the difference between the weight of oxygen consumed and carbon dioxide produced is (4 × 192) – (4 × 264) = –288 grams/day. Water loss through breathing and evaporation via the skin is on average one-third to two-thirds of the average daily water turnover of 3 l/day, or 1000–2000 g/day depending on clothing, ambient temperature and humidity. Thus, water loss through evaporation makes up for the largest part and for a highly variable part of insensible perspiration. The next step in the measurement of energy expenditure was a calorimeter, measuring the heat released by an organism. It assumes that all energy provided by the oxidation of nutrients is eventually transformed into heat. Current techniques for the measurement of energy expenditure are based on the determination of oxygen consumed and carbon dioxide produced for energy metabolism. Measuring energy expenditure based on heat release is named direct calorimetry, to distinguish it from measurements based on gas exchange, named indirect calorimetry. Indirect calorimetry, including the simultaneous determination of oxygen consumption, carbon dioxide production and nitrogen loss in urine, can also be used to estimate the contribution of carbohydrate, protein and fat to energy production. There are several ways to measure the heat release of an organism to quantify energy expenditure. In Paris in 1780, Lavoisier placed a guinea pig in a wire cage surrounded by chunks of ice. As the ice melted from the animal’s body heat, the water collected below in a container, which could be weighed. The amount of melted water allowed calculation of the heat production, 334 J/g. The calorimeter was adiabatic in that the outer space around the ice cavity surrounding the cage was packed with snow to maintain a constant temperature around the inner shell, which was filled with ice. The days when these measurements could be made were limited by the mild winters in Paris at a time when ice machines had not yet been invented. Later developments were an airflow calorimeter and a water-flow calorimeter. An airflow calorimeter consists of a temperature-insulated ventilated space, for instance a room to house a subject. The temperature change of air flowing through the room multiplied by its mass flow rate and specific heat gives the rate of heat loss from the subject. The change in water vapour content of the air stream is measured to determine additional evaporative heat loss. A recent development of a water-flow calorimeter is a suit calorimeter. The suit calorimeter was developed from a device needed to cool astronauts while they are active outside their spacecraft. The subject is dressed in a close-fitting elastic undergarment, which carries a network of small plastic tubing over the entire body surface, except for the face, fingers and soles of the feet (Figure 12.1). Water circulated through the tubing carries heat from the skin, which is measured as the product of the mass flow of water and the change in temperature across the suit. Layers of insulating garments limit the exchange of heat with the environment. Evaporative heat loss is calculated from insensible perspiration; that is, body weight change corrected for intake and output of solids and liquids, and for the mass difference between estimated oxygen intake and carbon dioxide output. Figure 12.1 A subject in a suit calorimeter: (a) an elastic undergarment carrying a network of plastic tubing over the entire body and connected with a circulation pump (b) with insulating garments during a measurement walking on a treadmill. In indirect calorimetry the energy production is calculated from oxygen consumption, carbon dioxide production and urine-nitrogen loss. The basis of the calculation is the gaseous exchange and energy release from the metabolised carbohydrate, fat and protein. As already described, 1 mol glucose is oxidised with 6 mol oxygen to 6 mol carbon dioxide and 6 mol water and produces 2.8 MJ energy. A similar equation exists for fat oxidation. Protein oxidation results in carbon dioxide, water and compounds of nitrogen (e.g. urea and creatinine), where the latter is excreted in the urine. The resulting three equations with three unknowns can be solved for energy expenditure (E): Examples of equations for the calculation of energy production derived from these figures are the Weir equation and the Brouwer equation. Differences in the coefficients are caused by differences in assumptions on gaseous exchange and energy release from the metabolised carbohydrate, fat and protein. The contribution of measured urine-nitrogen loss to calculated energy production, the so-called protein correction, is only small. In the case of a normal protein oxidation of 10–15% of daily energy production, the protein correction for the calculation of E is smaller than 1%. Usually, urine-nitrogen is only measured when information on the contribution of carbohydrate, fat and protein to energy production is required. For calculating the energy production the protein correction is often neglected. Current techniques utilising indirect calorimetry for the measurement of energy expenditure in man are a face mask or ventilated hood; a respiration chamber; and the doubly labelled water method. A typical example of a ventilated-hood system is an open canopy. It is used to measure resting energy expenditure and energy expenditure for food processing or diet-induced energy expenditure. The subject lies with his or her head enclosed in a plastic canopy, sealed off by plastic straps around the neck (Figure 12.2). Air is sucked through the canopy with a pump and blown into a mixing chamber, where a sample is taken for analysis. Measurements taken are those of the airflow and of the oxygen and carbon dioxide concentrations of the air flowing in and out. The most common device to measure the airflow is a dry gas meter comparable to that used to measure heating gas consumption at home. The oxygen and carbon dioxide concentrations are commonly measured with a paramagnetic oxygen analyser and an infrared carbon dioxide analyser respectively. The airflow is adjusted to keep differences in oxygen and carbon dioxide concentrations between inlet and outlet within a range of 0.5 to 1.0%. For adults this means airflow rates around 50 l/min.
Energy Expenditure and Intake Methods
12.1 Introduction
12.2 Measuring energy expenditure
History
Direct calorimetry
Indirect calorimetry
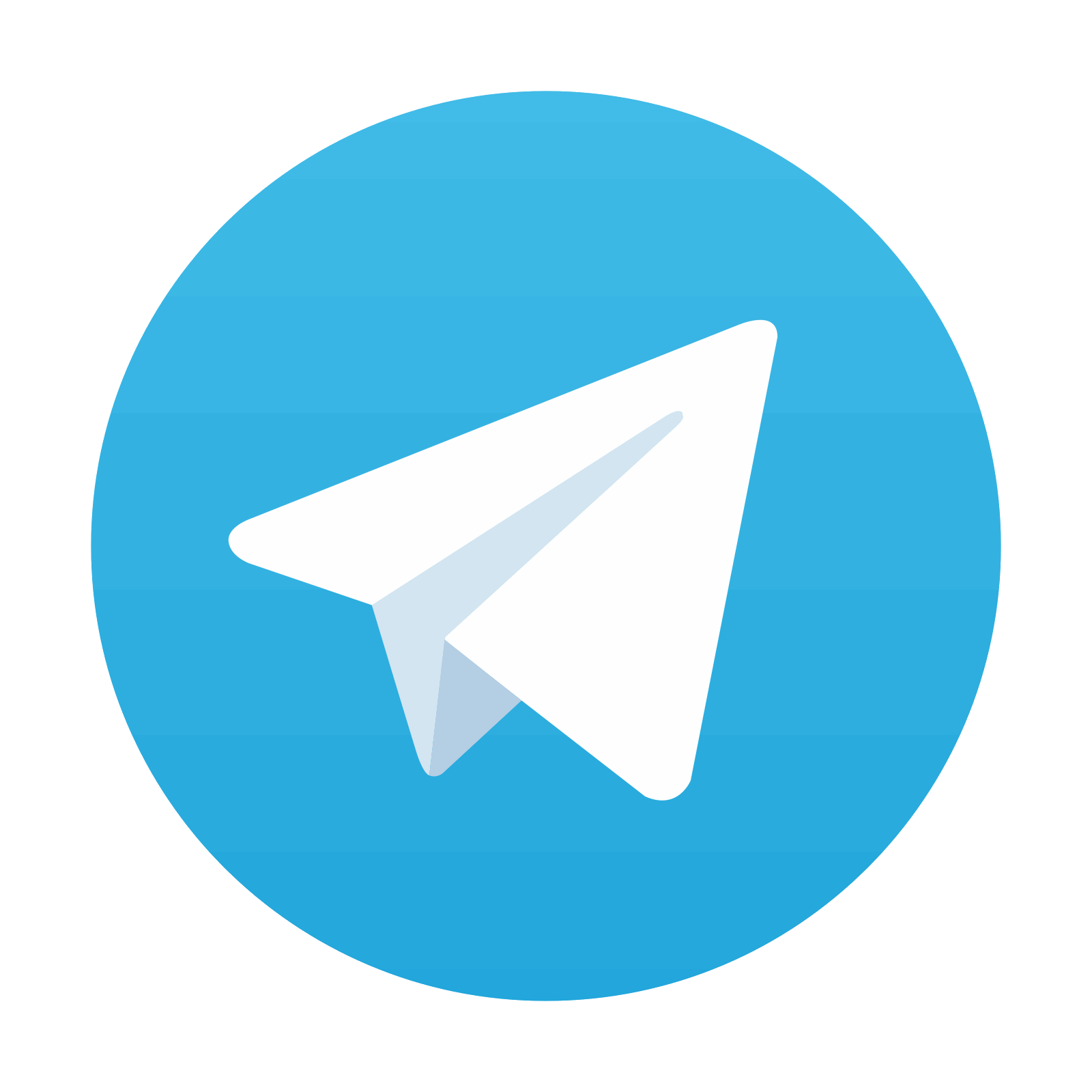
Stay updated, free articles. Join our Telegram channel

Full access? Get Clinical Tree
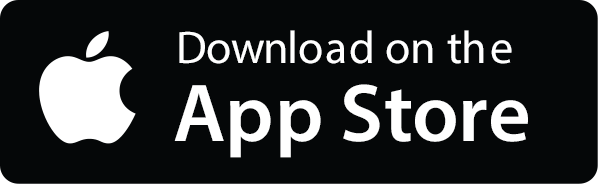
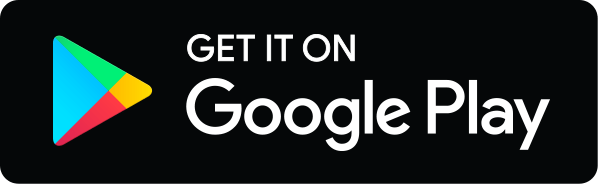