, Camilo Jimenez1, Naifa L. Busaidy1 and Mouhammed Amir Habra1
(1)
Department of Endocrine Neoplasia and Hormonal Disorders, The University of Texas MD Anderson Cancer Center, Houston, TX, USA
Chapter Overview
This chapter outlines the important and common late-onset, long-term endocrine disorders that occur as a consequence of cancer therapy in survivors: bone metabolism disorders, metabolic (i.e., glucose and lipid) disorders, thyroid neoplasia and dysfunction, hypothalamic-pituitary dysfunction, adrenal dysfunction, and gonadal system disorders. Strategies for evaluating and managing these effects are included.
Introduction
Advances in targeted, antineoplastic therapies have positively influenced progression-free survival and cure rates in cancer survivors. However, long-term undesirable effects are recognized and often require a multidisciplinary approach for evaluation and management. Adverse effects of cancer therapy on the endocrine system can span from a subtle laboratory abnormality with limited clinical significance to an end-organ effect with significant morbidity.
Bone Metabolism Disorders
One of the most prevalent long-term health effects in cancer survivors is bone loss or osteoporosis. Osteoporosis is a systemic skeletal disorder defined by low bone mineral density (BMD) and deterioration of the bone tissue microarchitecture, which may result in an increased propensity to fracture.
Several groups of cancer survivors are recognized to be at particularly high risk of developing osteoporosis. Women with breast cancer and men with prostate cancer treated with modalities to decrease or suppress estrogen and testosterone levels are at high risk for excessive bone loss. A third group at risk for bone loss is patients with lymphoma, myeloma, or leukemia, because of their exposure to osteoclast-activating cytokines secreted by neoplastic cells and to high-dose glucocorticoids included in treatment regimens. In most cases, it is undetermined whether bone loss in cancer survivors arises directly from the therapy itself, from the underlying disease process (including the impact of cachexia, malnutrition, and poor calcium and vitamin D intake), or from a combination of the two. Declining BMD, with complications of fractures and pain, becomes more important with increased duration of survival. Developing strategies to monitor and prevent significant bone loss and to treat osteoporosis and insufficiency fractures in cancer survivors has become a prominent focus of clinical research.
Therapies Affecting Gonadal Function
Patients with breast and prostate cancer may develop osteoporosis as a consequence of therapeutic hypogonadism, an important strategy in controlling hormone-dependent growth in these cancers. Unfortunately, estrogen and testosterone deficiencies lead to increased bone resorption and diminished bone density owing to abnormally increased bone production of interleukin-1, interleukin-6, and tumor necrosis factor-alpha, as well as reduced bone synthesis of transforming growth factor-beta 1 (Janssens et al. 2005). Additionally, an abnormal increase in the ratio of RANKL (receptor activator of nuclear factor kappa-B ligand) to osteoprotegerin leads to increased osteoclastogenesis. Because estrogen- and androgen-deprivation therapies severely decrease hormone levels, similar or even worse microarchitectural abnormalities, manifesting clinically as osteoporosis or fractures, are expected in survivors of breast or prostate cancer compared with non–cancer survivors who have hormonal deficiencies.
Bone loss in the spine is more rapid and severe within the first year of various treatments for breast cancer (2.6–7.7%) than with natural menopause (averaging 2% per year for 5–10 years). More importantly, the annual incidence of vertebral insufficiency fractures is higher in patients with early-stage breast cancer than in the general population. Results from the Women’s Health Initiative found that breast cancer survivors had a 15% higher rate of all fractures, regardless of the treatment they received, than women without any cancer history (Chen et al. 2005).
In patients with prostate cancer, androgen deprivation therapy (bilateral orchiectomy, leuprolide, or other gonadotropin-releasing hormone analogues), either alone or in combination with an antiandrogen therapy (e.g., flutamide, bicalutamide), causes profound hypogonadism characterized by loss of libido, muscle mass, and bone. Significant bone loss can occur in men within a year of castration or 6 months after initiating treatment with a gonadotropin-releasing hormone (GnRH) analogue. The annual incidence of osteoporotic fractures is higher in patients with prostate cancer treated with surgical or medical castration than in those who receive nonhormonal treatment or in healthy men (Melton et al. 2003). Fractures occur within 2 years of beginning androgen deprivation therapy and increase in frequency with the duration of the therapy. Importantly, skeletal fractures in patients with prostate cancer may be associated with decreased survival, independent of the pathologic stage of the cancer.
Selective Estrogen Receptor Modulators and Aromatase Inhibitors
In patients with breast cancer, selective estrogen receptor modulators (i.e., tamoxifen or raloxifene) have antagonistic effects in breast tissue, which supports the use of these agents as adjuvant therapy for patients at high risk for recurrence or as preventive therapy for healthy women at risk of developing breast cancer. However, both tamoxifen and raloxifene have antagonistic and agonistic effects on bone depending on the patient’s menopausal status. Premenopausal women receiving tamoxifen or raloxifene can experience loss in bone mass attributable to antagonism of endogenous estrogen in bone. In contrast, postmenopausal women, who already have extremely low levels of bioavailable estrogen, typically exhibit increased bone density because the estrogen-like effect of a selective estrogen receptor modulator is sufficient to positively affect bone density (Vehmanen et al. 2006).
Aromatase inhibitors (AIs; e.g., anastrozole, letrozole, exemestane) have become preferred for postmenopausal women with hormone receptor–positive breast cancer. By inhibiting the enzyme that mediates peripheral conversion of androgenic precursors (testosterone and androstenedione) of adrenal origin to estradiol and estrone, AIs can further suppress estrogen levels in postmenopausal women with breast cancer. Thus, AIs can lead to significant bone loss and increased fracture risk compared with placebo or tamoxifen (Baum et al. 2003; Howell 2006; Perez et al. 2006; Coleman et al. 2007). Interestingly, several studies revealed that patients with normal BMD at baseline did not become osteoporotic; in comparison, osteopenic patients experienced greater decreases in BMD after treatment with an AI (Perez et al. 2006; Coleman et al. 2007). Thus, surveillance and management of bone loss should be personalized to each patient on the basis of appropriate risk assessment.
Gonadotropin-Releasing Hormone Agonists
For premenopausal women, estrogen suppression is required for the treatment of hormone-sensitive breast cancer. This can be accomplished by bilateral oophorectomy, radiation-induced ovarian ablation, or administration of an agonist of GnRH or luteinizing hormone–releasing hormone (LHRH). Goserelin, an LHRH-agonist, in combination with tamoxifen is preferred over either treatment alone in premenopausal women with hormone-sensitive breast cancer. Consequently, BMD decreases substantially; however, BMD can recover partially within 1 year after cessation of treatment with goserelin.
In patients with prostate cancer, significant losses in BMD can occur in those treated with leuprolide or goserelin, in ranges higher than observed with normal male aging (Maillefert et al. 1999; Smith et al. 2001b). These losses in BMD are associated with abnormally increased bone turnover, in which bone destruction is predominant over bone formation.
Bilateral Orchiectomy
A significant reduction of BMD in both trabecular and cortical bone occurs in patients with prostate cancer 1–2 years after bilateral orchiectomy (Eriksson et al. 1995; Daniell 1997; Smith et al. 2001b). Fracture incidence is higher in patients treated with bilateral orchiectomy than in healthy, noncastrated cohorts (40% vs. 19%; Melton et al. 2003).
Chemotherapy-Induced Hypogonadism
Adjuvant systemic chemotherapy can induce ovarian failure in premenopausal women with early-stage breast cancer and can result in excessive bone loss in the first 12 months: 7% in the lumbar spine and 4.6% in the femoral neck. In addition, chemotherapy-induced menopause is a more important cause of osteoporosis than the direct effects of cytotoxic agents and glucocorticoids. Chemotherapy-induced amenorrhea is dependent on age (older than 40 years), dose, and medication type (e.g., cyclophosphamide, L-phenylalanine mustard, busulfan, chlorambucil, mitomycin-C). Patients with testicular cancer treated with cisplatin also develop hypogonadism.
Other Factors Contributing to Bone Loss and Fractures
Hematopoietic stem cell transplantation can lead to significant bone loss, most notably in the femoral neck, within the first 3–6 months after transplantation. In addition to increasing the risk of developing a hip fracture, reduced femoral neck BMD in patients undergoing transplantation is associated with avascular osteonecrosis.
Radiotherapy after surgical resection of breast, prostate, or gynecologic cancer can increase the risk of developing rib or pelvic insufficiency fractures. Exposure to high-dose corticosteroids used with systemic chemotherapy or to treat graft-versus-host disease exacerbates bone loss acutely. Other conditions, such as vitamin D deficiency or decreased physical activity during and after cancer therapy, may potentiate bone loss in this patient population.
Prevention and Management of Bone Loss in Cancer Patients
The National Comprehensive Cancer Center Network published guidelines on bone health surveillance in cancer patients. These guidelines incorporated fracture risk analysis by BMD, FRAX (an online calculation tool developed by the World Health Organization), and risk factors for bone loss, with guidance of pharmacologic therapy for low bone mass or osteoporosis (Gralow et al. 2009).
Prevention and treatment of bone loss in cancer patients has been best studied in breast cancer survivors receiving bisphosphonates for the prevention of bone loss related to chemotherapy, tamoxifen, and AIs (Gnant et al. 2008; Greenspan et al. 2008). Early initiation of bisphosphonate therapy may be beneficial in preventing or delaying bone loss. The clinical value of this practice will need to be validated, however, by demonstration of diminished fracture rates in longer follow-up studies of these patients. Very few trials have evaluated the effect of antiresorptive agents in patients with prostate cancer who undergo androgen deprivation therapy. For now, bisphosphonates remain the standard of care for men with osteoporosis.
Anabolic therapy with teriparatide, or parathyroid hormone 1-84, induces bone formation and prevents fractures in both men and women with severe osteoporosis. However, anabolic therapy induces production of growth factors that may theoretically induce tumor growth or tumor activation. Prior skeletal radiotherapy often precludes the use of teriparatide because of the perceived increased risk of developing osteosarcoma in these patients. These agents have not yet been investigated in patients with cancer; therefore, the risks and benefits of the use of anabolic agents should be considered carefully for cancer survivors (Hu et al. 2007).
Finally, denosumab, a fully human monoclonal antibody against RANKL to inhibit osteoclast activation, has been investigated in postmenopausal women with osteoporosis, cancer-related bone loss, and metastatic bone disease (Ellis et al. 2009; Smith et al. 2009; Stopeck et al. 2010; Boonen et al. 2011; Fizazi et al. 2011; Henry et al. 2011). Advantages of denosumab include the lack of nephrotoxicity and the reversibility of the suppressive effect on osteoclast activity.
Currently, no reports on the bone-protective effects of calcium and vitamin D in patients with cancer have been published. However, supplementation with vitamin D has been shown to reduce the risk for hip fractures in healthy ambulatory women. Evaluations of low bone mass are recommended to include an assessment of vitamin D status.
Osteomalacia and Rickets
Osteomalacia is a disorder of decreased bone mineralization of osteoid at sites of bone turnover, which can lead to bone pain, fracture, and difficulty walking or muscle weakness. In children, the abnormal mineralization and maturation of the growth plate at the epiphysis is called rickets. Vitamin D deficiency (related to poor nutritional intake, lack of sun exposure, or malabsorption) and renal wasting of phosphorus are common causes of osteomalacia, especially in cancer survivors. Other contributing factors include chronic kidney disease, exposure to aluminum in total parenteral nutrition, and systemic acidosis. Antineoplastic agents (e.g., ifosfamide, estramustine) can also cause or worsen osteomalacia. Treatment should include vitamin D replacement and correction of hypophosphatemia and hypocalcemia.
Metabolic Disorders
Glucose Metabolism Disorders
Glucocorticoids are used with many chemotherapy protocols and can have profound effects on glucose levels by increasing insulin resistance. Glucocorticoids can unmask preexisting prediabetic states by precipitating overt diabetes or make diabetes more difficult to control. The severity may range from asymptomatic hyperglycemia to nonketotic hyperosmolar coma. Most patients who are receiving glucocorticoids and have elevated glucose require insulin therapy to achieve blood glucose control, especially when given high-dose steroids.
Currently, there are no evidence-based, specific guidelines for the management of steroid-induced diabetes mellitus in patients with cancer. Long-acting and intermediate-acting insulin formulations are more effective at controlling glucose levels when they are combined with mealtime rapid-acting or short-acting insulin than with sliding-scale regimens that use short-acting insulin alone. Conflicting clinical study results concerning glargine and cancer have led to concerns that the mitogenic effect of insulin and insulin analogues, which cross-activate IGF-1 receptors, may promote malignancy. These concerns have drawn attention to the gap in knowledge about proper diabetic management strategies for cancer patients and survivors to maximize their survival (Eckardt et al. 2007; Hemkens et al. 2009; Home and Lagarenne 2009; Weinstein et al. 2009).
Temsirolimus, an inhibitor of mammalian target of rapamycin (mTOR), may cause secondary diabetes in 10–30% of patients with renal cell carcinoma. The mechanism by which temsirolimus leads to diabetes may be similar to that of tacrolimus, which decreases glucose-stimulated insulin release in the pancreatic islets.
L-asparaginase and pegylated asparaginase, used to treat hematologic malignancies, may cause hyperglycemia and occasionally diabetic ketoacidosis through unclear mechanisms (Gillette et al. 1972; Land et al. 1972). It has been postulated that inhibition of insulin, insulin receptor synthesis, or both may lead to a combined insulin deficiency and resistance syndrome. Pancreatitis, another known side effect, may contribute to hyperglycemia through islet cell destruction. Insulin therapy is frequently required to treat the hyperglycemia, which reverses upon discontinuation of treatment with L-asparaginase. Close monitoring for hypoglycemia is recommended after discontinuation of treatment with L-asparaginase. Long-term insulin therapy may not be needed in all cases of L-asparaginase-induced diabetes mellitus.
Streptozocin, used to treat malignant islet cell tumors and other neuroendocrine tumors, can lead to long-lasting impairment of pancreatic β-cells in the production and release of insulin; however, most of the effects of streptozocin are reversible upon discontinuation of treatment with the drug. Although the reported incidence of glucose intolerance varies from 6 to 60%, cases are often mild to moderate in severity.
Use of recombinant interferon-alpha (IFN-alpha-2a, -2b) to treat malignancies has been associated with the development of new-onset hyperglycemia, deterioration of glycemic control in diabetics, and diabetic ketoacidosis. Although the incidence of IFN-alpha–induced diabetes mellitus in patients with cancer is unclear, it arises in about 0.7% of patients treated for chronic active hepatitis C (Okanoue et al. 1996). The exact mechanism of IFN-alpha–induced diabetes is not well understood.
Lipid Disorders
Lipid disorders are seldom evaluated in the process of active anticancer therapy because patients are often encouraged to maintain a positive metabolic balance via liberal oral intake. Some lipid disorders may be short-lived without clear clinical consequences, but some may be of clinical importance and need to be detected and treated. In general, triglyceride levels higher than 1,000 mg/dL need to be treated urgently owing to serious potential complications, such as pancreatitis and visual impairment.
Hypertriglyceridemia
IFNs may induce hypertriglyceridemia (as high as 1,000 mg/dL) by increasing hepatic and peripheral fatty acid production and suppressing hepatic triglyceride lipase. In one case, a controlled diet and gemfibrozil had therapeutic effects despite continued treatment with IFN-alpha (Berruti et al. 1992).
All-trans retinoic acid (tretinoin) and other retinoic acid derivatives, which are used to treat several malignancies (e.g., head and neck cancer, acute promyelocytic leukemia), are well known to induce hypertriglyceridemia and hypercholesterolemia (Marsden 1986; Castaigne et al. 1990; Vahlquist 1991; Fujiwara et al. 1995; Kanamaru et al. 1995). Hypertriglyceridemia is the most common drug-related adverse effect (79%) observed with bexarotene, a synthetic retinoid X receptor–selective retinoid used to treat cutaneous T-cell lymphoma (Duvic et al. 2001a, b). Cerebrovascular accidents and pancreatitis have been described in association with retinoid-induced hypertriglyceridemia. Retinoid-induced hyperlipidemia has been successfully treated with fibrates or fish oil.
mTOR inhibitors frequently cause hyperlipidemia owing to impaired clearance of lipids from the bloodstream, but often this issue disappears when treatment with the drug is discontinued.
Hypercholesterolemia
Dose-related hypercholesterolemia has been described in patients with adrenocortical carcinoma treated with mitotane from inhibition of cholesterol oxidase (Vassilopoulou-Sellin and Samaan 1991). Patients with adrenocortical carcinoma usually have a poor prognosis, making mild to moderate elevation of cholesterol less clinically relevant. However, long-term survivors who continue to receive treatment with mitotane can experience early development of atherosclerotic disease. The benefits of treating mitotane-induced lipid abnormalities have not been established in long-term survivors.
Thyroid Neoplasia and Dysfunction
Thyroid Neoplasms
Ionizing radiation is the only well-established etiology of thyroid cancer, most commonly the papillary subtype, through induction of DNA damage and formation of chromosomal rearrangements. A dose-response relationship between radiation exposure and relative risk of thyroid cancer occurs with radiation doses of ≤5 Gy (Ron et al. 1995). Female sex, age younger than 15 years when exposed to radiation, and a 20- to 30-year period since radiation exposure (even to areas outside of the head and neck) are associated with increased risk for thyroid cancer. Incidence of local invasion, multicentric disease, and distant metastasis at presentation is higher among patients with radiation-induced thyroid cancer than among patients with sporadic thyroid cancer. Thyroid carcinoma is most evident in long-term survivors of Hodgkin disease and non-Hodgkin lymphoma.
Chemotherapy is not a proven risk factor for thyroid carcinoma despite rare case reports to the contrary. The administration of radioactive iodine for diagnostic purposes does not seem to increase the risk of developing thyroid carcinoma.
Hyperthyroidism
Radiation-induced hyperthyroidism has been described but is far less common than radiation-induced hypothyroidism, which has long-term consequences. Thyroiditis-induced thyrotoxicosis occurs within 2 years of radiotherapy in most cases; several months later, hypothyroidism occurs. Risk of developing Graves disease also increases following radiotherapy. Patients with lymphoma treated with radiotherapy constitute the largest population to develop Graves disease after radiotherapy. Patients treated with radiation for nasopharyngeal, breast, or laryngeal carcinoma may also develop Graves disease.
Cytokine therapy (e.g., IFN-alpha, interleukin-2) has also been reported to lead to thyroid disease. IFN-alpha, via autoantibody production, can lead to autoimmune thyroid disease, specifically primary hypothyroidism, transient thyrotoxicosis, or, more rarely, Graves disease. Thyroid scans showing increased homogeneous uptake in the presence of hyperthyroidism are highly suggestive of Graves disease and warrant antithyroid medications. Treatment with interleukin-2 alone causes transient hyperthyroidism followed by hypothyroidism in about 50% of patients through an unclear mechanism (Vialettes et al. 1993).
Hypothyroidism
Head and neck radiotherapy, an important etiology of thyroid dysfunction, can induce primary hypothyroidism when administered in doses higher than 25 Gy to the region near the thyroid gland. Most cases of primary hypothyroidism occur about 5 years after radiotherapy. Subclinical hypothyroidism (elevated thyroid-stimulating hormone levels with normal thyroxine levels) without overt hypothyroidism occurs more often when doses of less than 40 Gy are administered. The probability of developing hypothyroidism increases with increasing radiation doses, increasing duration of follow-up after treatment, combined radiation and surgical treatments, and failure to shield midline structures. Other more immediate risk factors for hypothyroidism include thyroid resection during laryngectomy and disruption of the vascular supply of the thyroid gland during head and neck surgery.
Various agents used in cancer management have been associated with primary hypothyroidism: IFN-alpha, interleukin-2, cisplatin, bleomycin, dactinomycin, vinblastine, and etoposide. Newer antineoplastic agents such as targeted therapies or immunotherapies are associated with a variety of thyroid abnormalities. Autoimmune thyroid disease has been observed with the use of monoclonal antibodies against CD52 for chronic lymphocytic leukemia (alemtuzumab) and CTLA-4 for melanoma (ipilimumab; Hamnvik et al. 2011).
Tyrosine kinase inhibitors (imatinib, sorafenib, sunitinib, and pazopanib) can lead to increased levothyroxine requirements in patients who have undergone thyroidectomy, suggesting that tyrosine kinase inhibitors may accelerate the clearance of levothyroxine (Dora et al. 2008). Additionally, it has been hypothesized that the inhibition of vascular endothelial growth factor by tyrosine kinase inhibitors may decrease vascular supply to the thyroid gland. Prospective studies of sorafenib estimated that the risk of thyroid dysfunction with sorafenib reached 68%; however, only 6% had clinical symptoms requiring thyroid hormone replacement (Miyake et al. 2009). Similarly, 36% of patients treated with sunitinib had persistently elevated levels of thyroid-stimulating hormone, suggestive of primary hypothyroidism; this was especially evident in those who had received long-term treatment with sunitinib (Desai et al. 2006). Some patients were reported to present with thyrotoxicosis preceding hypothyroidism, which supports the theory that sunitinib leads to destructive thyroiditis (Faris et al. 2007; Grossmann et al. 2008). Impaired iodine uptake and inhibition of peroxidase activity were also suggested as potential mechanisms to explain hypothyroidism (Mannavola et al. 2007; Wong et al. 2007).
Hypothalamic-Pituitary Dysfunction
It is generally believed that chemotherapy alone does not induce hypothalamic-pituitary dysfunction, although Rose et al. (2004) showed that hypothalamic dysfunction could occur in survivors of non–central nervous system tumors who received chemotherapy instead of radiotherapy. Malignancies in the vicinity of the hypothalamic-pituitary axis are often treated with surgery or radiotherapy. The hypothalamus is more sensitive than the pituitary gland to the effects of radiation. Hypothalamic-pituitary dysfunction resulting from radiotherapy in the cranial region may be delayed and can linger for many years. Reports have suggested that the number of pituitary deficiencies increases with the length of time since the radiotherapy. Surgery to remove malignancies such as craniopharyngioma in the hypothalamic region may also result in hypothalamic dysfunction.
Hypopituitarism
Hypopituitarism is not as common for survivors of adult nonpituitary brain tumors as it is for survivors of childhood nonpituitary brain tumors (Agha et al. 2005). IFN has been associated with hypopituitarism (Concha et al. 2003; Chan and Cockram 2004). Besides surgery, radiotherapy is often used to treat malignancies in the craniospinal region. Hypopituitarism, one of the most common complications after radiotherapy to the hypothalamus-pituitary axis, has been reported to occur in 41% of survivors of nonpituitary brain tumors (Agha et al. 2005). After adjuvant radiotherapy for pituitary macroadenoma, 36% of patients developed partial pituitary hormone deficiency and 61% had panhypopituitarism; 87% needed hormone replacement therapy to alleviate symptoms (Langsenlehner et al. 2007). The prevalence of hypopituitarism increases with the duration of follow-up.
Hypophysitis is a rare immune-related side effect that has been reported in 1–6% of patients with melanoma treated with ipilimumab. Hypophysitis leads to headaches, visual disturbances, and panhypopituitarism, and it is usually visible on magnetic resonance imaging as a pituitary enlargement with thickening of the stalk. Patients should initially receive high doses of glucocorticoids along with thyroid hormone replacement therapy. The necessary duration of replacement therapy with physiologic doses of hydrocortisone and levothyroxine could be very long, and permanent substitution therapy may be required (Dillard et al. 2010).
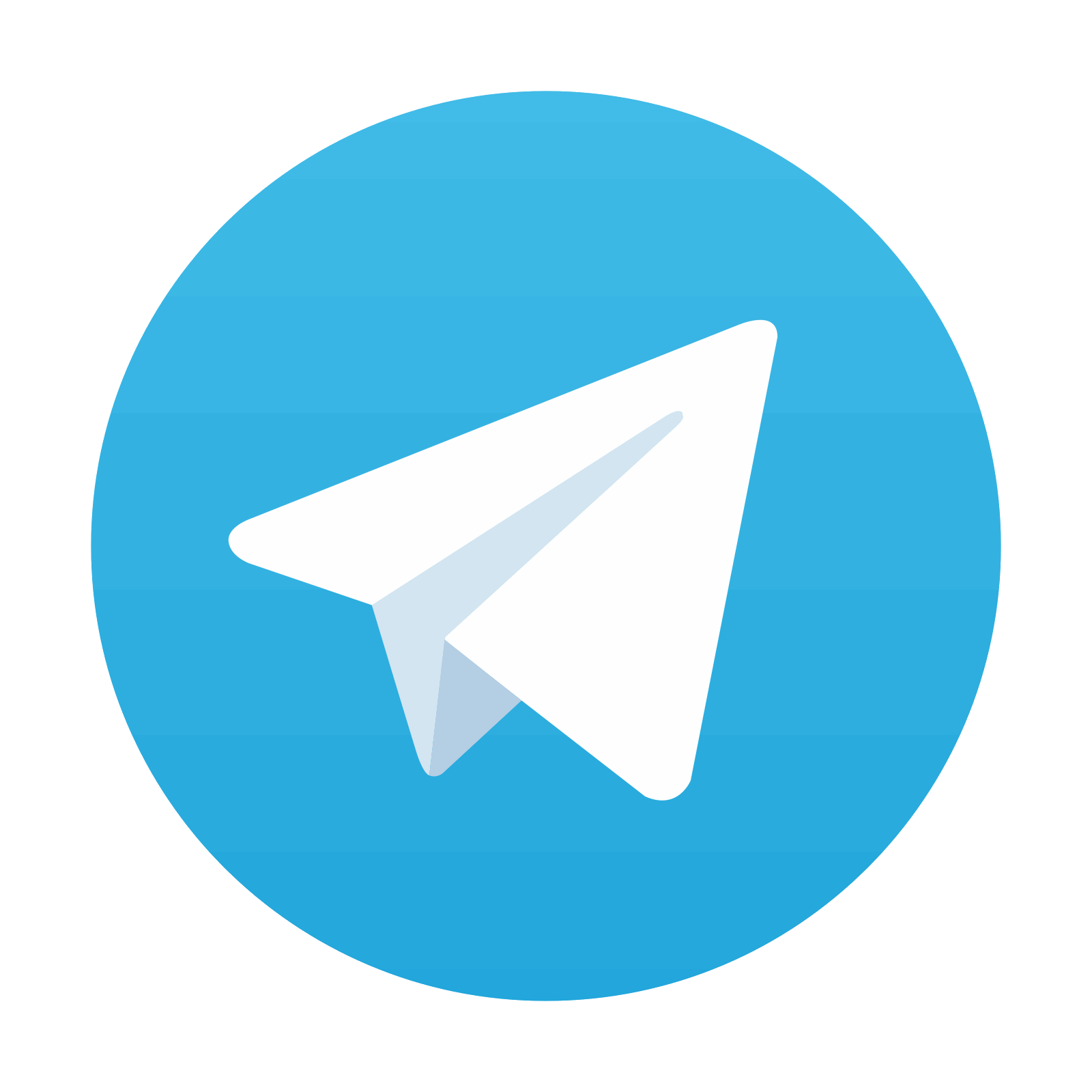
Stay updated, free articles. Join our Telegram channel

Full access? Get Clinical Tree
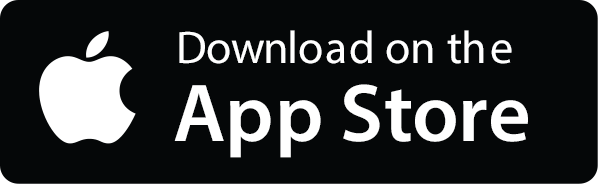
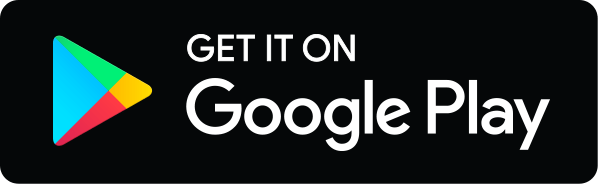