Abbreviations
- AADC Aromatic L-amino acid decarboxylase
- ACA Antibodies recognizing the adrenal cortex
- ADCC Antibody-dependent cell-mediated cytoxicity
- AICD Activation-induced cell death
- AIRE Autoimmune regulator gene
- APECED Autoimmune polyendocrinopathy-candidiasis-ectodermal dystrophy
- APS Autommune polyglandular syndrome
- BB Bio breeding
- BCR B-cell receptor
- cAMP Cyclic adenosine monophosphate
- CaSR Calcium-sensing receptor
- CD Cluster of differentiation
- CTLA Cytotoxic T lymphocyte antigen
- DPT Diabetes Prevention Trial
- FOXP3 Forkhead box P3
- GABA Gamma-aminobutyric acid
- GAD Glutamic acid decarboxylase
- HLA Human leukocyte antigen
- IA-2 Islet cell antigen-2 (tyrosine phosphatase)
- IFN Interferon
- IL Interleukin
- IPEX Immunodeficiency, polyendocrinopathy, and enteropathy, x-linked
- LFA Lymphocyte function-associated antigen
- MHC Major histocompatibility complex
- NALP1 NACHT leucine-rich-repeat protein 1
- NALP5 NACHT leucine-rich-repeat protein 5
- NK Natural killer (cells)
- NOD Nonobese diabetic (mice)
- SCA Steroid-producing cell antibodies
- SCID Spontaneous combined immunodeficiency
- TAP Transporter associated with antigen processing
- TBI Thyrotropin-binding inhibition
- TCR T-cell receptor
- TD Thymus-dependent
- Tg Thyroglobulin
- TI Thymus-independent
- TNF Tumor necrosis factor
- TPO Thyroperoxidase
- TSH Thyroid-stimulating hormone
- TSH-R Thyrotropin receptor
- TSI Thyroid-stimulating immunoglobulin
- VNTR Variable number of tandem repeats
Endocrine Autoimmunity: Introduction
Epidemiologic analysis of a large population reported that about 1 of 30 (3.2%) people in the United States (more than 8.5 million individuals) are currently affected by autoimmune diseases. Graves disease, type 1 diabetes, pernicious anemia, rheumatoid arthritis, Hashimoto thyroiditis, and vitiligo are the most prevalent such conditions, accounting for 93% of affected individuals. A more global approach at calculating prevalence led to a corrected estimate between 7.6% and 9.4% of the world’s population as affected by autoimmune diseases (2.5 in 30 people worldwide).
These autoimmune diseases have traditionally been looked upon as forming a spectrum. At one end are found organ-specific diseases with organ-specific targets. Hashimoto thyroiditis is an example in which a specific lesion affects the thyroid (lymphocytic infiltration, destruction of follicular cells) and autoantibodies are produced with absolute specificity for thyroid proteins. At the other end of the spectrum are the systemic autoimmune diseases, broadly belonging to the class of rheumatologic disorders. Systemic lupus erythematosus is an example of a disease characterized by widespread pathologic changes and a collection of autoantibodies to DNA and other nuclear constituents of all cells. Many organ-specific autoimmune diseases are autoimmune endocrinopathies. Furthermore, most endocrine glands are subject to autoimmune attack including the adrenals (autoimmune Addison disease), gonads (autoimmune oophoritis), pancreas (type 1 diabetes), pituitary (autoimmune hypophysitis), and thyroid (autoimmune thyroid disease) (Table 2–1).
Disease | Gland | Autoantigen | Autoantibody |
---|---|---|---|
Autoimmune (lymphocytic) hypophysitis Granulomatous hypophysitis | Pituitary | Pituitary cytosolic protein | Antipituitary |
Graves disease | Thyroid | TSH-R, TPO | TSI, TBII, anti-TPO |
Hashimoto thyroiditis | Thyroid | TPO, Tg | Anti-TPO, anti-Tg |
Autoimmune (idiopathic) hypoparathyroidism | Parathyroid | CaSR, NALP5 | Antiparathyroid |
Type 1 diabetes mellitus | Pancreas (β cells) | GAD65, IA-2, insulin | Anti-GAD, anti-IA-2 (ICA), antiinsulin |
Type B insulin resistance with acanthosis nigricans (rare) | Adipocytes, muscle cells | Insulin receptor | Insulin receptor blocking |
Autoimmune Addison disease (autoimmune adrenal failure) | Adrenal | 21-Hydroxylase 17α-Hydroxylase P450scc | Anti-21-hydroxylase (ACA) Anti-17α-hydroxylase and anti-P450scc (SCA) |
Autoimmune oophoritis (premature ovarian failure) | Ovaries | Not yet identified unequivocally, 17α-hydroxylase P450scc | Also SCA in association with adrenal insufficiency |
Autoimmune orchitis Male infertility (some forms) | Testes | Sperm | Antisperm |
By far the most common autoimmune endocrine diseases are those involving the thyroid and type 1 diabetes. When the target is the thyroid gland and the clinical manifestation is hypothyroidism (Hashimoto thyroiditis), the prevalence is about 1%. When the manifestation is hyperthyroidism (Graves disease), the prevalence is about 0.4%. Both thyroid autoimmune disorders affect women preferentially. When the targets of the autoimmune response are the β cells of the pancreas, the clinical presentation is type 1 diabetes. The prevalence of type 1 diabetes is close to that of Graves disease (0.2%-0.5%); however, it has no gender predilection.
Basic Immune Components and Mechanisms
The immune system is constantly confronted with a variety of molecules and recognizes them as either self or foreign. The adaptive immune system has evolved to recognize virtually any foreign molecule, either in existence or yet to come. The repertoire of immune recognition molecules randomly formed by gene rearrangements is not limited by the genetic information encoded in the genome (Figure 2–1). As a result, an enormously wide array of immune recognition molecules is acquired by the human immune system. By way of illustration, the theoretical diversity of T-cell receptors (T-cell recognition molecules) by random rearrangements reaches 1015. This mechanism of rearrangement also applies to B-cell recognition molecules (ie, immunoglobulins). The random mechanism of gene rearrangement, however, produces immune recognition molecules that react with self components. Potentially dangerous immune cells carrying self-reactive recognition molecules are eliminated (negatively selected) during development of T lymphocytes in the thymus and of B lymphocytes in the bone marrow. It appears that only immune cells which react with foreign antigen strongly and with self antigen very weakly are positively selected and comprise the peripheral immune cell repertoire. This selection mechanism of immune cells is termed “central tolerance.” Self-reactive immune cells that skip central tolerance and reach the periphery are managed by other control mechanisms against autoimmunity and are either eliminated, rendered unresponsive, or suppressed (“peripheral tolerance”). Failures in these mechanisms of immunologic regulation, as proposed by Mackay and Burnet in 1964, are central features of the concept of autoimmunity.
Figure 2–1
Rearrangement of the T-cell receptor (TCR) α and β genes to form a functionally diverse receptor. During T-cell development, the TCR α and β gene segments rearrange by somatic recombination so that one of the Vα segments pairs with a single Jα segment, and a Vβ segment pairs with a single Dβ and Jβ segment. The C (constant) segments are brought together with the rearranged segments by transcription and splicing to generate the functional mRNA that will be translated into the α and β protein chains that compose the TCR.
Immune Recognition and Response
T and B lymphocytes are the fundamental and predominant immune cells. T lymphocyte precursors (pre-T cells) originate in the bone marrow and migrate to the thymus, where they undergo maturation and differentiation. At early stages, they express several T-cell surface molecules but still have genomic (not rearranged) configuration of their T-cell receptors (TCRs). These pre-T cells, destined to become T cells with TCR α/β chains (T α/β cells), pass through a critical phase during which self-reactive T cells are deleted by negative selection (see T-Cell Tolerance, later in this chapter). Few pre-T cells will express other types of chains on their TCR (T γ/δ cells). T α/β cells differentiate into either mature CD4 or CD8 cells. These now mature lymphocytes migrate to T-cell areas of peripheral lymphoid organs and exert their function as helper (TH) or cytotoxic (TC) cells when activated.
B lymphocytes mature and differentiate in the bone marrow and then migrate to the B-cell areas of lymphoid organs. Influenced by factors derived from TH cells previously activated by professional antigen-presenting cells (APCs) such as macrophages, some B cells differentiate to become immunoglobulin M (IgM)-producing cells (plasma cells). Most of the other activated B cells that do not differentiate into plasma cells revert to the resting state to become memory B cells. When memory B cells are further activated, two events occur: isotype switching (immunoglobulin class switching) and hypermutation of the immunoglobulin-variable region to further increase diversity and specificity (affinity maturation).
Activation of B cells requires recognition of the antigen as a whole, while T cells require recognition of antigenic peptides bound to major histocompatibility complex (MHC) molecules on the surfaces of professional APCs. Therefore, T-cell recognition is said to be MHC restricted.
The human MHC (human leukocyte antigen; HLA) consists of a linked set of genes encoding major glycoproteins involved in antigen presentation (Figure 2–2). The complex locates to the short arm of chromosome 6 and divides into three separate regions: class I, class II, and class III genes. The class I “classic” region encodes HLA-A, HLA-B, and HLA-C loci; the nonclassic or class I-related region encodes HLA-E, HLA-F, and HLA-G loci and other immunity-related genes such as CD1. The class II region (HLA-D) encodes HLA-DP, HLA-DQ, and HLA-DR loci and other genes related to antigen processing, transport, and presentation, such as, transporter associated with antigen processing (TAP). The class III region encodes genes for tumor necrosis factors α and β (TNF-α and TNF-β); complement factors C2, C4, and B; and the steroidogenic enzyme 21-hydroxylase. MHC class I (classic) molecules are found on all somatic cells, whereas MHC class I nonclassic antigens are expressed only on some (eg, HLA-F on fetal liver, HLA-G on placental tissues). CD1 molecules are expressed on Langerhans cells, dendritic cells, macrophages, and B cells (all professional APCs). MHC class II molecules are exclusively expressed on these professional APCs. However, virtually all cells except mature erythrocytes can express MHC class II molecules under particular conditions (eg, stimulation with interferon-γ [IFN-γ]). As a general rule, MHC class I molecules present peptides derived from endogenous antigens that have access to cytosolic cell compartments (eg, virus) to CD8 TC cells. On the other hand, MHC class II molecules present peptides derived from antigens internalized by endocytosis into vesicular compartments (eg, bacteria) to CD4 TH cells. MHC class II molecules also bind peptides derived from many membrane-bound self antigens.
Figure 2–2
Gene organization of the human major histocompatibility complex or human leukocyte antigen (HLA) complex. Regions encoding the three classes of MHC proteins are on top. APTP denotes a cluster of genes within the class II region, encoding genes related to antigen processing, transport, and presentation. Class III region encodes genes unrelated to class I or class II not involved in antigen presentation (TNF-α and -β, complement factors C2, C4, B, and 21-hydroxylase and others).
APCs process and present antigen in order to activate T cells utilizing MHC-peptide presentation (Figure 2–3). T cells require at least two signals to become activated. The interaction of a TCR expressed on antigen-specific T cells and the antigenic peptide–MHC complex expressed on APCs provides the first signal. The second signal is delivered primarily by the interaction between costimulatory molecules CD80 (B7.1) and CD86 (B7.2) on APCs and CD28 on T cells. These two signals induce proliferation of T cells, production of interleukin-2 (IL-2), and expression of the antiapoptotic protein Bcl-xL. TH cells and TC cells are effector cells that require both signals in order to become activated. However, TC cells also need the “help” provided by TH cells. Until recently, it was thought that TH and TC cells needed to interact with the same APC simultaneously and that cytokines (such as IL-2) produced by the TH cell would then act on the TC cell to facilitate its response. New studies suggest that the interaction between another costimulatory molecule, CD40 ligand (CD154), present on T cells, and CD40, present on APCs, may provide an alternative explanation. It appears that TH cells–recognizing antigenic peptides presented by APCs deliver a signal through the CD154–CD40 complex that “licenses” APCs to directly stimulate TC cells (Figure 2–4). Thus, there is no need for simultaneous interactions of TH and TC cells while encountering the APC. CD154–CD40 interaction also enhances expression of CD80 and CD86 as well as secretion of cytokines (IL-1, -6, -8, -10, and -12 and TNF-α).
Figure 2–3
Antigen recognition by T cells. From top to bottom: A. Antigen uptake: Incorporation of antigen (via phagocytosis, pinocytosis, or FcR-mediated endocytosis of immune complex). B. Antigen presentation: APCs deliver an antigen-specific signal through the MHC-peptide–TCR interaction on T cells (MHC I coupled to CD8 interacts with TC cells, MHC II coupled to CD4 interacts with TH cells). C. T-cell activation: The required second signal is provided via CD80/86 (B7.1; B7.2)–CD28 that induces the expression of CD154 (CD40 L) first and CD152 (CTLA-4) later on. Binding of CD154 on T cells with CD40 on APCs enhances expression of CD80/86. The APC–CD80/86 increased expression and consequent binding of CD28 on T cells perpetuates the activation and proliferation of these effector cells (downstream events). D. T-cell inactivation: CD152 (expressed 48-72 hours after T-cell activation) will preferentially bind to CD80/86 on APCs because of its higher affinity, displacing CD28 and in turn suppressing T-cell activity.
Figure 2–4
Licensed APCs directly activate TC cells. According to the traditional model, TH cells and TC cells recognize antigen on the same APC. The APC-activated TH cell produces IL-2, which contributes to the activation of TC cells while in simultaneous interaction with the same APC. According to the proposed new model (A), APCs are licensed to activate TC cells by TH or other stimuli (lipopolysaccharides, IFN-γ, viruses). APCs first interact with TH cells. The association of CD154 (CD40 L) on the TH cell and CD40 on the APC allows (or licenses) the latter to activate TC cells directly (B). Thus, once licensed, APCs are capable of activating cytotoxic T cells without the need of simultaneous interaction with TH cells.
Yet another molecule on T cells, the CD28 homolog cytotoxic T lymphocyte antigen 4 (CTLA-4 or CD152), functions to suppress T-cell responses (see Figure 2–3). CD152 is expressed at low to undetectable levels on resting T cells. It is upregulated by the ligation of CD28 on T cells with CD80/86 on APCs, or by IL-2. CD152 and CD28 on T cells share the same counterreceptors, namely, CD80/86 on APCs. However, CD152 has a 20-fold higher affinity than CD28 for their ligands.
The integration of all these interactions may be as follows (see Figure 2–3): After processing antigen, APCs deliver an antigen-specific first signal through the MHC-peptide–TCR interaction on T cells. A second signal is provided by a costimulatory interaction of the CD80/86–CD28 complex that induces the expression of CD154 first and then CD152. Binding of CD154 on TH cells with CD40 on APCs enhances expression of CD80/86 and licenses APCs for direct activation of TC cells. Other inflammatory cytokines as well as lipopolysaccharides and viruses may do the same. The increased expression of APC–CD80/86 and consequent binding of CD28 on T cells then perpetuates the activation and proliferation of these effector cells. However, the expression of CD152 48 to 72 hours after T-cell activation leads to the preferential binding of this molecule to CD80/86 on APCs because of its higher affinity for CD80/86. This may displace CD28 from CD80/86 and, in turn, suppress T-cell activity. This sequence of complex events is probably simplistic relative to what nature has to offer. A new B7 family of receptors has been reported: some with positive costimulatory capacity, many with a role in downregulating immune responses, yet others with dual functions. For some of these pathways such as B7H3/H4 (expressed in APCs with unknown counterreceptor on T cells), very little is known, but even for those pathways that have been extensively studied, such as the CD80/86:CD28/CTLA-4 (mentioned above); B7h:ICOS (inducible costimulator) and PD-L1/PD-L2:PD1, new insights are still being generated. The intricacies of controlling T-cell activation are enhanced by the complexity of the costimulatory pathways, such that there are multiple possible receptor–ligand interactions.
Activation and differentiation of B cells often require two signals also. Naive B cells are triggered by antigen but may also require accessory signals that come from activated TH cells. Some antigens can directly activate naive B cells without the need for TH cells (eg, lipopolysaccharides from gram-negative bacteria or polymeric protein structures). The former type of B-cell activation (MHC class II-restricted T cell help) is called thymus-dependent (TD). The latter type is called thymus-independent (TI). TH cells also control isotype switching and initiate somatic hypermutation of antibody variable-region genes (see Tolerance, later). Interaction between CD154 on TH cells and CD40 on B cells and the cytokines produced by TH cells are essential for isotype switching and formation of germinal centers in peripheral lymphoid organs. The immunoglobulin isotype switching is critical for the generation of functional diversity of a humoral immune response. Somatic hypermutation (point mutations of the variable-region genes of immunoglobulins during the course of an immune response) is necessary for the affinity maturation of antibodies.
Overall, the immune response is a combination of effector mechanisms that function to eliminate pathogenic organisms. These effector mechanisms include, as innate immunity, phagocytosis (by macrophages, neutrophils, monocytes, and dendritic cells) and cytotoxicity (by natural killer [NK] cells); and as adaptive immunity, antibody-dependent complement-mediated cytotoxicity, antibody-dependent cell-mediated cytotoxicity (ADCC), cytotoxicity by T γ/δ cells that recognize heat shock proteins on target cells, and cytotoxicity by CD8 or CD4 TC cells. CD8 and CD4 TC cells are activated by the described recognition of specific antigenic peptides bound to class I (for CD8), class II (for CD4) MHC molecules on the APCs and classically by IL-2 from nearby activated CD4 TH cells. These cells kill the target by either secreting cytotoxins (perforin, granzyme) or by inducing apoptosis through the Fas–FasL (Fas ligand) interaction (killer cells carrying FasL molecules activate programmed cell death in target cells expressing Fas molecules). FasL or CD95L is a type II transmembrane protein that belongs to the TNF family. The binding of FasL with its receptor induces apoptosis. FasL–Fas receptor interactions play an important role in the regulation of the immune system.
The specificity of the immune response is crucial if self-reactivity is to be avoided. In order to ensure that lymphocyte responses and the downstream effector mechanisms they control are directed exclusively against foreign antigens and not against “self” components, a number of safety-check barriers must be negotiated before autoreactive lymphocytes can differentiate and proliferate.
Tolerance
T cells developing in the thymus (pre-T cells) are destined to become T α/β cells through rearrangement of the TCR β gene initially, followed by the TCR α gene (Figure 2–5). If unproductive rearrangements of TCR genes occur (nonfunctional TCR α or β proteins), apoptosis of these pre-T cells follows (Figure 2–5A). If functional rearrangements of TCR α and β proteins occur, cells express TCR α/β dimer and CD3 molecules at low levels on the cell surface. TCR-rearranged cells proliferate 100-fold. Positive and negative selection occurs based on the ability of the rearranged TCR α/β to recognize antigenic peptides in association with self-MHC molecules on thymic epithelial and dendritic cells. Negative selection (clonal deletion) appears to take place in the thymus medulla, where pre-T cells–bearing TCRs specific for self peptides bound to self-MHC molecules are deleted. At least 97% of developing T cells undergo apoptosis within the thymus (central tolerance). Positively selected pre-T cells increase expression of TCR α/β, express either CD4 or CD8, and become mature T cells. These mature T cells exit the thymus and go to the periphery. CD4 T cells are activated in the periphery in an MHC class II-restricted fashion, while CD8 T cells are activated in an MHC class I-restricted fashion.
Figure 2–5
A. Central T-cell tolerance: Mechanisms of central tolerance (at the thymus level) are depicted. From top to bottom, pre-T cells first rearrange their TCR. Unproductive (nonfunctional) rearrangements lead to apoptosis, while productive ones engage pre-T cells in self-antigen recognition. Clonal deletion indicates elimination of cells based on their high or no avidity for self antigen (apoptosis). Surviving low-avidity cells reach the periphery as mature CD4 and CD8 cells. B. Peripheral T-cell tolerance: May be accomplished through any of the five depicted mechanisms. 1. Clonal deletion: After encountering self antigen in the context of self-MHC molecules and simultaneous delivery of a second signal (CD80/86–CD28) by APCs (top left), autoreactive T cells become activated. These activated T cells express Fas molecules on their surface but are resistant to Fas ligand (FasL)-mediated apoptosis because of the simultaneous expression of Bcl-xL (not shown) induced by CD28 ligation during activation. Several days after activation, when Bcl-xL presence has declined, CD4 cells become susceptible to FasL-mediated apoptosis. Natural killer cells (NK-T) may then accomplish the task of eliminating these autoreactive T cells. 2. Anergy: Anergy may be induced via CD80/86–CD152 interaction 48 to 72 hours following activation or may result from the lack of a second costimulatory signal from APCs presenting self antigen (nonprofessional APCs). 3. Active suppression: Active suppression is thought to occur when nonhematopoietic cells (stimulated by IFN-γ) present antigen in an MHC class II–restricted fashion to CD4 T-suppressor cells (TS, also known as CD4 + CD25 + FOXP3 + regulatory T cells, T regs). Before becoming unresponsive, these cells may induce specific CD8 TS cells. In turn, these CD8 TS cells may suppress antigen-specific autoreactive T cells. 4. Ignorance (top right): Some autoreactive T cells may never encounter self antigen because it may be sequestered from the immune system. Although they may persist in the circulation, they never become activated. 5. Immune deviation: Under specific circumstances, noninflammatory TH2 responses could suppress inflammatory (autoreactive) TH1 responses (see text).
A differential avidity model in which the fate of T cells is determined by the intrinsic affinity of TCRs for their ligands has been advanced to explain the paradox between positive and negative selection. According to this model, T cells with high avidity for MHC-self peptide complexes would be eliminated (negative selection), whereas T cells with low avidity to MHC-self peptide complexes would be positively selected. If the avidity is close to zero, T cells would not be selected (for lack of effective signal to survive). The biochemical factor or factors that signal survival (low avidity of TCR binding) versus apoptosis (triggered by high avidity interactions) have yet to be found.
Costimulatory interactions between CD28 and CD80/86 and between CD154, CD40, and adhesion molecules, such as lymphocyte function-associated antigen-1 (LFA-1), are also involved in preferential deletion of self-reactive T cells in the medullary region of the thymus. It is known that negative selection is not 100% effective and that some potentially autoreactive T cells do escape to the periphery. Not all self peptides would be presented to pre-T cells during their development in the thymus. Self peptides derived from secluded proteins (ie, intracytoplasmic enzymes) only timely expressed after rigid regulatory control (ie, puberty) in endocrine glands are believed to be a likely source. Therefore, the peripheral immune system must maintain tolerance through complementary control mechanisms.
“Peripheral tolerance” (Figure 2–5B) may be maintained by the induction of unresponsiveness to self antigen (anergy) or by the induction of regulatory T cells (T regs), such as suppressor T cells (active suppression). Peripheral clonal deletion (apoptosis) of autoreactive T cells that have escaped from the thymus may play an important role in limiting rapidly expanding responses, but there are many examples where autoreactive T cells persist. Some autoreactive T cells may never encounter the self antigen because it may be sequestered from the immune system (ignorance). Lastly, immune deviation, whereby noninflammatory TH2 responses suppress an autoreactive inflammatory TH1 response, inducing peripheral tolerance, deserves further discussion. TH1 cells, which regulate cell-mediated responses, secrete IFN-γ and small amounts of IL-4. In contrast, TH2 cells, which provide help for antibody production, secrete abundant IL-4 and little IFN-γ. A prevailing concept in human autoimmunity is that TH1 responses are believed to dominate. It has been shown in animal models that induction of TH2 responses ameliorates TH1 responses. Hence, unbalanced TH1 immune deviation may lead to a breakage of peripheral tolerance. However, evidence to the contrary exists in some endocrinopathies. (See autoimmune response in the section on Autoimmune Aspects of Thyroid Disease, later in the chapter.)
Clonal deletion and anergy occur through apoptosis at the site of activation or after passage through the liver. High antigen dose and chronic stimulation induce peripheral elimination of both CD4 and CD8 T cells. Activated T cells express Fas molecules on their surfaces but are resistant to FasL-mediated apoptosis because of the simultaneous expression of Bcl-xL (apoptosis-resistance molecules), induced by CD28 ligation during activation (see Immune Recognition and Response, earlier in the chapter). Several days after activation, when Bcl-xL has declined, CD4 cells become susceptible to Fas-mediated apoptosis (activation-induced cell death; AICD). A similar mechanism via p75 tumor necrosis factor (TNF) receptor has been shown for CD8 cells. Therefore, autoreactive T cells might be deleted by apoptosis induced by chronic stimulation with self antigens, present abundantly in the periphery. However, autoreactive T cells specific for very rare self antigens may be difficult to eliminate.
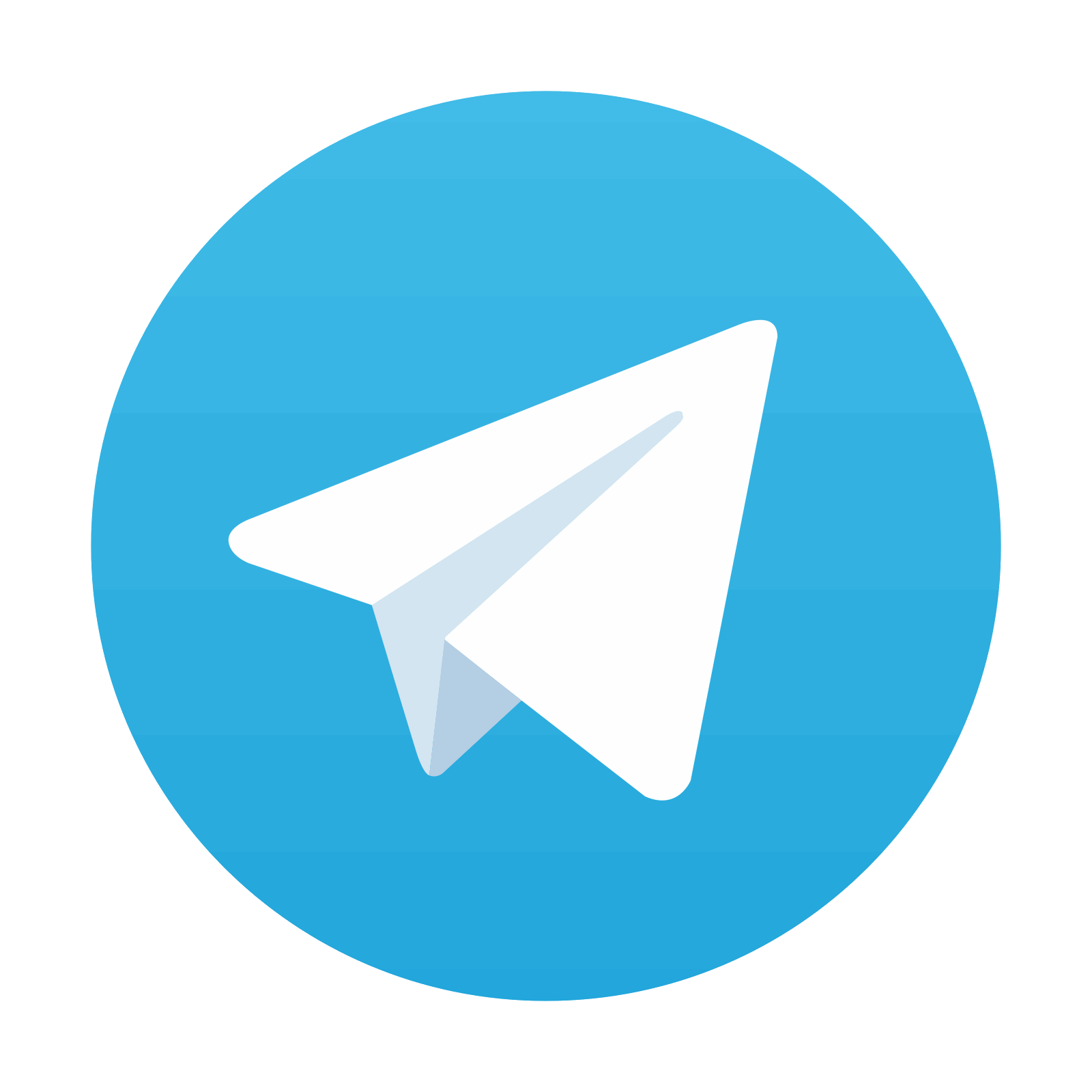
Stay updated, free articles. Join our Telegram channel

Full access? Get Clinical Tree
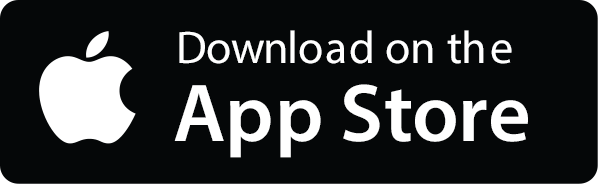
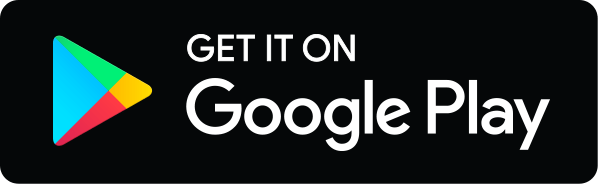