7
ELECTRON TREATMENT
Question 2
What is the practical range Rp for electrons?
Question 3
What effect does changing the source-to-skin distance (SSD) have on the electron depth dose curve?
Question 4
What is the field size dependence of R90 and Rp?
They lose energy through collisional interactions (ie, ionization and excitation) and radiative interactions (bremsstrahlung). In therapy, radiative losses only become significant at higher energies and high-Z materials.
Answer 2
The practical range is the depth of electron penetration. It is the point at which the tangent to the descending portion of the depth dose curve intersects with the backward extrapolation of the bremsstrahlung tail. Practical range in cm is approximately ½ E(MeV).
Answer 3
The effect is fairly minimal due to the shallowness of the penetration of the electron beam. However, for higher energies the R90 can be shifted distally a few mm as SSD increases.
Answer 4
When the field size is above a threshold value, there is no depth dose variation. Below this threshold (which varies with beam energy), there can be significant variation. The depth of R90 or range of 90% dose is shifted increasingly toward the surface as field size decreases. Rp does not change with field size as it reflects the energy of the beam only.
Question 6
What are some cases where skin collimation would be preferable to using a cutout?
Question 7
What is the rough approximation for the useful depth for an electron beam as it relates to its energy?
Question 8
An electron beam will be used to treat a tumor whose most distal depth is 4.8 cm. Assume there are no critical structures beyond the tumor. What energy should be used?
The surface dose increases with beam energy, from approximately 75% to 95%. Skin sparing is minimal with electrons, and almost nonexistent at higher energies. This is in contrast to photons where surface dose decreases with increasing beam energy.
Answer 6
The primary benefit of skin collimation is a much sharper penumbra compared with a cutout. This is useful for:
• Small field treatments
• Providing maximal protection to adjacent critical structures
• Reducing penumbra beneath a bolus
• Reducing penumbra beneath an extended air gap
• Reducing penumbra in electron arc therapy
Answer 7
The useful depth in cm is the approximate point where the 80% to 90% isodose line occurs:
D80(cm) ~ 1/3 E(MeV) or D90(cm) ~ E(MeV)/3.3. Note that E/4 has also been used to approximate D90. It is important to check percent depth dose curves (PDDs) for specific field size to choose the right energy for depth of treatment required.
Answer 8
Typically, the tumor should be covered by the 90% isodose line. A rule of thumb for determining the energy to use is
Ep,0 ~ 3.3 × R90(cm)
So in the example the energy selected would be 3.3 × 4.8 = 15.8, so a 16 MeV beam should be selected. To cover with the 80% isodose line, the rule of thumb is
Ep,0 ~ 3 × R80(cm)
and so the energy would be 3 × 4.8 = 14.4 or 15 MeV.
Where does the X-ray contamination of an electron beam come from, and how does it vary with energy?
Question 10
Why is bolus used in electron therapy and what kind of material is bolus made from?
Question 11
How would the depth dose for a rectangular field of width L × W be determined, if the depth doses for square fields L × L and W × W are known?
Question 12
Electron beams are ideally treated en face, or perpendicular to a flat patient surface. What is the effect of treating on a sloped, or oblique, skin surface?
The X-ray contamination of an electron beam results from bremsstrahlung interactions within the linac head (scattering foils, ion chambers, collimator jaws, etc.) and in patient tissues. Contamination increases with increasing energy, typical values are: 0.5% to 1% for 6 to 12 MeV, 1% to 2% for 12 to 15 MeV, and 2% to 5% for 15 to 20 MeV.
Answer 10
Bolus is used to: (1) reduce surface irregularities, (2) limit the penetration of the electron beam in certain places, and (3) increase the surface dose. Bolus is made of a tissue equivalent Z material. Commonly used bolus materials include wax, paraffin gauze, superflab, and other pliable plastics.
Answer 11
At a given depth d,
Answer 12
Treating on an oblique surface creates: increased surface dose, a higher maximum dose, a shift in R90 toward the surface, and an increase in Rp. These changes are most significant at angles of obliquity >30°. Beam obliquity increases side scatter at the point of maximum dose, increases the depth of penetration of the beam, and moves Dmax deeper into the patient.
What is the effect of treating through an air cavity? What should be done to mitigate this effect when treating through nasal passages?
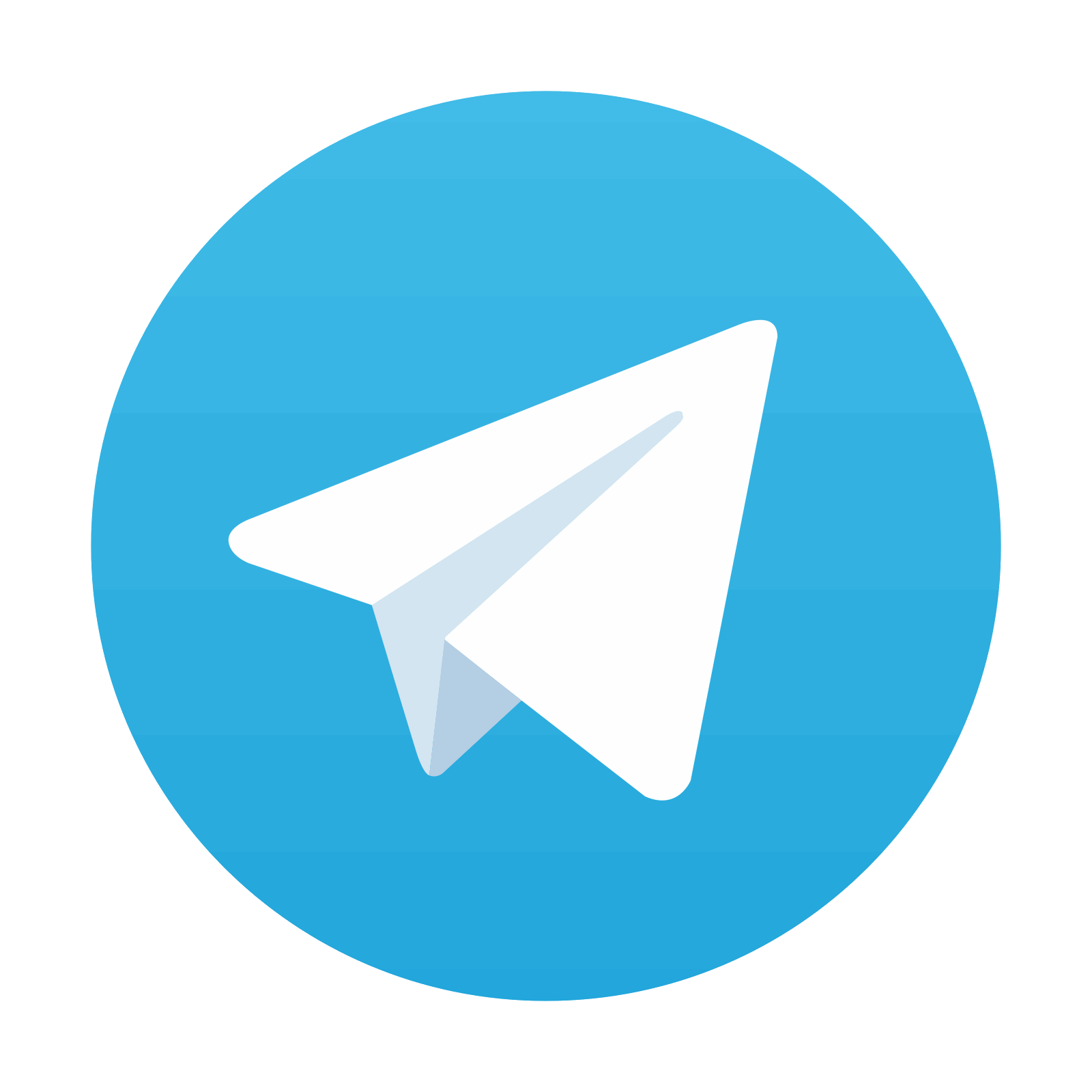
Stay updated, free articles. Join our Telegram channel

Full access? Get Clinical Tree
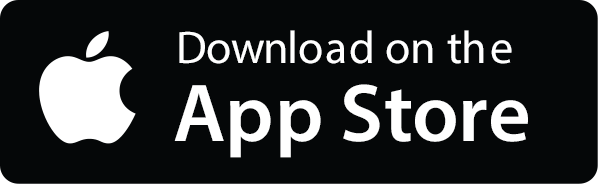
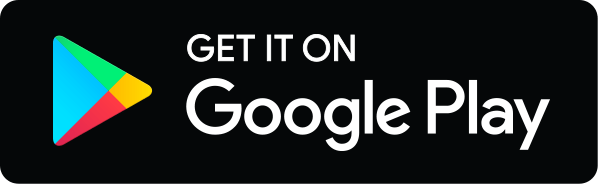