© Springer Science+Business Media New York 2016
Marina Kurian, Bruce M. Wolfe and Sayeed Ikramuddin (eds.)Metabolic Syndrome and Diabetes10.1007/978-1-4939-3220-7_1010. Effect of Bariatric Surgery on Insulin Secretion
(1)
Columbia University Medical Center, New York, NY, USA
(2)
Division of Endocrinology, Department of Medicine, Columbia University Medical Center, 650 West 168th Street, Black Building, Room 905, New York, NY 10032, USA
Keywords
ObesityType 2 DMInsulin resistanceInsulin secretionGlucose toleranceGlycemic controlBariatric surgery10.1 Introduction
Increase in obesity has led to higher prevalence of type 2 DM (T2DM), a chronic and progressive disease marked by insulin resistance and the eventual loss of insulin secretion. While weight loss improves glucose tolerance in obese patients with T2DM, conventional medical management often fails to achieve sustained weight loss and glycemic control in severely obese patients [1–3]. Multiple studies have documented the efficacy of bariatric surgery in attaining substantial weight loss as well as long-term improvement in diabetes and its associated comorbidities [4–10].
The effect of bariatric surgery on insulin sensitivity and secretion differs depending on the type of surgery, with the greatest effect observed following malabsorptive procedures [4, 5, 11]. Metabolic improvement after restrictive procedures such as gastric banding (LAGB) appears to be driven mostly by weight loss [12]. Greater insulin sensitivity and insulin secretion after malabsorptive procedures such as Roux-en-Y gastric bypass (RYGB) and biliopancreatic diversion (BPD) are driven not only by greater weight loss but also by anatomical changes that alter gut hormone secretion and nutrient transport. Sleeve gastrectomy (SG), initially thought to be mostly restrictive, also alters gut hormone secretion and leads to improvements in insulin sensitivity more comparable to RYGB than LAGB [13, 14].
The mechanisms by which bariatric surgery improves glucose homeostasis in obese patients with T2DM have yet to be fully elucidated, and likely encompass both weight loss dependent and weight independent mechanisms. In the immediate postoperative period, before notable weight loss has even occurred, there is a rapid decrease in fasting insulin and fasting glucose that reflects increased hepatic insulin sensitivity [15, 16]. Following significant weight loss several months after surgery, peripheral insulin sensitivity also improves, in parallel with the amount of weight loss. However, RYGB and SG are also associated with increased β-cell function and altered secretion of the incretin, glucagon-like peptide-1 (GLP-1), which occurs early and persists postoperatively.
This chapter discusses altered insulin sensitivity and insulin secretion after bariatric surgery, focusing on the factors contributing to increased insulin secretion particularly after RYGB.
10.2 Insulin Sensitivity
In obese diabetic patients, weight loss is accompanied by a corresponding increase in insulin sensitivity and increase in β-cell activity [17, 18]. It is also well established that significant caloric restriction improves glucose tolerance in diabetic patients [19]. Altered insulin sensitivity after bariatric surgery reflects the impact of both caloric restriction and weight loss.
Profound improvements in fasting glucose concentration and insulin action have been noted early after bariatric surgery, often before significant weight loss has even occurred [20–22]. Caloric restriction, which occurs immediately after all surgical interventions, likely plays an important role in the favorable metabolic changes observed, particularly in the early postoperative period [19, 22]. For example, after losing an equivalent amount of weight over about 3 weeks through RYGB or a 500 kcal per day diet, obese diabetic patients exhibited similar improvements in insulin sensitivity, acute insulin response, and β-cell function as assessed by an intravenous glucose challenge [23]. This early improvement reflects mostly improved hepatic insulin sensitivity from caloric restriction, as the profound negative calorie balance leads to decreased liver fat and increased insulin sensitivity [24], reduced basal glucose production, and increased liver clearance of insulin [25, 26].
Subsequent change in peripheral insulin sensitivity varies by surgical procedure. After LAGB, further improvement in peripheral insulin sensitivity occurs more gradually and is in proportion to the amount of weight loss [12]. In contrast, RYGB leads to profound changes in insulin sensitivity and insulin action much more rapidly [27–32]. Moreover, several studies have noted greater improvement in T2DM and greater increase in insulin sensitivity after RYGB over time, even after equivalent weight loss by dietary or restrictive interventions, suggesting weight-independent mechanisms [27, 28, 33]; however, these studies did not necessarily control for equivalent daily caloric intake. Others observe that the increased insulin sensitivity after RYGB occurs in proportion to weight loss, which is greater after malabsorptive surgery [34, 35].
Improved peripheral insulin sensitivity is greater after BPD than after other procedures and occurs as early as 2 weeks postoperatively [6, 36, 37]. As noted by Mari et al., BPD increases insulin sensitivity regardless of the baseline glucose tolerance, and leads to a significant decrease in insulin hypersecretion. Insulin sensitivity after BPD has even been observed to exceed that of obese patients with normal glucose tolerance [37]. Neither weight loss nor GLP-1 secretion after oral glucose or meals appears to correlate with improved insulin sensitivity after BPD [36]. The factors contributing to improved insulin sensitivity remain unclear, although insulin sensitivity after BPD is greater after an oral than an intravenous challenge, consistent with a mechanism linked to bypass of the duodenum and jejunum [38]. In addition, the diversion of bile acid to the ileum leads to decreased lipid absorption and increased bile acid reabsorption, which may affect glucose metabolism through increased binding of bile acids to farnesoid X receptor (FXR ) [39, 40]. Increased activation of FXR in the ileum activates fibroblast growth factor-19 (FGF-19), which then binds to the fibroblast growth factor receptor-4 (FGFR-4) and suppresses hepatic gluconeogenesis [40]. This mechanism is also likely to contribute to improved insulin sensitivity after RYGB and SG.
While the long-term effects of SG on weight loss and glycemic control need further study, SG has demonstrated metabolic effects beyond that of purely restrictive procedures such as LAGB. Changes in insulin sensitivity after SG are thought to be similar to RYGB, although results have been discrepant between studies [41–44]. Abbatini et al. compared LAGB, SG, and RYGB and observed that T2DM improved similarly for RYGB and SG. Sleeve gastrectomy actually led to greater improvement in insulin sensitivity than RYGB when assessed by a euglycemic hyperinsulinemic clamp in the absence of any hypoglycemic medication [43]. Another study that assessed insulin sensitivity by applying a mathematical model to data from a mixed meal tolerance test found greater improvement in insulin sensitivity after RYGB than SG [41]. Differences in study population and mechanistic studies may account for the discordant results, but further research into the impact of sleeve gastrectomy on glucose homeostasis is needed.
In summary, insulin sensitivity improves after bariatric surgery and weight loss. Initial improvement occurs largely due to caloric restriction and primarily reflects improved hepatic insulin sensitivity, while subsequent improvement in peripheral insulin sensitivity occurs secondary to weight loss and possibly due to mechanisms specific to the surgical technique.
10.3 β-Cell Function and Insulin Secretion
Assessment of β-cell function is complicated because β-cells adapt to chronic stimuli with a standard set point of secretory capacity, but must also be able to respond to acute challenges such as feeding by promptly releasing sufficient insulin to control glycemia. Bariatric surgery leads to greater insulin sensitivity and insulin action which relieves secretory pressure on the β-cell and leads to a decrease in total insulin secretion; it also improves β-cell response to dynamic changes.
Basal β-cell function is reflected in fasting insulin and total insulin secretion. β-cell responsiveness to dynamic change is assessed using parameters such as the insulinogenic index (change in insulin relative to change in glucose over an interval of time), acute insulin response (AIR) to intravenous glucose, and β-cell glucose sensitivity (measured as the slope of the insulin secretion to plasma glucose dose-response relationship) [45, 46]. Disposition index (DI) is an overall measure of β-cell function that combines both insulin sensitivity and insulin secretion [15]. Comparison of insulin secretion patterns and changes in peripheral insulin and glucose levels should take into account the altered dynamics of accelerated transit and absorption of nutrients after procedures such as RYGB, SG, and BPD.
After LAGB, the insulin curve is characterized by a parallel downward shift in concentration, consistent with increased insulin sensitivity [47, 48]. Early and dynamic insulin secretion is not necessarily improved, but DI increases after at least moderate weight loss [30, 33, 47]. Overall peripheral insulin levels decrease in proportion to the degree of weight loss and are more consistent with increased hepatic clearance than reduced insulin secretion [12].
Insulin secretion after oral ingestion of nutrients is singularly altered after RYGB, with an earlier and exaggerated rise in insulin concentration followed by rapid decline [28, 35, 48–53]. Intrinsic β-cell function recovers early on after RYGB, with several studies reporting an improved acute insulin response [18, 26, 31, 35, 47, 52, 54, 55], although residual glucose intolerance persists [35, 49, 53]. Fasting insulin and total insulin output decrease after RYGB [31, 35, 48]. Insulinogenic index also increases after RYGB [31, 49, 53, 57]. In contrast to LAGB, β-cell glucose sensitivity increases early after RYGB in response to an oral glucose challenge, although responsiveness to intravenous glucose is most notable several months after surgery, in parallel with weight loss [25, 35, 47]. Dutia et al. found that β-cell glucose sensitivity , DI, and insulin secretion rapidly and markedly improved after an oral glucose tolerance test but these early changes were less apparent after an intravenous glucose tolerance test, highlighting the importance of the oral route and gastrointestinal factors in the improvement of β-cell function after RYGB.
Further evidence of the impact of altered nutrient transport and glucose absorption after RYGB on β-cell function and insulin action is provided by the rare cases of severe postprandial hypoglycemia that arise only after RYGB but not LAGB [57–59]. Islet cell hypertrophy may be involved based on surgical specimens from symptomatic patients treated with partial pancreatectomy, but this has been disputed by later studies [58–60]. While the pathogenesis of this syndrome remains unclear, the condition is characterized by excessive insulin response despite improved insulin sensitivity [57, 61]. Given the exaggerated GLP-1 response following RYGB, it has been hypothesized that enhanced GLP-1 secretion contributes to excessive insulin response, and affected patients have been found to have greater insulin and GLP-1 response to meal tests relative to other postsurgical patients without postprandial hypoglycemia [57, 61–63]. One study suggested that postprandial hypoglycemia could be successfully treated with a GLP-1 receptor antagonist, which avoided postprandial hypoglycemia by decreasing the exaggerated release of GLP-1 and insulin [63].
In contrast to the exaggerated insulin response after RYGB, the major effect of BPD is a rapid and significant decrease in insulin resistance [37]. Total insulin secretion decreases in parallel with the increase in insulin sensitivity in all patients after BPD, regardless of baseline glucose tolerance [36, 64]. Improvement in insulin sensitivity and β-cell glucose sensitivity, as well as acute insulin response and DI, occurs as early as 1 week after BPD, before significant weight loss has occurred [36–38, 64]. The early increase in insulin sensitivity and greater insulin response after oral glucose challenge but not to intravenous glucose challenge implicate early and rapid delivery of unabsorbed nutrients to the distal small intestine as a mechanism for improvement in T2DM [38].
Sleeve gastrectomy , like RYGB, leads not only to improved insulin sensitivity but improved insulin secretion and an exaggerated postprandial increase in GLP-1 [42, 43, 65, 66]. Insulin sensitivity improves rapidly and markedly after SG and fasting insulin decreases similarly to RYGB [7, 42, 67]. While further studies are needed, an increase in the insulinogenic index and early insulin response after SG correlates with baseline C-peptide levels, suggesting that SG similarly increases β-cell function [67].
In summary, β-cell function progressively increases over time according to weight loss after restrictive procedures, leading to lower fasting insulin and total insulin secretion. RYGB and BPD also improve the dynamic responsiveness of the β-cell often before significant weight loss has even occurred. While altered nutrient transit and glucose absorption may partly explain the changes in acute insulin response and β-cell glucose sensitivity, concurrent changes in gut hormone secretion also influence further adaptation of β-cell function .
10.4 Mechanisms for Changes in Insulin Action and β-Cell Function
Caloric restriction and weight loss clearly play a significant role in the improvement of insulin resistance and β-cell dysfunction after bariatric surgery. However, early and profound improvement in insulin sensitivity and insulin action, before significant weight loss has even occurred, as well as the magnitude of improvement after surgeries such as RYGB and BPD, suggests that mechanisms independent of weight loss and specific to the surgical intervention may also account for the sustained and marked improvement of T2DM.
10.4.1 Gut Hormone Secretion
Altered nutrient transit after RYGB, SG, and BPD lead to changes in glucose absorption as well as the secretion of gut-derived hormones from enteroendocrine cells. The incretin effect , which is the greater insulin response after oral glucose compared with an equivalent intravenous glucose dose, is diminished in T2DM [68]. GLP-1 and glucose-dependent insulinotropic polypeptide (GIP) are the two incretins responsible for one-half to two-thirds of insulin secretion. GLP-1 is secreted mostly from ileal L cells while GIP is secreted from duodenal K cells, and both are rapidly inactivated by the enzyme dipeptidyl peptidase IV (DPP-IV). GLP-1 analogues and DPP-IV inhibitors have been developed as antidiabetic medications, stressing the importance of GLP-1 in glucose regulation [69, 70].
Multiple studies have observed that postprandial secretion of GLP-1 is substantially and durably increased after RYGB, but not after LAGB or diet-induced weight loss [27, 28, 32, 48, 52, 62, 71]. The increase in GLP-1 occurs early after RYGB, before significant weight loss has occurred, and is clearly related to the more rapid delivery of nutrients to the distal small intestine [27, 71–73]. Recovery of the incretin effect has been associated with exaggerated GLP-1 secretion even after equivalent weight loss [71], although the insulinotropic effect of GLP-1 on β-cell function may be smaller than previously thought. Pharmacologic blockade of GLP-1 receptors with exendin (9-39) only minimally decreases β-cell glucose sensitivity, glucagon suppression, and insulin secretion [74–76]. Interindividual variability in GLP-1 receptor response to GLP-1 may also play a role in the variable weight loss and range of glucose control achieved after RYGB [77].
Postprandial changes in GIP after RYGB have been inconsistent, likely reflecting variability in surgical technique, test meal composition, and timing of blood sample collections [28, 29, 48, 78]. BPD leads to decreased fasting and stimulated GIP, while GLP-1 is increased but to a smaller extent than seen after RYGB [36]. Decreased GIP activity, as in GIP-receptor knockout mice, has been shown to cause hyperglycemia and impaired insulin secretion after oral administration of glucose, suggesting a role for GIP in the acute insulin response [79]. GIP infusion has been shown to mildly increase insulin secretion and decrease glucagon [80]. Decreased GIP secretion after bariatric surgery may reflect restoration of the insulinotropic effect of GIP, which is impaired in T2DM [68, 81]. Although GIP may not mediate early recovery of β-cell function or insulin secretion, decreased GIP may contribute to decreased glucagon postoperatively and further potentiate incretin response by decreasing glucotoxicity and secretory pressure on the β-cell.
Decreased ghrelin , an orexigenic peptide produced in the fundus and body of the stomach, is thought to decrease hunger and may also lead to lower HbA1c after RYGB and VSG [66, 82, 83]. Ghrelin increases after diet-induced weight loss and LAGB, where it correlated with insulin levels and hepatic insulin clearance but not insulin sensitivity [32, 84, 85]. Reports of ghrelin levels after RYGB have been inconsistent, with some groups reporting a decrease while others have noted lack of change or an increase [50, 52, 86–88]. Differences between studies such as varying degrees of weight loss, postoperative intervals, study time points, and the use of assays measuring “active” octanoylated isoform versus total ghrelin likely account for the discrepant results. SG, through its resection of the fundus as well as a greater part of the stomach body, leads to decreased fasting and postprandial ghrelin [66]. While decreased ghrelin may attenuate hunger and contribute to further weight loss and thereby affect insulin sensitivity, it does not appear to influence insulin secretion or insulin sensitivity directly .
10.4.2 Altered Nutrient Flow
Early and rapid delivery of unabsorbed nutrients to the distal small intestine may activate the “ileal brake” that potentiates the secretion of GLP-1 and Peptide YY, an anorexigenic hormone also elevated after RYGB that is known to slow intestinal transit time, increase satiety, and delay gastric emptying. Exaggerated GLP-1 secretion helps to improve insulin secretion and insulin sensitivity while PYY facilitates maintenance of glycemic control by decreasing food consumption. Consistent with this hypothesis is the observation that the procedures most consistently leading to improved T2DM shorten the route of nutrient flow from the stomach to the intestine and increase the rate of transport of ingested nutrients [5, 7, 13, 30, 66].
Rodent models of ileal interposition (IT) , a surgical procedure whereby a segment of the ileum is inserted into the proximal intestine so that there is no gastric restriction or duodenal bypass, provide further evidence for the role of the distal ileum in improving glucose tolerance. IT in different rodent models resulted in elevated levels of GLP-1 and PYY and improvements in glucose tolerance, insulin sensitivity, and β-cell function [89–93]. Improvement in oral glucose tolerance after IT was reversed by inhibition of GLP-1 receptors with exendin (9-39), reinforcing the hypothesis that early and increased GLP-1 receptor activation contributes to improved insulin action after RYGB [93].
Bypass of the proximal small intestine and exclusion of nutrient transit through the duodenum and jejunum has also been hypothesized to contribute to improvement in T2DM. Duodenal-jejunal bypass (DJB) without gastric restriction in animal studies of both nonobese and diet-induced obese diabetic rats led to improvement in hyperglycemia independent of food intake and weight reduction [78, 94, 95]. Intestinal glucoregulatory hormones and vagal innervation may contribute to alleviation of hyperglycemia in this DJB model [95]. In humans, DJB had only a moderate effect with improvement in HbA1c that mildly deteriorated by 12 months after surgery [96]. It is unclear if the glycemic impact of DJB lies in its alteration of the intestinal site of nutrient delivery or in its moderate weight loss.
Further evidence that exclusion of the proximal small intestine may affect glucose homeostasis through unknown mechanisms can be derived from more recent studies of endoluminal sleeves (ELS) that allow nutrients to flow from the pylorus to the jejunum without contacting duodenal mucosa. Aguirre et al. demonstrated that despite less weight loss than similar rats that had undergone RYGB, rats treated with ELS attained similar improvement in glycemic control. Studies in humans have also demonstrated reduced HbA1c after various endoluminal duodenal-jejunal bypass sleeves, although investigation into safety, efficacy, and long-term outcomes are ongoing [97, 98].
10.4.3 Bile Acids
Increased bile acid reabsorption has been proposed as a potential mechanism for improved insulin sensitivity after RYGB and BPD, but not LAGB [39, 40, 98–104]. After RYGB, increased bile acids were two-fold higher and positively correlated with peak GLP-1 and adiponectin, and inversely correlated with 2-hour postprandial glucose and thyroid stimulating hormone (TSH), implicating altered bile acid concentrations as a mechanism in glucose and lipid metabolism. Bile acids may affect insulin action through binding to the TGR5 receptor on L-cells, which release GLP-1 [99, 100]. Gerhard et al. also showed that diabetic patients achieving remission after RYGB had larger increases in fasting bile acids than non-diabetics or diabetics who did not go into remission[ [98].
Altered enterohepatic recycling of bile acids and consequent increase in serum bile acids induced by IT in rats also suggest a mechanism for improved glycemia after BPD. Increased bile acids bind to FXR and stimulate secretion of FGF19, which subsequently inhibits hepatic glucose production and improves insulin sensitivity [39, 40]. Whole body FXR knock-out mice have been shown to be refractory to the metabolic improvements seen in wild-type mice after SG [103]. In prospective human and animal studies, Pournaras et al. concluded that RYGB but not LAGB causes more rapid delivery of bile acids to the terminal ileum and therefore higher total bile acid levels, plasma GLP-1, PYY, and FGF19 [101]. Other studies have similarly found increases in plasma bile acids and FGF19 after RYGB, but Kohli et al observed that surgery-induced increase in bile acids did not correlate with postprandial insulin secretion or insulin sensitivity [100, 105].
10.4.4 Microbiota
Data from mouse studies and humans have provided evidence that gut microbiota may play an important role in energy storage and possibly the development of obesity and associated complications, although it remains unclear whether changes in the gut microbiome are a consequence or cause of obesity [107]. Bariatric surgery, though inducing anatomic, systemic, and environmental changes, may impact the composition of gut microbiota. Several studies have suggested that bariatric surgery shifts bacterial flora in obese patients toward profiles more similar to that of lean patients. Altered anatomy may play a key role in the changes in microbiota, as differences in microbial ecology were most notable distal to the site of surgical manipulation in rat models [107–110]. Transfer of gut microbiota from RYGB treated mice to non-operated, aseptic mice has been shown to be sufficient to cause decreased weight and adiposity, possibly due to altered microbial synthesis of short-chain fatty acids [108]. Whether changes in microbiota affect insulin sensitivity or insulin secretion directly is unknown.
10.4.5 Insulin Clearance
Early improvement in hepatic gluconeogenesis and hepatic insulin sensitivity may account for the rapid decrease in fasting glucose and fasting insulin after RYGB. Bojsen-Moller et al. found that fasting hepatic insulin clearance increased as early as 1 week and further at 3 months for both diabetic and normoglycemic patients after RYGB. Postprandial insulin clearance increased only in the T2DM patients, in whom the increased insulin clearance occurred as early as 1 week following surgery and persisted at 3 months and after 1 year [25]. Based on this study and others who have noted rapid improvement in fasting glucose and fasting insulin before significant weight loss, it becomes plausible that nonenteral factors such as increased hepatic insulin sensitivity and insulin clearance account for some of the early improvement following caloric restriction [25, 111]. Early postoperative increases in plasma free fatty acids are followed by increased suppression consistent with improved peripheral insulin sensitivity with progressive weight loss, as demonstrated during clamp studies in T2DM patients 1 year after RYGB [25 ].
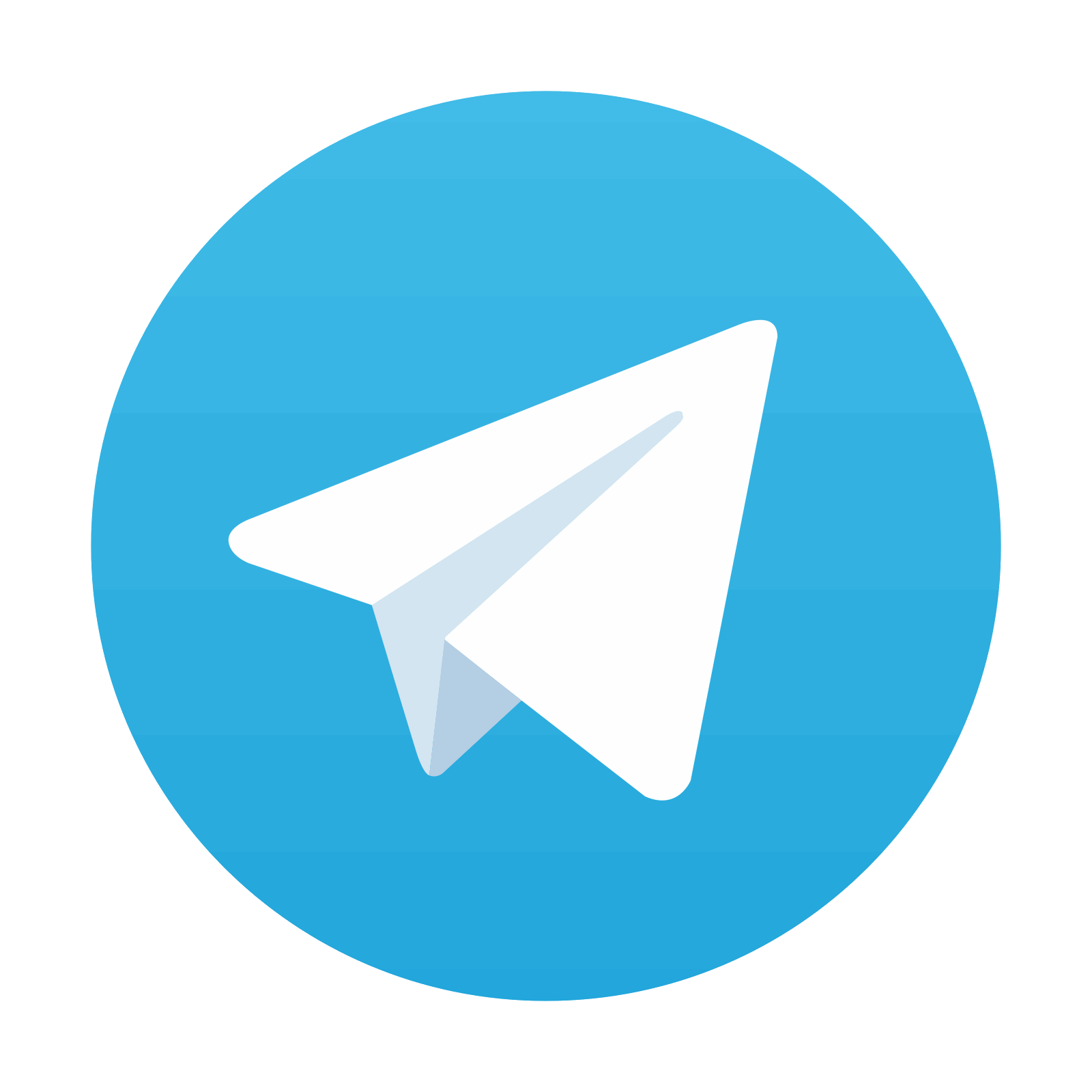
Stay updated, free articles. Join our Telegram channel

Full access? Get Clinical Tree
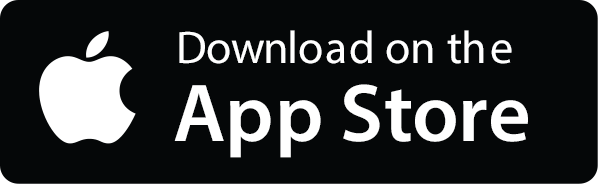
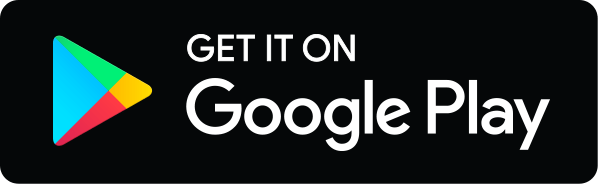