Fig. 5.1
DECT post-processing workflow. (a) 100 kVp dataset, (b) Sn150 kVp dataset, (c) 120 kV mixed image, (d) virtual unenhanced image, (e) color-coded iodine map, (f) virtual monoenergetic image (50 keV)
The calculation of VU images may replace the acquisition of pre-contrast images, therefore substantially lowering the radiation burden especially in younger patients who are more at risk for radiation-induced consequences or patients who undergo repeated follow-up CT examinations. Iodine maps can be useful to improve visualization and detection of contrast uptake, potentially increasing the diagnostic confidence for assessment of metastatic lesion contrast enhancement or conversely ruling out the presence of any contrast enhancement in small benign cystic lesions. Virtual monoenergetic images at low energy levels are beneficial in increasing image contrast, thus potentially improving the visualization and assessment of both hypovascular and hypervascular liver lesions. Due to increased image contrast, virtual monoenergetic imaging may also allow for a reduction of contrast material administered. These capabilities may have beneficial effects on the detection of cancer-related comorbidities and the evaluation of treatment response (Table. 5.1) [2]. All these applications, if routinely applied, could improve the safety of CT examinations by reducing the radiation dose and contrast medium amount administered, while at the same time enhancing the detection and characterization of liver lesions.
Table 5.1
Advantages of DECT datasets in liver imaging
DECT of liver tumors | |
---|---|
Dataset | Advantages |
Virtual unenhanced | Replacement of conventional unenhanced acquisition |
Radiation dose reduction | |
Iodine mapping | Intralesional iodine detection |
Iodine uptake quantification | |
Response to therapy assessment | |
Virtual monoenergetic | Liver lesion detection |
Liver lesion conspicuity | |
Contrast material reduction |
5.2 Material-Specific Applications
5.2.1 Virtual Unenhanced Images
Material-specific images may be used for determining the presence and quantity of materials with unique absorption characteristics, including chemical elements such as iodine, iron, calcium, and gadolinium, or compound materials such as fat, uric acid, or blood.
In particular, detection and computed removal of iodine enables the calculation of VU images, which may obviate the need of routine conventional unenhanced (CU) scans (Fig. 5.2). This may be of special relevance in follow-up liver CT, which is commonly performed using biphasic or triphasic scan protocols which result in a relatively high radiation dose. VU images may allow for a reduction of approximately 30 % in clinical practice [3–6].
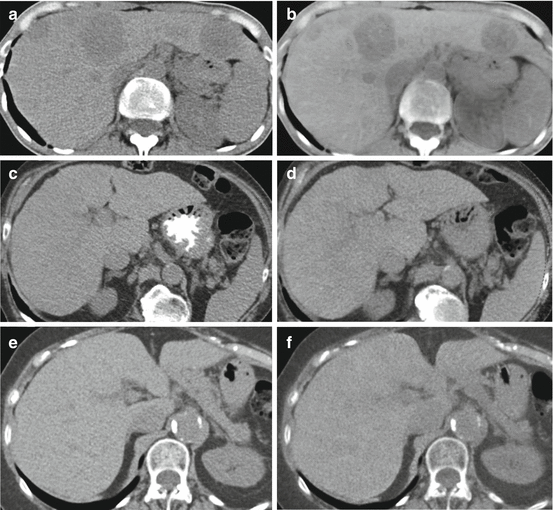
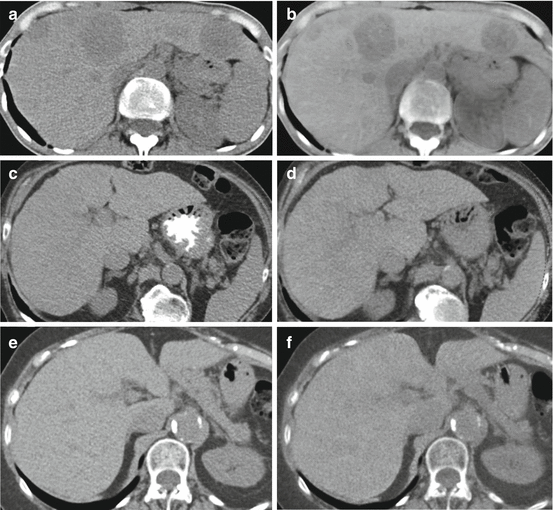
Fig. 5.2
Comparison of virtual unenhanced images obtained with dual-source dual-energy scanners from different generations. (a, c, e) Conventional unenhanced datasets obtained with first, second, and third generation dual-source dual-energy CT, respectively; (b, d, f) corresponding virtual unenhanced images reconstructed from the venous phase. A significant improvement in image quality and iodine subtraction from the liver parenchyma is evident in each generation compared to the last
First- and second-generation dual-source DECT abdominal VU datasets, despite mostly excellent image quality, were not ready to replace CU images in clinical practice due to limitations that could impair diagnostic accuracy such as inhomogeneous iodine subtraction and reduction in the attenuation of calcifications and metallic clips [3–6]. In particular, inhomogeneous iodine subtraction has been reported in the presence of high iodine concentration, e.g., in small peripheral liver vessels. A lack of contrast subtraction on VU images has also been observed in the case of liver metastasis as a ring artifact around the lesion. Furthermore, erroneous calcium subtraction was shown and could interfere with characterization of calcified liver lesions. While these artifacts do not affect the image quality, they could be the source of pitfalls in certain clinical cases.
Recently, third-generation dual-source CT has become commercially available that provides several technological innovations which may be beneficial in DECT. A preliminary report on VU images reconstructed with this scanner demonstrated good quality of VU images and, unlike second generation, a complete subtraction of liver iodine has been reported [7] (Fig. 5.3). However, the issue of erroneous subtraction of calcified aortic lesions (Fig. 5.4) and metallic clips persists, as it is intrinsic to the technique; thus, radiologists should be aware of these limitations when incorporating VU datasets into their DECT protocols.
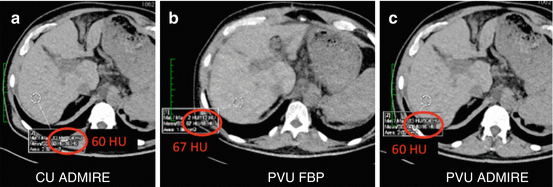
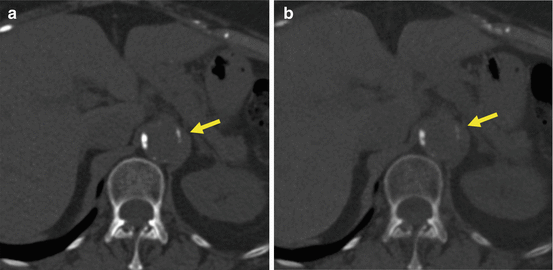
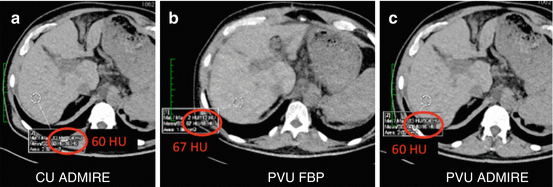
Fig. 5.3
Third-generation conventional (a) and virtual unenhanced (b, c) datasets from the venous phase reconstructed with filtered back projection (FBP) and advanced modeled iterative reconstruction algorithm (ADMIRE, strength 3). Virtual unenhanced datasets show similar liver attenuation values as conventional unenhanced images, demonstrating a complete subtraction of contrast signal from the liver parenchyma. ADMIRE significantly improved overall image quality
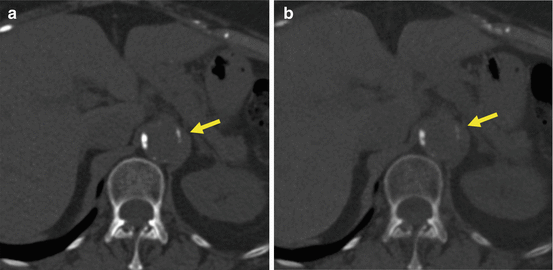
Fig. 5.4
Third-generation conventional unenhanced image (a) and virtual unenhanced image from the arterial phase (b). A reduction in the attenuation of aortic calcifications (arrow) can be observed in the virtual unenhanced dataset
Future improvements in DECT post-processing may alleviate these issues to transform VU image reconstruction into a routine technique in DECT to reduce radiation exposure.
5.2.2 Iodine Mapping
DECT-derived maps of iodine distribution in the liver parenchyma can be used to identify iodine uptake in tumors and can act as a surrogate for lesion vascularization. In fact, DECT can provide a direct and accurate quantification of the iodine uptake in milligrams per milliliters (mg/ml) (Fig. 5.5). Besides its diagnostic value for lesion characterization (i.e., differential diagnosis of a hepatic lesion too small to differentiate between a tiny cyst and a solid metastasis) (Fig. 5.6), DECT iodine maps may also be beneficial for the assessment of lesion response after therapy.
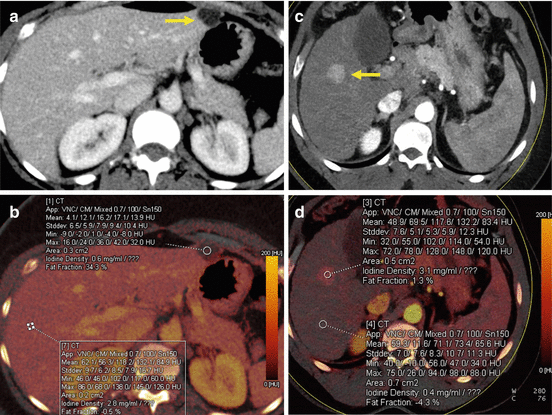
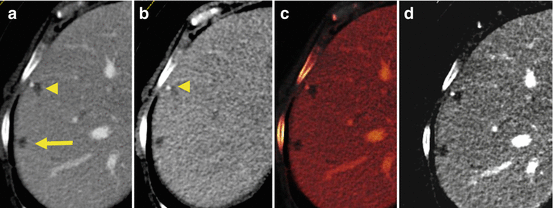
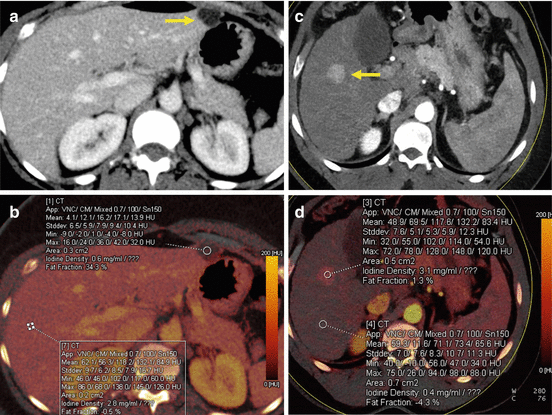
Fig. 5.5
DECT-derived maps of iodine distribution in the liver parenchyma can be used to identify and quantify iodine uptake of liver lesions. (a, b) Benign cystic lesion (arrow) showing no contrast enhancement (iodine density: 0.6 mg/ml) in comparison with the liver parenchyma in the venous phase (iodine density: 2.8 mg/ml). (c, d) HCC nodule with significant iodine uptake (iodine density: 3.1 mg/ml) during the arterial phase in comparison with the surrounding liver parenchyma (iodine density: 0.4 mg/ml). HCC hepatocarcinoma
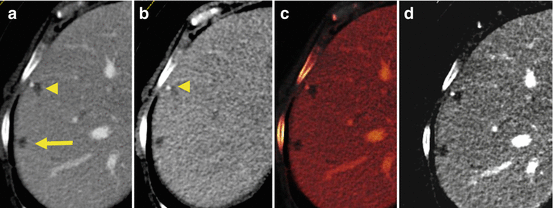
Fig. 5.6
The combination of virtual unenhanced images and iodine maps can be used to improve diagnostic confidence in liver lesion characterization. In patients with colorectal cancer, the discrimination of small liver cysts from metastatic disease can represent a diagnostic dilemma (a, arrow and arrowhead). Virtual unenhanced images (b) demonstrate the presence of a small lesion with calcification (arrowhead). Iodine-based maps (c, d) show the absence of any intralesional contrast uptake, suggesting a diagnosis of benign liver cysts
Recently, the assessment of lesion-specific iodine concentration has been used to differentiate hepatic hemangiomas from hepatocellular carcinoma (HCC) [8]. Atypical hemangiomas, which are frequently observed in patients with liver cirrhosis, represent an imaging challenge when attempting to differentiate them from HCC [9]. The capability to employ quantification of iodine concentration to differentiate these two entities may render DECT an important tool in the surveillance of cirrhotic liver parenchyma. Furthermore, in patients with colorectal carcinoma, the early detection of liver metastasis may positively impact treatment options and outcome. In the presence of small liver nodules in these patients, the reliable differentiation of small benign cysts from metastasis may not be feasible with CT in cases. The accurate detection and assessment of iodine in the lesion may thus improve the diagnostic confidence for lesion characterization [1].
5.3 Energy-Specific Applications
5.3.1 Low kVp Images
A general advantage of DECT in liver imaging is the possibility to simultaneously obtain two different image datasets at low (80–100 kVp) and high (140–150 kVp) tube voltages and therefore energy spectra.
Since in low kV datasets are acquired with energy levels closer to the k-edge of iodine, a mean significant increase in attenuation can be observed, in particular in contrast-enhanced structures. This represents a well-known advantage in vascular studies in which a significant increase in signal-to-noise ratio (SNR) and contrast-to-noise ratio (CNR) can be achieved, with better visualization of contrast-enhanced vessels, accompanied by a distinct reduction in radiation dose in SE acquisition. This approach can also be applied to the detection of hypervascular liver lesions. For the evaluation of late arterial phase 80-kVp datasets in comparison to standard 120-kVp images, several studies demonstrated a significant improvement in HCC detection and lesion conspicuity (Fig. 5.7). Apparently, the associated increase in image noise does not impair detection of small hepatic lesions [10, 11]. However, Park et al. did not observe an improvement in HCC detection with 80-kVp images despite significantly higher CNR [12]. Since the increased attenuation of iodine in low-kVp images comes at the cost of increased image noise, overall image quality and CNR may be lower especially in obese patients. Nevertheless, the recently introduced third-generation DSCT system provides higher tube currents in low-kVp imaging which may overcome this limitation.
