6. Drug–nutrient interactions
Rebecca White
LEARNING OBJECTIVES
By the end of this chapter the reader will be able to:
• Explain the effect of nutritional status on drug metabolism;
• Demonstrate awareness of the effect of drug therapy on nutritional intake;
• Define drug effects on specific nutrients: vitamins, minerals and macronutrients;
• Discuss nutrient effects on drug therapy;
• Identify drug administration issues in enteral nutrition; and
• Identify patients ‘at risk’ of drug-nutrient interactions.
Introduction
A drug–nutrient interaction is any interaction between a drug and specific nutritional compound or a drug-induced effect on nutritional intake; these can be both positive and negative in clinical consequence and vary in significance. There are many mechanisms by which these interactions can occur.
Drugs that affect nutrition can be broadly categorised as drugs that affect oral intake, drugs that affect nutrient absorption and drugs that affect nutrient metabolism.
The effect of nutritional status or specific nutrients on drug therapy has been extensively researched and falls into two main categories: the effect of nutritional status on drug metabolism and storage and the effect of nutrient intake on drug metabolism.
Drug metabolism occurs in four steps: absorption, distribution, metabolism and excretion. A drug–nutrient interaction can occur at any stage in this process.
This chapter aims to give clinically relevant examples of drug–nutrient interactions in all these areas.
Effects of nutritional status on drug metabolism
Effects of malnutrition
It has long been known that malnutrition per se reduces the ability to effectively metabolise drugs and increases the incidence of adverse effects. 1
Malnutrition can significantly affect drug distribution, as a severely malnourished patient will have reduced plasma proteins for drug binding, increasing the free circulating concentrations; an increase in total body water, increasing the volume of distribution of water-soluble drugs; decreased fat stores, decreasing the volume of distribution for fat-soluble drugs; and reduced microsomal enzyme activity and reduced substrates available for metabolism. 2 These factors should be considered, particularly when dosing drugs with narrow therapeutic ranges.
Effects of obesity
Drug metabolism in obesity is a growing research area as the prevalence of obesity increases. Drug disposition in obese individuals is dependent on body composition, where 60–80% of the excess weight may be adipose tissue. 3 However, it is the lean body mass that correlates with total body water, metabolic activity and drug clearance. Therefore loading doses of drugs may be affected by lean body mass, and maintenance doses affected by excess fat mass.
Weight-based dosing is a cause for discussion in obese individuals. However, there are many other aspects of drug dosing that need consideration. There are no data to suggest that intestinal absorption of drugs in this patient group is any different to those with normal body weight. There are also no data relating to transdermal and intralipomatous drug delivery in this patient group.
It should not be assumed that lipophilic drugs will have a larger volume of distribution in obese individuals. 4
Recommendations on the use of actual body weight and adjusted body weight vary depending on the drug. For example, it is recommended that neuromuscular blocking agents should be dosed on lean body weight; an adjusted body weight plus 40% is recommended for gentamicin dosing, 5 whereas total body weight is recommended for vancomycin dosing. 6 Particular care should be taken when using actual body weight to calculate body surface area for chemotherapy dosing. 7
Effect of drug therapy on nutritional intake
There are many ways that drug therapy can compromise nutritional intake. Gastrointestinal disturbances are the most commonly reported side effects of drug therapy. 8 These commonly include abdominal discomfort, nausea, vomiting, constipation, diarrhoea, taste disturbances and anorexia, all of which may have an adverse effect on nutritional intake.
There are also circumstances where appropriate use of drug therapy can improve nutritional intake such as the use of steroids as appetite stimulants or prokinetics to aid toleration of enteral nutrition. At the other extreme, drug therapy is also used in the management of obesity.
There are many drugs that have specific dietary guidance relating to dosing. Several antibiotics are recommended to be taken ‘on an empty stomach’ or ‘half an hour before food’; others are recommended ‘not to be taken at the same time as milk’. These restrictions are necessary to optimise the oral absorption of these therapies due to a significant drug nutrient interaction. These can reduce oral intake due to concerns about taking medication on an empty stomach. 8
The bisphosphonates alendronate and risedronate are required to be taken on waking, half an hour before food, as co-administration with food reduces the absorption to negligible levels. 9 This may affect nutritional intake at breakfast, considered by some to be the most important meal of the day.
Nausea and vomiting
Most drugs are capable of causing nausea or vomiting depending on how and when they are taken. However, there are several classes of drugs with a high incidence of nausea and vomiting, the cytotoxic agents being the most extreme example.
Drugs can cause vomiting through a direct action on the chemoreceptors located in the stomach, jejunum and ileum; these are the same receptors that respond to other toxins. This neuronal pathway leads to the vomit centre, which is where multiple neuronal pathways meet, including those from the chemoreceptor trigger zone (CTZ), higher cortical centres, vagal sensory pathways and the labyrinths (Box 6.1).

Chemoreceptors in GI tract | CTZ |
---|---|
• Cytotoxics | • Cytotoxics |
• Potassium | • Anaesthetics |
• Iron preparations | • Opiates |
• Antibiotics | • Nicotine |
• Levodopa | |
• SSRI (newer antidepressants e.g. fluoxetine) |
Drugs can also act directly on the serotonin and dopamine receptors in the CTZ, the CTZ being outside the blood–brain barrier and therefore can respond to stimuli from both the blood or the cerebrospinal fluid.
The emetic effect of local irritants such as the potassium and iron salts can be minimised when the drugs are taken with food. The emetic effects of other therapies are usually managed with co-prescription of anti-emetic medication.
Taste disturbances
Altered taste sensation or loss of taste can significantly affect nutritional intake by changing food choice patterns or reducing food intake.
Changes to the sense of taste may be broadly divided into either loss or alteration of taste. Loss of taste may be either incomplete, hypogeusia, or complete, ageusia. Taste alteration, dysgeusia, may occur as aliageusia in which stimuli such as food or drink produce an inappropriate taste or as phantogeusia, sometimes referred to as gustatory hallucination, in which an unpleasant taste is not associated with an external stimulus.
Taste disturbances have many causes including infections, metabolic or nutritional disturbances, radiation, CNS disorders, neoplasms and drug therapy, or may occur as a consequence of normal aging. Management primarily consists of treatment of any underlying disorder. Numerous drugs are associated with taste disturbances, usually described as metallic or bitter; withdrawal of the drug is commonly associated with resolution but occasionally effects persist and may require treatment (Box 6.2). 10

Fluconazole | Sulphonylureas |
Metformin | Terbinafine |
Azelestine | Idoxuridine |
Zidovudine | Selenium (toxicity) |
Zinc (deficiency) | Propafenone |
Clindamycin | Levamisole |
Zopiclone | Linezolid |
Metronidazole | Sodium aurothiomalate |
Amiodarone | Amitriptyline |
Levodopa and combination drugs | Captopril |
Losartan | Simvastatin |
Sulfasalazine | Griseofulvin |
Lithium | Rifampicin |
Allopurinol | Penicillamine |
Effects on gastrointestinal motility
Both a decrease in motility leading to bloating and fullness or an increase in motility causing loose stools or diarrhoea can affect nutritional intake.
Drugs commonly associated with decreased GI motility are the opiates and drugs with anticholinergic activity (Box 6.3a).

Anticholinergic
• Tricyclic antidepressants e.g. amitriptyline
• Oxybutynin
• Propantheline
• Benzhexol
• Benztropine
• Procyclidine
• Dicyclomine
Opiate
• Morphine
• Codeine
• Ondansetron
Drug therapy can cause diarrhoea by a number of mechanisms. Increasing gut motility, alteration of gut flora or disturbance of the mucosal surface can all result in diarrhoea. A large number of drugs are associated with diarrhoea, which may result in a decrease in oral intake as the patient attempts to minimise this side effect (Box 6.3b).

• Prokinetics; erythromycin and metoclopramide
• Antibiotics
• Auranofin (affects 50%)
• Misoprostil (affects 8%)
• Proton pump inhibitors, e.g. lansoprazole (affects 1–3%)
• Antivirals; adefovir, tenofovir, lamivudine
• Magnesium salts
• Iron
• Lithium (sign of toxicity)
• Digoxin (sign of toxicity)
• Acarbose
• Sevelamer
• Metformin
• Colchicine (sign of toxicity)
Weight gain
Weight gain secondary to drug therapy can be either a desirable or an undesirable outcome.
Psychotropic medication is the most common group associated with clinically significant weight gain; however, there are other commonly used medications which are also associated.
Drugs causing weight gain through stimulation of appetite are used clinically in the management of anorexia and cachexia secondary to cancer and HIV. The corticosteroids remain the most commonly used agents in the UK for appetite stimulation in cancer cachexia. 11 There is increasing evidence for the use of anabolic steroids in HIV-associated cachexia (Box 6.4). 12

Amitriptyline | Chlorpromazine |
Clozapine | Olanzapine |
Lithium | Valproic acid |
Mirtazapine | Beta blockers |
Insulin | Oral contraceptives |
Oestrogen | Testosterone and derivatives |
Anabolic steroids |
Weight loss
Weight loss secondary to drug therapy is predominantly due to two reasons: loss of appetite, anorexia, leading to reduced nutritional intake, or an increase in the basal metabolic rate.
Anorexia is a commonly reported side effect of many drugs; however, there are a few examples where this is severe enough to be associated with significant weight loss (Box 6.5).

• Metformin
• Procainamide
• Spironolactone
• Digoxin
• Penicillamine
• Amantadine
• Fluoxetine
Drug effects on specific nutrients
Drug therapy can affect nutrients at every stage of their metabolism in the same way that nutrients can affect drugs at any point in the metabolic process. Most reports of drug–nutrient interactions relate to absorption. Drugs can cause the malabsorption of nutrients in several different ways. They can bind directly to the nutrient and prevent or reduce its absorption, they can compete for the active transport pathway used by the nutrient or they can reduce absorption through a direct effect on the intestinal mucosa.
Drugs such as the antacids which contain aluminium, magnesium or calcium salts and foods rich in copper, calcium, zinc, magnesium and iron are most often responsible for drug chelation or the formation of insoluble salts, usually preventing the absorption of both drug and nutrient components, e.g. quinolone antibiotics and calcium, cholestyramine and iron.
It is also not surprising that there are distinct interactions between specific nutrients and drug compounds in the metabolic pathway as many drugs owe their pharmacological activity to their interaction with a specific nutrient, such as the dihydrofolate reductase inhibitors, or require vitamin components for their metabolism.
Vitamins
Vitamin A
Vitamin A deficiency leads to visual problems, suppressed immune function, diarrhoea and kidney stones. The mechanism of interaction affecting vitamin A is that of malabsorption (Box 6.6).

• Ethanol
• Cholestyramine
• Mineral oil
• Neomycin
Thiamine (Vitamin B 1)
The co-enzyme thiamine pyrophosphate is essential for decarboxylation and transketolase reactions. Classical signs of thiamine deficiency are lactic acidosis, cardiovascular, cerebral and peripheral neurological impairment. The extent of symptoms that would be seen with subclinical deficiency is uncertain.
There are several studies demonstrating a measurable thiamine deficiency in patients taking furosemide for heart failure. 13,14 Researchers were able to demonstrate an improvement in left ventricular ejection fraction using thiamine supplementation (200 mg daily) in this patient population. 15 However, the direct causal effect of furosemide on thiamine status is not understood and a subsequent study could not confirm earlier findings. 16 At present there is insufficient evidence to recommend routine supplementation of thiamine in this patient population (Box 6.7). 17

• Aminoglycosides
• Cephalosporins
• Digoxin18
• Ethanol
• Fluoroquinolones
• Loop diuretics
• Phenytoin
• Sulphonamides
• Tetracyclines
Riboflavin (Vitamin B 2)
Riboflavin in its active form is required for the electron transport system, and overt deficiency causes rough scaly skin, angular stomatitis, cheilosis, glossitis and stomatitis. Experimental evidence suggests that drugs such as chlorpromazine, imipramine, amitriptyline and Adriamycin may cause riboflavin depletion. 19 Such drugs could increase the risk of deficiency when the dietary intake is poor (Box 6.8).

• Aminoglycosides
• Doxorubicin20
• Cephalosporins
• Fluoroquinolones
• Oral contraceptives
• Phenothiazines
• Sulphonamides
• Tetracyclines
Nicotinic acid (Niacin, Vitamin B 3)
The precursor of niacin is dietary tryptophan. Niacin is used therapeutically in the management of dyslipidaemias at doses of 1.5–3 g daily. Niacin acts on multiple levels of lipoprotein metabolism, by reducing transport of free fatty acids to the liver and decreasing liver triglyceride synthesis. The adverse effect of excessive niacin is flushing and dyspepsia which is aggravated by coffee or tea; it can also cause hepatotoxicity at high doses.
The important co-factors in oxidation–reduction reactions, NAD and NADP, are formed from nicotinic acid. Pellagra, a syndrome of dermatitis, diarrhoea and dementia, is a result of nicotininc acid deficiency. Pellagra-like symptoms have been associated with isoniazid treatment. 21 This is due to pyridoxine antagonism, inhibiting the conversion of tryptophan to niacin. Nicotininc acid deficiency has also been associated with sodium valproate and glibenclamide therapy (Box 6.9). 22,23

Pyridoxine (Vitamin B 6)
Pyridoxine is part of the co-enzymes pyridoxal phosphate (PLP) and pyridoxamine phosphate (PMP) used in amino acid and lipid metabolism. It is also a co-factor for the conversion of tryptophan to niacin and serotonin and involved in the production of red blood cells. Deficiency leads to anaemia, peripheral neuropathy, dermatitis and a sore tongue.
It is well documented that isoniazid causes clinically significant pyridoxine deficiency leading to peripheral neuropathy; pyridoxine (10 mg daily) is routinely added to antituberculosis therapy on a prophylactic basis.
Pyridoxine metabolism is affected by theophyllines effect on liver enzymes, 26 and pyridoxine deficiency has been reported in patients on theophylline. 27 Attenuation of the side effects of theophylline with pyridoxine therapy has been demonstrated in both animal models and patients (Box 6.10). 28,29

Vitamin B 12
Vitamin B 12 depletion is associated with a megaloblastic anaemia, cognitive impairment and peripheral neuropathy. B 12 deficiency is also a causative factor in the development of hyperhomocysteinaemia.
The majority of drugs that affect vitamin B 12 status do so through their effect on its absorption from the gut. There are data to show that up to 30% of patients taking the antidiabetic metformin malabsorb vitamin B 12; 30,31 this interaction is also seen with phenformin, and there have been reports of megaloblastic anaemia as a result of this interaction. 32
The proton pump inhibitor group of drugs, such as omeprazole, and the histamine receptor antagonists, such as ranitidine, reduce the secretion of intrinsic factor and impair the absorption of B 12 although the extent of this interaction is not yet clear. 33
Nitrous oxide inactivates B 12, causing both neurologic and haematological abnormalities. This interaction is only significant if there is chronic exposure to nitrous oxide. The high doses used in anaesthesia are not considered to have a significant effect if exposure is less than 6 hours (Box 6.11). 34

Folate
There are a significant number of drugs that interact with folate; indeed many of them owe their therapeutic activity to this drug–nutrient interaction. This group of drugs are the dihydrofolate reductase (DHFR) inhibitors. Drug therapy may also inhibit the reduction of dietary polyglutamate to monoglutamate, thereby preventing its intestinal absorption. Drugs with a significant affect on pyridoxine and B 12 may also affect folate as both are involved in the demethylation of methylfolate. 36 Drug therapy such as carbamazepine or phenobarbital may accelerate folate metabolism, thereby causing deficiency. 37
Folate deficiency is easily detected as it has a profound effect on haematological parameters; because of its involvement in DNA synthesis a deficiency is also associated with neural tube defects in the developing foetus.
Treatment of the deficiency state depends on the drug involved. If a therapeutic effect is required from the interaction, as in the case of methotrexate therapy in chemotherapy, then the timing of rescue therapy is critical.
Folate can be administered as folic acid or folinic acid (calcium leucovorin). Folic acid requires conversion and therefore is also subject to the inhibitory effects of the dihydrofolate reductase inhibitors, whereas folinic acid is a derivative of the active metabolite tetrahydrofolic acid and therefore is biologically active even in the presence of these drugs.
Concomitant folinic acid therapy can be used to prevent the deficiency-induced side effects of pyrimethamine and trimethoprim without affecting the therapeutic efficacy of the drug on the bacterial cells as they cannot utilise this form of folate.
In the case of sulphasalazine the induced folate deficiency and resultant macrocytic anaemia respond better to folinic acid than folic acid. 38
The use of supplements to treat anticonvulsant-induced folate deficiency is controversial. If phenytoin and folate therapy is initiated concurrently it overcomes the problem of folate deficiency and the phenytoin levels reach steady state sooner than without supplements (Box 6.12). 39

Vitamin C
There are limited data to support drug–nutrient interactions resulting in a significant effect on ascorbic acid. Deficiency of ascorbic acid causes scurvy, usually due to dietary deficit, which is now rare.
Vitamin D
As the majority of vitamin D is produced in the skin it is unsurprising that deficiency has been reported in chronic sunscreen users and institutionalised individuals. 44,45
The anticonvulsants are the most prominant group of drugs with a significant association with vitamin D deficiency, resulting in osteoporosis. 46 It has been suggested that these effects are secondary to effects on hepatic hydroxylation of vitamin D 3 to the active form 1,25-hydroxycholecalciferol. 47
Vitamin E
Vitamin E plays an important role in maintenance of cell membrane integrity. There have been few reports of drug–nutrient interactions involving vitamin E. Cholestyramine appears to reduce vitamin E levels but the clinical significance of this is unknown. It has also been suggested that anticonvulsants may affect vitamin E status, but these claims have not been substantiated.
Vitamin K
Drug–nutrient interactions affecting vitamin K are evident through their effect on prothrombin time and clinical signs such as excessive bruising and bleeding.
Warfarin, and the other coumarins, owe their clinical activity to their effect on the enzymatic reduction of vitamin K. Alterations in the dietary intake of vitamin K can influence the clinical effectiveness of anticoagulant therapy. 50
Vitamin K 2 is synthesised by gut flora in the colon; therefore broad spectrum antibiotics affecting these bacteria can indirectly cause deficiency and affect clotting time. 51
Carnitine
Carnitine is a nonprotein amino acid that is present in tissues with high energy requirements such as the skeletal muscle and myocardium. It is also present in large amounts in such tissues as the liver, kidney and adrenal glands. The major function of carnitine is to transport long-chain fatty acids into the mitochondrial matrix for their oxidation. Carnitine deficiency is a recognised side effect of long-term dialysis; 52 signs and symptoms include muscle cramps, weakness and cardiomyopathy.
Sodium valproate is associated with carnitine deficiency, and although it is unclear whether carnitine supplementation is of value in patients receiving valproate, some neurologists consider it justified in selected cases. 53 There is also evidence suggesting that the hepatic injury caused by sodium valproate may be related to the carnitine deficiency; 54 there is also report of treatment of valproate-induced hyperamonaemia using carnitine. 55
Minerals
Drug–nutrient interactions affecting trace elements are rarely reported due to lack of awareness of clinical signs of isolated trace element deficiency or toxicity and difficulty in measuring and interpreting biochemical tests. Like the vitamins, they are co-factors in many enzymatic processes and therefore it is unsurprising that they may be influenced by drug therapy.
Aluminium
There are no reports of aluminium deficiency in the literature. All associations between drug therapy and aluminium are of toxicity. The symptoms of aluminium toxicity mimic those of Alzheimer’s disease and osteoporosis. Aluminium is a recognised contaminant of large volume parenteral preparations, 58 and high levels are seen in dialysis patients and those on parenteral nutrition. Antacid preparations, and those used as phosphate binders in renal patients, are also associated with aluminium toxicity. 59
Copper
Penicillamine is used clinically in the management of Wilson’s disease due to its ability to reduce copper levels by increasing urinary excretion; 60 this accounts for reports of alopecia and loss of taste in patients with rheumatoid arthritis treated with this drug.
Iron
The majority of drugs associated with iron deficiency are those that cause blood loss anaemia. This is a significant side effect of drugs such as the non-steroidal anti-inflammatory drugs and anticoagulants.
There are few reports of iron deficiency induced by drug therapy.
Several drugs sequester iron and are used therapeutically in the management of iron overload in patients who receive repeated transfusions, such as those with thalassaemia or haemophilia. Desferrioxamine is a parenteral therapy, and deferiprone is given orally; both chelate iron and allow it to be excreted in the urine or faeces.
Selenium
Selenium deficiency is rare; symptoms include cardiomyopathy. Sodium valproate reduces plasma selenium levels in patients chronically treated with this drug. 63 Valproate also decreases levels of glutathione peroxidase, a selenium-dependent enzyme, in children experiencing serious adverse reactions to drug therapy. 63,64 Hepatotoxicity and other adverse effects may be related to diminished antioxidant capacity due to depleted selenium and glutathione levels.
Electrolytes
Drug–nutrient interactions with a clinical effect on electrolytes are easily detectable. Plasma and urinary levels are easily monitored.
Calcium
Calcium homeostasis is predominantly controlled by parathyroid function and vitamin D status; however, thyroid function, corticosteroids and growth hormone can also influence the plasma calcium level. These substances exert their effect via the kidney, bone and intestine; however, the kidney plays the predominant role.
Drug-induced hypercalcaemia through oversupplementation is becoming increasingly common as calcium carbonate supplementation is used routinely for the prevention of osteoporosis and as calcium salts are used as phosphate binder in renal disease. 73 Hypercalcaemia is usually asymptomatic at levels below 3.5 mmol/L; diagnosis from clinical symptoms can be difficult as they are insidious and include nausea, vomiting and lethargy (Box 6.13a).

Increased absorption from intestine
• Milk-alkali syndrome (excessive oral calcium salt intake especially carbonate)
• Vitamin D
Increased mobilisation from bone
• Vitamin A and derivatives
• Vitamin D
• Calcipotriol, tacalcitriol
Decreased renal excretion
• Calcium-channel blockers
• Lithium
• Thiazides
• Tamoxifen
• Theophylline
In severe cases hypocalcaemia can cause tetany and cardiac arrest. In most circumstances mild hypocalcaemia causes tingling of the peripheries. Treatment involves discontinuation of the causative drug and supplementation using oral or, if severe, intravenous calcium (Box 6.13b).

• Altered vitamin D metabolism
• Alcohol, anticonvulsants
• Decreased bone mobilisation
• Calcitonin, bisphosphonates, mithramycin
• Increased renal excretion
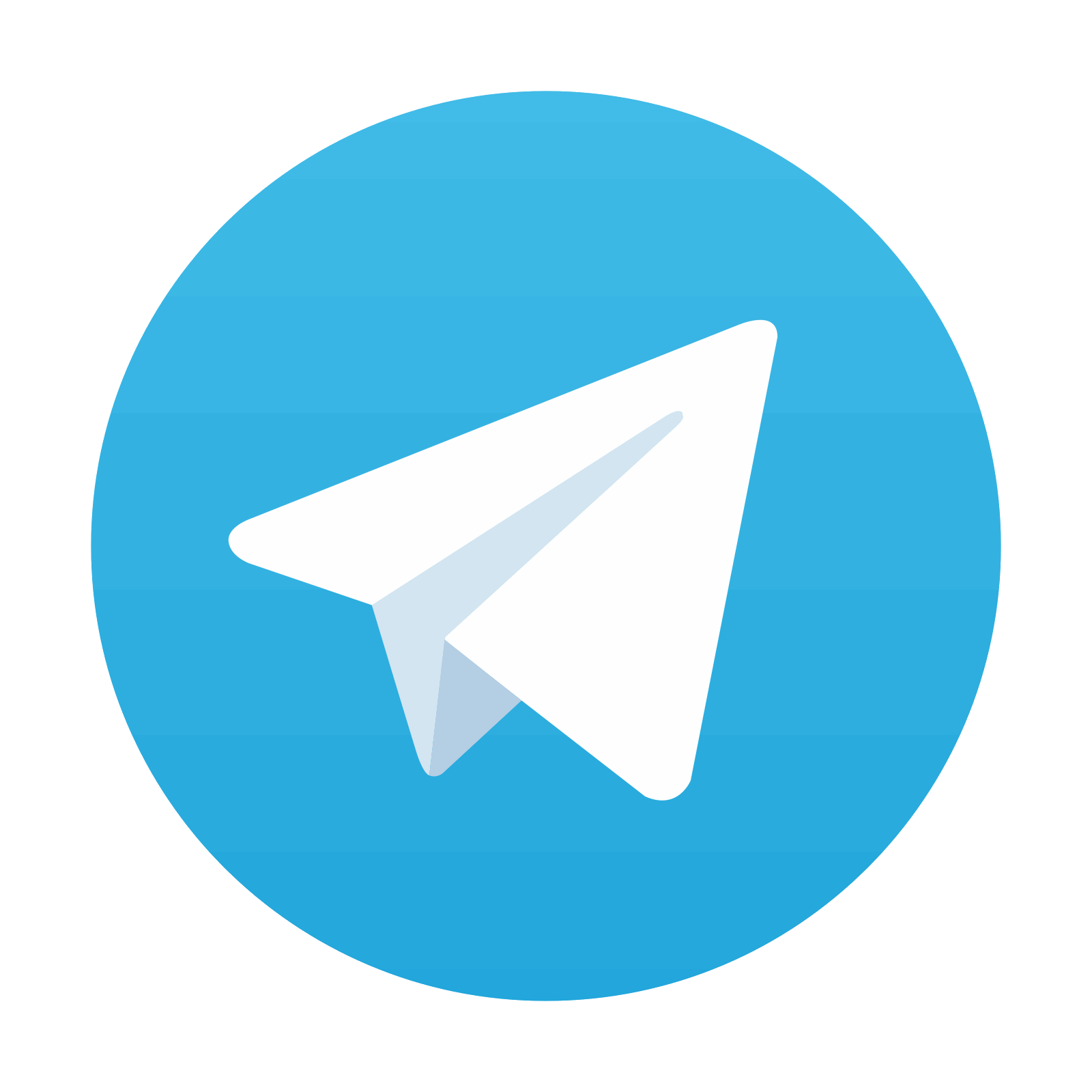
Stay updated, free articles. Join our Telegram channel

Full access? Get Clinical Tree
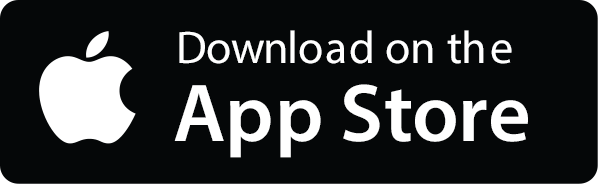
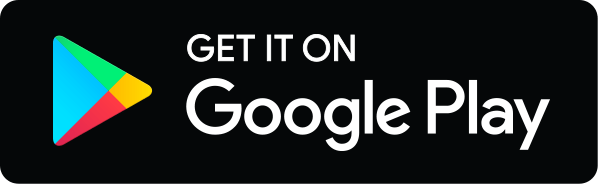
