Fig. 16.1
Various strategies for nanoparticle-based combinational drug delivery systems
Recently, a great deal of interest has been focused on the application of combinational therapies in drug delivery systems [5]. As shown in Fig. 16.1, nanoparticle-based combination strategies include more than just the co-encapsulation of different chemotherapeutic agents in nanoparticles:
- 1.
Combination of chemotherapeutics with other drugs (e.g., chemosensitizing agents, molecular targeting and nucleic acid-based drugs)
- 2.
Combination of chemotherapeutics with other treatments (e.g., radiotherapy, photodynamic therapy, thermotherapy, and immunotherapy)
- 3.
Combination of drug delivery with diagnoses
Due to the different pharmocokinetics of drugs administered in combinatorial regimens, the therapeutic effect of combination chemotherapy is often suboptimal. However, when drugs are co-encapsulated into one nanoparticle, they are simultaneously delivered to the tumor site and modulate tumor cells via different pathways. This allows for the synergistic effect to be maximized, thereby improving target selectivity, overcoming drug resistance, and reducing side effects.
It should be noted that since a lower drug dosage is required for combination therapies [6], their side effects are often reduced as well. When combining drug delivery systems with other therapies, therapeutic efficacy could also be enhanced by this synergistic effect. Drug-loaded nanoparticles themselves also act as sensitizers for other treatments due to their exceptional physical and chemical properties. In this chapter, we will summarize the current state of nanoparticle-based combination strategies for use in gastric cancer treatment. These will include both in vitro and in vivo approaches and will also attempt to highlight their benefits, potential applications, and remaining challenges to their use in clinical translational research.
16.2 Combination of Chemotherapeutics with Other Drugs
Anticancer drugs are often used in combination so as to achieve the maximum synergistic therapeutic effect, itself greater than the sum of each drug’s individual effects. This provides a marked advantage: When only one signal pathway is blocked by a single drug, tumor cells can compensate by either regulating relative protein expression or mutations, resulting in resistance, which is considered as the major reason for many anticancer treatment failures [7]. Alternatively, using two or more drugs that target different cellular pathways can overcome developed chemotherapeutic resistance. However, obtaining the optimal parameters for both the drug cocktail ratio and the treatment sequence is one of the major challenges facing combination drug therapy [6]. This is due in large part to the fact that different drugs have different pharmacokinetic and pharmacodynamic characteristics, biodistribution behavior, and bioavailability when used in combination with each other.
Given this, simultaneous encapsulation of different drugs into one nanoparticle is designed to take advantage of combination therapy as well as the nano-drug delivery system. In addition to using traditional anticancer chemotherapeutics, chemosensitizer agents, molecular targeting drugs, and gene and/or protein therapeutics are often adopted within the sphere of “combinational nanotherapy.” We will now discuss each of these in turn.
16.2.1 Chemosensitizing Agents
Multidrug resistance (MDR) is a major obstacle to the effectiveness of chemotherapy. Tumor cells often increase the efflux of drugs through upregulation of P-glycoprotein (P-gp) or multidrug resistance protein 1 (MRP1), resulting in enhanced chemotherapy resistance. In particular, P-gp has been shown to be overexpressed in many types of cancer, including gastrointestinal, ovarian, and liver cancers [8, 9]. To combat this problem, cyclosporin A, verapamil, tariquidar, and quinacrine (Fig. 16.2) are all capable of inhibiting efflux pump activity. As such, they are the main chemosensitizing agents used to overcome multidrug resistance and enhance the therapy effectiveness [10, 11].


Fig. 16.2
Chemosensitizing agents used in combination with traditional chemotherapeutics
The response rate of 5-fluorouridine (5-FU) in gastric cancer is only about 11% [12]. The chemoresistance to 5-FU in many cancers is associated with the hypermethylation of transcription factor AP-2 epsilon (TFAP2E) [13]. Therefore, it is reasonable to suppose that a combination of DNA methyltransferase inhibitors and 5-FU could promote the sensitivity of many kinds of tumors to chemotherapy, further improving its therapeutic response. To this end, 5-Aza-2’-deoxycytidine (DAC) is one of the most widely investigated demethylating agents and is able to increase the expression of TFAP2E through its demethylating activity [14]. However, the efficacy of DAC in cancer treatment is inhibited by the drug’s inherent instability [15]. When DAC and 5-FU were co-delivered using nanoparticles (NPs-5-FU-DAC) into a single gastric cancer cell, not only was DAC stability enhanced, but targeted cellular uptake was also achieved [16]. Importantly, the synergistic effect of NPs-5-FU-DAC could be detected 72 h after treatment in MKN-45 cells with high TFAP2E methylation levels. In contrast, this synergistic effect was not present in MKN28 cells after any length of incubation [16].
Paclitaxel (PTX) chemoresistance in tumor cells is proportional to total cellular antioxidant capacity. Importantly, PTX cytotoxicity is enhanced when the cell’s endogenous antioxidant capacity is also inhibited by agents that can induce the formation of intracellular reactive oxygen species (ROS) [17]. Tetrandrine (Tet, an alkaloid isolated from Hang-Fang-Chi) is one such compound that can effectively increase the generation of intracellular ROS by enhancing cellular oxidative stress [18]. To illustrate this effect, Li et al. co-encapsulated PTX and Tet in PEG-PCL nanoparticles [19]. The synergistic antitumor effects of co-loaded Tet and PTX (PTX/Tet) were then shown in vitro in a gastric cancer cell line (BGC-823) [11]. Specifically, the viability of BGC-823 cells treated with PTX/Tet nanoparticles was significantly lower than cells treated singly with either nanoparticles containing PTX or Tet. In this study, the combination index (CI) [20] was below 1 when fraction affected (FA, fractional cell growth inhibition) was below 0.75, which indicated a synergistic therapeutic effect for nanoparticles co-loaded with PTX and Tet. Further research investigating the mechanism behind this synergistic antitumor effect when using combinational nanoparticles revealed that it acted to increase ROS production in tumor cells. Moreover, such co-loading also engendered its effect via suppression of the downstream Akt pathway, including Bcl2, Bax, and Caspase 3. Collectively, this resulted in the activation of tumor cells apoptosis [19].
Nanomedicine enables clinicians to precisely modulate the pharmacological properties of multidrug cocktails with cell-specific targeting. Accordingly, delivery of chemotherapeutics in combination with chemosensitizing agents using either passive or active targeting nanoparticles is a powerful approach to overcoming tumor.
16.2.2 Monoclonal Antibody Drugs
Monoclonal antibodies (mAbs) have been harnessed as a type of targeted therapeutic drug and have been primarily in the development for cancer treatment and immunological disorders. For instance, trastuzumab (Herceptin) was approved by the US Food and Drug Administration in combination with 5-fluorouracil and cisplatin for the treatment of HER2-overexpressing metastatic gastric cancer [21]. In the context of nanomedicine, mAbs have typically been used as conjugates on the surface of drug-loaded nanoparticles. In this way, specific tumor targeting can increase drug accumulation to the tumor site as well as resulting in enhanced intracellular uptake [22]. To this end, Li et al. covalently decorated an anti-HER2 Fab onto doxorubicin (Dox)-loaded, thermosensitive, polymeric micelles, which are self-assembled from poly(N-isopropylacrylomide-co-N,N-dimethylacrylamide)118-b-poly(d,l-Lactide)71 (PID118-b-PLA71) [23]. The resulting Fab-conjugated immunomicelles (FCIMs) have dual-targeting properties. Accordingly, it is expected that the tumor accumulation and cellular uptake of Dox would be enhanced by both of these properties—specific recognition and temperature. For the latter, the nanoparticle shell undergoes a hydrophilic-to-hydrophobic transition when the temperature is above the volume phase transition temperature (VPTT). This results in increased antibody-antigen interactions between the nanoparticles and the cell membrane. When incubated with N87 (HER2-overexpressing human gastric cancer cells), the IC50 of FCIM was 9 times lower than that of Doxil. It was also half that of non-targeting micelles. After injection, FCIMs accumulated at the tumor site through passive targeting (EPR effect) and active targeting (HER2). Moreover, a hydrophilic-to-hydrophobic transition was also expected to occur, since solid tumor temperatures are often slightly higher than the VPTT of FCIMs. Based on an in vivo anticancer experiment, the relative tumor volume of the FCIMs group was <2. This is markedly lower than the volumes seen for the non-targeting micelle and Doxil groups 3 and 4, respectively. Thus, the antitumor activity was promoted both in vitro and in vivo by the combined effect of temperature and active targeting.
Similarly, epidermal growth factor receptor (EGFR) is overexpressed in many solid tumors. Synergistic effects have been observed when an EGFR inhibitor is combined with chemotherapeutics, such as PTX, irinotecan, and pemetrexed [24, 25]. Moreover, tumor-penetrating iRGD peptide is often used in drug delivery systems to enhance the accumulation of drug-loaded nanoparticles at the tumor site. This is done by increasing the vascular as well as tissue permeability. To this end, Bian et al. incorporated a recombinant protein—iRGD-fused EGFR single-domain antibody (anti-EGFR-iRGD)—to PTX-loaded silk fibroin nanoparticles (A-PTX-SF-NPs) by way of a carbodiimide-mediated coupling procedure [26]. This combined active tumor-targeting drug delivery system showed superior in vitro antitumor efficacy in a highly expressing EGFR cancer cell line (Hela) than in the low expressing line (MKN-45). These results were also corroborated in vivo.
16.3 Genetic-Based Therapeutics (DNA, siRNA, and miRNA)
Cancer gene therapy is the delivery of therapeutic nucleic acids at the tumor site. This treatment can eliminate tumors by (1) substituting in for mutated genes, (2) downregulating or silencing oncogenic pathways, (3) expressing pro-apoptotic proteins, and/or (4) activating the immune system. Co-delivery of a chemotherapeutic agent and genetic therapeutics against one or more targets could induce cancer cell apoptosis synergistically. Due to their different physicochemical properties, there are great challenges in effectively engineering encapsulation of macromolecular nucleic acids and small-molecule drugs to yield a combined gene and chemotherapy delivery system. Plasmid DNA, siRNA, and miRNA are the most widely used nucleic acid therapeutics that can be delivered using nanoparticles. Among them, micro-RNA (miRNA) is a type of small noncoding RNA molecule that contains about 22 nucleotides. They can act as oncogenes or tumor suppressors during the process of cancer initiation and progression [27]. The advantages of miRNA/small-molecule anticancer drugs combination are numerous, including the promotion of apoptosis and autophagy, downregulating adenosine triphosphate (ATP)-binding cassette (ABC) transporters, suppressing tumor angiogenesis, and reverting the epithelial-to-mesenchymal transition (EMT) [28]. However, owing to their relatively small molecular weight, miRNA are quickly eliminated from circulation and rapidly cleared via renal excretion [28]. In addition, the oligonucleotides are not stable in the acidic environment presented by endosomes/lysosomes and are thus susceptible to degradation before even reaching their cytosolic targets. Therefore, co-delivery of miRNA and anticancer drugs in the same nanovehicles could not only overcome the above miRNA delivery hurdles but also afford a synergistic antitumor effect through simultaneous cargo delivery to the same cancer cells.
To investigate this option, Liu et al. fabricated poly(ethylene glycol)-peptide-poly(ε-caprolactone) (PEG-PEP-PCL) nanoparticles for co-delivery of miR-200c and docetaxel (Doc). With this system, nanoparticles accumulated in the tumor region by EPR effect and the PEG corona was subsequently shed under gelatinase stimuli, resulting in enhanced delivery efficiency [29]. The miR-200c/DOC nanoparticles exhibited a significantly superior antitumor effect than either DOC or DOC-loaded nanoparticles alone. Collectively, their results demonstrated the miR-200c/DOC nanoparticles remarkably inhibited tumor growth in a synergistic manner. This combinational delivery system increased E-cadherin expression levels and decreased CD44 mRNA expression to simultaneously inhibit both cancer stem cells (CSC) and EMT cells. In addition, miR-200c also downregulated TUBB3 expression levels and restored the chemosensitivity of gastric cancer BGC-823 cells to DOC both in vitro and in vivo [17].
Special AT-rich binding protein (SATB1) is a critical regulator of cancer progression that regulates the expression of E-cadherin, ABL1, MMP2, and ERRB2 [30]. It has been reported that inhibiting SATB1 expression can induce the apoptosis of many tumor cells as well as inhibit their proliferation and invasion [31]. To investigate SATB1-focused therapies, Peng et al. fabricated a doxorubicin and SATB1 shRNA co-loaded thermosensitive magnetic cationic liposome. This magnetic-targeting delivery system had a phase transition temperature of 40.8 °C, thereby allowing its use for hyperthermic-response release of therapeutic agents [32]. Additionally, gene delivery efficiency could also be improved by the magnetic targeting properties of the delivery system. Collectively, their results showed that the combined delivery of DOX and SATB1 shRNA not only led to tumor cell apoptosis but also to in vitro as well as in vivo synergistic antitumor effects. Thus, this approach and delivery system resulted in improved tumor inhibition than other previously used formulations.
16.4 Combination of Chemotherapeutics with Other Treatments
In addition to chemotherapy, radiotherapy, photothermal therapy (PTT), and immunotherapy have all been used in the clinic as important cancer treatment options. Standard treatment plans in select European and American guidelines recommend that gastric cancer patients receive concurrent chemo- and radiotherapy (chemoradiotherapy) after surgery [4]. Physical therapies have the advantage of irreversibly destroying tumors. They also offer a synergistic treatment to eradicating cancer by generating a variety of therapeutic outcomes. Overall, the clinical efficacy in cancer patients would benefit from a specific combination of novel drug delivery system and some—or all—of these therapeutic treatments. A deeper discussion of each is provided below:
16.4.1 Radiotherapy
Radiotherapy (RT) is one of the dominant cancer treatment strategies. DNA double-strand breaks and induced tumor cell apoptosis are the key mechanisms through which radiotherapy kills cancer cells. It has been reported that this localized radiation effect can be enhanced by high-Z-containing material. To harness this effect, nanoparticles that are made of noble metals may be useful in increasing the radiation energy absorption due to their unique physical and chemical properties. The mechanisms involved in this synergistic anticancer effect have been attributed to increased ROS, oxidative stress, as well as vascular damage, resulting in apoptosis, necrosis, and autophagy of tumor cells [33, 34].
Many chemotherapeutics are often applied in the clinic with the goal of augmenting radiotherapy tumor effects, termed “radiosensitization.” Docetaxel and concurrent radiotherapy are commonly used for a variety of cancers, including head and neck cancer, non-small cell lung cancer, and gastric cancer [35–37]. However, owing to the nonspecific distribution and side effects caused by docetaxel itself, further insights into effective sensitizer delivery of this combination treatment are needed to improve the therapeutic approach. Doxorubicin-, paclitaxel-, and etanidazole-loaded nanoparticles have been investigated and show better radiosensitizing effects than their free drug formulations [38, 39]. For instance, Cui et al. constructed a docetaxel-loaded nanoparticle (DOC-NPs) using the abovementioned gelatinase-responsive polymer, PEG-PEP-PCL. This was done to selectively enhance the radiation-induced cell death efficacy at the tumor site in addition to reducing the toxicity to normal tissue [40]. When compared with free docetaxel, the nanoparticle formulation exhibited higher drug concentrations and longer time acting in the tumor tissue. Given these results, it is quite possible that nanoparticles have the potential to support the radiation process in fewer doses. Sensitization enhancement ratios of DOC-NPs were 1.09-, 1.18-, and 1.24-fold higher than free docetaxel, respectively, in three gelatinase overexpressing GC cell lines. This is compared to only a 1.02-fold increase in normal, gelatinase-deficient gastric mucosa cells. In a subcutaneous transplant tumor model, DOC-NPs were more effective radiosensitizers than docetaxel and also had a superior ability to delay tumor growth in vivo. Mechanistic research revealed that 79.7 ± 4.9% cells arrested at the G2/M phase when treated with DOC-NPs, which is significantly higher than both the docetaxel and control groups. Since cells in the G2/M phase are more radiosensitive, this might be a major factor behind the enhanced radiosensitization observed in DOC-NPs. Furthermore, the improved radiosensitization efficacy of DOC-NPs was also associated with increased generation of ROS, promotion of cellular apoptosis, and more effective DNA double-strand breaks.
16.4.2 Photothermal Therapy
Tumor cells appear to be more sensitive to heat-induced destruction than normal cells, and treatment approaches have been developed to take advantage of this fact [41]. Photothermal therapy (PTT) refers to treatment utilizing (typically) near-infrared wavelengths of laser-generated heat for the treatment of cancer. Accordingly, tumor tissue can be hyperthermically eradicated through various mechanisms, including protein structural changes and/or tissue carbonization. To this end, several kinds of nanomaterials with the ability to convert light into hyperthermia have been exploited for minimally invasive tumor ablation [42]. Among them, gold-based nanomaterials and carbon materials exhibit unique photothermal properties have been widely investigated as therapeutic agents in photothermal therapy. Furthermore, combinations of photothermal and chemotherapies have also been shown to be a useful approach for cancer treatment [43]. During laser irradiation, the encapsulated drugs can be released under a controllable manner. Importantly, the permeability of tumor cells is increased due to the laser-induced hyperthermia, leading to overall more effective permeation of drugs across cancer cell membranes.
Furthermore, combining tumor imaging and targeted therapy in one nanoparticle holds great promise for the treatment of cancer. This would include all stages of cancer, from diagnosis to evaluation to treatment. Recently, a folic acid (FA)-modified gold nanorods/silica hybrid nanodelivery system (GNR-SiO2-FA) was developed and investigated as a multifunctional nanoprobe for X-ray/CT imaging-guided targeting dual-mode radio- and photothermal therapies [44]. The weight of the conjugated FA on the surface of the prepared nanoparticles was approximately 15.21%, thus ensuring a highly selective targeting ability. The GNR-SiO2-FA nanoparticles also showed enhanced RT and PTT effects in vitro, which depended on the targeted uptake efficiency of gold by MGC-803 gastric cancer cells. Therefore, these nanoparticles are a good candidate for in vivo X-ray/CT imaging due to their strong X-ray attenuation.
16.5 Combination of Therapy and Diagnoses
When combining therapeutic drugs with imaging agents, the integrated nanoparticles are referred to as a “theranostic system.” This multifunctional and integrated system combines different properties, including the tumor targeting, imaging, selective cancer therapy, and response monitoring in an all-in-one system, thereby providing more useful multimodal methods for cancer treatment. Coupling therapeutic effects and contrast properties in combinational delivery system shows great potential in applications such as targeted primary/metastasis tumor diagnosis, simultaneous therapy, and in vivo tracking. Nanoparticles are good alternatives to realize theranostic function, thanks to its particular properties [45, 46]. There are generally two categories of theranostic nanoparticles: (1) the nanoparticles themselves can be detected by imaging modalities, such as gold-, magnetic- and other inorganic nanoparticles [47, 48] and (2) targeted co-delivery of diagnostic and therapeutic agents by nanoparticles. Up till now, a number of various nanoparticle-based theranostic modalities have been reported in the simultaneous diagnosis and treatment of gastric cancer.
16.5.1 Primary Tumor Imaging
Quantum dots (QDs) are a kind of photoluminescence material with strong fluorescent intensity and a tunable emission spectrum. QDs have begun to be widely used in live-cell imaging, immunoassays, and other biological application. For example, Jing et al. synthesized a targeting quantum dot nanoprobe (HER2-RQDs) by conjugating an HER2 monoclonal antibody to the surface of RNase A-associated CdTe quantum dot clusters [49]. The prepared HER2-RQDs were 40 nm in diameter and had an emission peak at 600 nm. Results showed that HER2-RQDs nanoprobes could perform in situ targeted imaging of a gastric cancer-bearing mouse model a mere 6 h after intravenous injection. In addition, this RNase A-loaded nanoprobe could selectively kill HER2 overexpressed gastric cancer MGC803 cells in vitro, inhibit tumor growth, and extend survival time in an in situ gastric cancer-bearing mouse model. This effect was caused by the degradation of total cytoplasmic RNA, inhibition of mRNA translation, and protein synthesis, which ultimately led to the induction of cellular apoptosis [49].
Cancer stem cells are cancer cells that possess the ability of self-renewal and the capacity to initiate tumor formation. They are also considered the responsible members of tumor invasion, metastasis, and resistance to anticancer treatment such as chemotherapy and radiotherapy [50]. Specifically, the CD44+ subpopulation of gastric cancer cells within tumor tissue is endowed with stem cell properties [51]. Given this, CD44 has been taken as a gastric cancer stem cell (GCSC) surface marker. To harness this property of CD44, Liang et al. prepared PEGylated gold nanostars conjugated with CD44v6 monoclonal antibodies (CD44v6-GNS) [52]. These multifunctional nanoprobes were used for photoacoustic, infrared microscopic imaging, as well as photothermal therapy of GCSCs. CD44+ cells were sorted from the MKN-45 GC cell line and then incubated with CD44v6-GNS. After the 24 h incubation, 83% of the CD44v6-GNS was either internalized or bound to CD44+ spherical cells, thereby demonstrating a highly affinity with the stem cell-like CD44+ subset of cells.
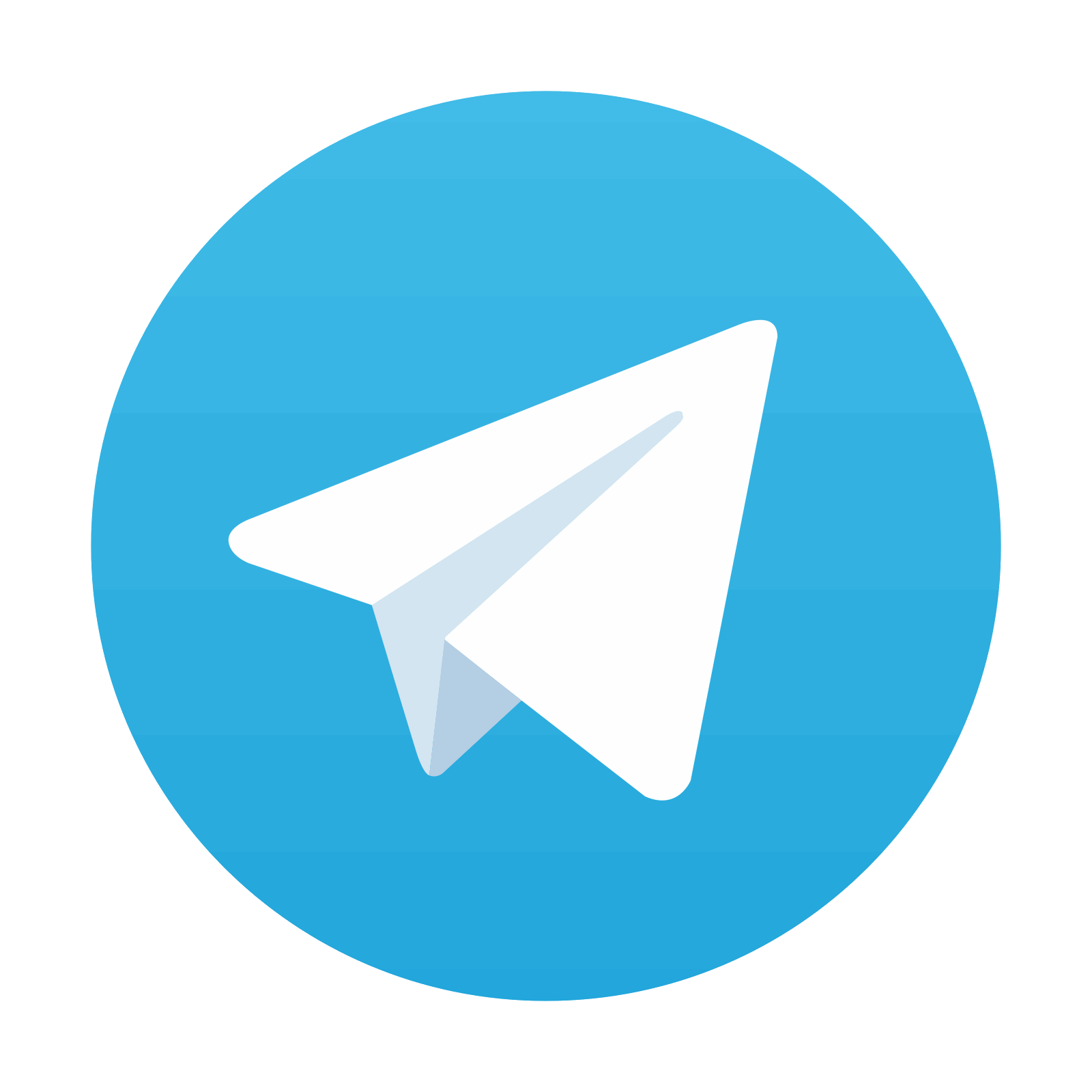
Stay updated, free articles. Join our Telegram channel

Full access? Get Clinical Tree
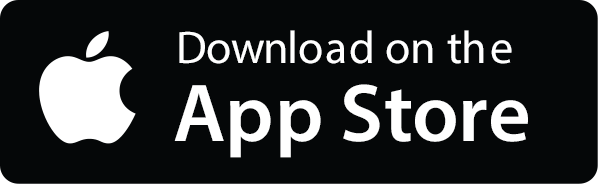
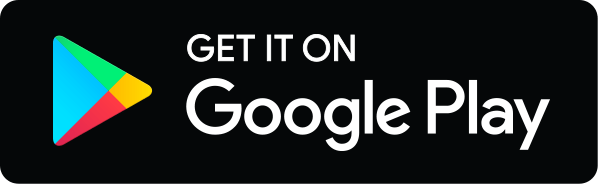