Fig. 7.1
(a) Photograph of a grade II diffuse glioma culture maintained 2 weeks after resection. (b) Detection of cells expressing the mutated form of IDH1 with R132H specific antibody. Co labelling with markers of OPC cells (OliG2 and PDGFRa) are presented
However, no or very limited proliferation was observed and cells could not be amplified. This may be due to the propensity of DLGG cells to undergo senescence in vitro [16]. However, interesting cell lines have been isolated from grade III gliomas. In 2010, two cell lines (BT054 and BT088) with IDH1 mutation and 1p19q deletions were isolated from grade III oligodendrogliomas [17] and in 2012, the BT142 cell line was derived from a grade III oligoastrocytoma. BT142 has aggressive tumor-initiating capacity in orthotopic xenografts [18]. Other groups isolated three cell lines with 1p19q deletions (OligPC40, OligPC49, Hs683) from grade III oligodendrogliomas [19, 20]. Additionally, three less genetically-characterized cells lines were isolated from oligodendrogliomas in 2003 [21].
7.2.2 Animal Models
Although animal models for DLGGs have been developed [22], these models do not include the classical mutations found in these tumors in man (notably IDH1, ATRX mutations and 1p19q codeletions). Therefore, the relevance of these models for studying DLGG dissemination remains to be established. A brain-specific IDH1 R132H conditional knock-in mice using the nestin-Cre promoter was generated in 2012 [23]. However as the nestin promoter is active early during development (E10.5), mice died shortly after birth as the result of massive hemorrhage within the cerebral hemispheres and cerebellum. Analysis confirmed the lack of cerebral development and the presence of large cavities in mutant brains so the relevance of these animals for low grade gliomagenesis was very limited. New transgenic mice enabling cell and time-specific expression of mutated IDH1 are under construction in several labs and these will be very useful tools to model DLGG. In addition to these genetic models, the above-mentioned cell lines grafted intracranially can generate useful models to study OG or AG dissemination even if they are derived from grade III and not grade II tumors. For DLGG tumors which cannot be amplified in vitro, serial grafts in mice could be an alternative to propagate these tumors. This has been demonstrated for a human anaplastic oligodendroglioma carrying mutations for FUBP1, CIC, and IDH1 [24].
7.2.3 Tools
Considering the diversity and the complexity of glioma migration modalities, multiple assays (see, for instance [25–28]) have been used to model high-grade glioma invasion in vitro. In contrast, very few studies aimed at exploring DLGG cell migration in vitro [29–32]. An original model developed by Colin et al. used freshly obtained tumoral explants (500 mm3) which they placed on poly-D-lysine-coated coverslips to analyze glioma cell migration [30]. This study revealed that OG (grade II and III) explants tended to generate few migrating cells. A more sophisticated approach (slice assay) was used by Palfi et al. [32] and de Boüard S [31] who implanted DII-labeled glioma fragments into 400-mm-thick slices of 7 days-old mice brains. In this organotypic assay, invasive glioma cells showed patterns of dissemination and phenotypes similar to those observed in vivo. This assay allowed a quantitative analysis of the invasive potential of gliomas of different types and grades.
As glioma dissemination can occur through migration along nerve fibers, several assays have been designed in vitro to model this phenomenon. One elegant method uses long retinal axons from embryonic chicken which are seeded with glioma cells and which are then visualized by videomicroscopy [27]. Synthetic fibers are also now commonly used. They offer several possibilities of modification regarding their stiffness, their diameter and can be functionalized with extracellular matrix proteins such as laminin [33–36]. The use of such nanofibres has also been considered as a potential therapeutic approach to guide glioma cells toward a cytotoxic hydrogel [36]. Microfluidic chambers and topographic patterns consisting of regular, parallel ridges are also used to study high grade glioma cell migration [37, 38]. Of note, analysis of tumor single-cell migration using high grade glioma patient resections could reveal different cell behavior between patients and predicts outcomes [37]. These different approaches could possibly be used for studying DLGG tumors so as to reveal inter patient heterogeneity. Another technique to be considered as a powerful tool to study DLGG dissemination is intravital imaging. In this approach, fluorescent glioma cells are grafted intracranially and visualized with biphotonic microscopy [39]. Combined with animals expressing fluorescent proteins in specific cell types such as vessels or microglia, this technique can reveal close interactions between glioma cell and other cell types in their environment.
Finally, database and bioinformatics tools are becoming very important and fast growing tools for studying glioma dissemination. For instance, the Ivy Glioblastoma Atlas Project (http://glioblastoma.alleninstitute.org) explored the anatomic and genetic basis of glioblastomas at the cellular and molecular levels. Of special interest, this database contains cellular resolution images of in situ hybridization tissue for several hundred of genes. Gene expression maps within the tumors are provided and this enables identification of genes which are differently expressed at the leading edge or around the vessels. Generalization of such approach in the context of DLGG would certainly provide useful insights on their dissemination.
7.3 2-Dissemination of DLGG: Influence of Location and Genetics
Gliomas have a propensity to be diffusely infiltrative, and it is now known that these migratory cells are refractory to conventional therapy, which may contribute to treatment failure [15, 40]. Investigation of growth patterns is therefore fundamental to understand the cellular interactions and tissue organization of these tumors. Glioma cells appear to invade the normal tissue in three main ways: [2, 41]: (1) migration along the nerve fibers which possibly leads to the perineuronal satellitosis (i.e., clustering of neoplastic cells around neurons) frequently observed in OGs, (2) migration along the vessels, and (3) cellular accumulation and spread through meninges. Data suggest important differences in the mode of invasion between AG and OG cells as a result of differences in genetic alterations, cellular phenotypes, or/and brain locations.
Glioma spatial organization could be divided in three patterns: type I (solid bulk tumor), type II (solid tumor with a diffuse halo of infiltrating cells), and type III (diffuse cloud of cells, no solid bulk) [42]. DLGG mainly comprise type II and III structures. Using an assay where tumors fragment are placed in young rodent brain slices, Palfi et al. [32] found was a clear differences between OGs and AG. OGs tended to be less invasive than astrocytomas and grew as circumscribed tumoral masses. In contrast, astrocytomas were more infiltrative. Further investigations on the correlation between molecular alterations and the in vitro growth patterns established that tumors with 1p19q loss were less invasive in this assay, which is very consistent with the prominent association of this genomic alteration with OGs. They also observed that there was no overt difference between grade II and grade III glioma invasion in this assay. OGs with 1p19q codeletion show a proneural phenotype characterized by a high level of expression of genes which are classically expressed during central nervous system development and in neuronal cells such as alpha-internexin [43]. In addition, electrical activity including action potentials has been described in OG cells [44]. Thus, it is possible that somehow this neuronal-like phenotype may endow the cells with a less invasive capacity compared to astrocytomas.
With regard to localization, DLGG showed a constant relationship with the cortex and a larger volume when they came in contact with the ventricles [45]. OG tumors have a propensity to arise in the gray matter or superficial white matter most commonly in the frontal lobe, but oligodendrogliomas may also arise in other regions of the central nervous system. OG imaging often present as an oval or round sharply marginated mass. Persson et al. [46] reported that 1p/19q-deleted OGs preferentially arose in white matter regions, while low-grade astrocytoma tumors were more frequently associated with the lateral ventricles. Chen et al. [47], reported seven cases located in the grey matter without definite involvement of white matter and 13 cases initially located in the junction of grey and white matter. They propose that glioma invasion may be facilitated by white matter fiber tracts and restricted by grey matter. Destruction of the grey matter could favor access to fibers and dissemination of tumoral cells.
Genetic alterations and probably epigenetic modifications impact the mode of DLGG dissemination. For instance, Jenkinson et al. [48] reported that for OG, tumors with infiltrative growth were more likely to have intact 1p/19q, and those with mixed or solid growth patterns were more likely to have 1p/19q loss. However establishing correlations between the magnetic resonance imaging patterns and the genetic alterations found in DLGG turned out to be challenging and conflicting due to the small number of cases examined and the paucity of genetic data. However, over the last 5 years, one major advance in the field has been obtained by high throughput sequencing of DLGG and the constitution of clinically-annotated database such as REMBRANDT and TCGA (http://betastasis.com/glioma/rembrandt/; http://betastasis.com/glioma/tcga_gbm/). These studies have unraveled many genes and pathways which are altered in DLGG [49–54]. These results have also led to a new WHO histomolecular classification of DLGG published in 2016 [55] where molecular alterations prevails over histological characteristics. Gliomas subtypes such as oligoastrocytomas and GBMO (glioblastoma with oligodendroglioma component) are now mainly defined molecularly [56, 57]. Remarkably, integrated multi-omics analysis of OG revealed previously unrecognized heterogeneity among 1p/19q co-deleted tumors and identified three new subgroups [53]. It is anticipated that such deep characterization of DLGG will lead to new studies correlating MR and genetically-defined DLGG to shed new light on the still-obscure heterogeneous modalities of DLGG dissemination subtypes.
7.4 3-Molecular Components Driving DLGG Migration
In DLGG, oligodendroglioma and astrocytoma cells have different morphology, express different genes and have different dysregulated pathways. This probably account for their different mode of dissemination in the brain. Indeed, it has been found that mutations in p53 and ATRX [alpha-thalassemia/mental retardation syndrome X-linked] are mainly found in astrocytomas [58], whereas genome-wide sequencing has recently revealed the frequent alteration of Fubp1, Tert and Cic genes in OGs [59]. How these alterations impact on cellular migration is totally unknown, but this will certainly be a major subject of investigation in the next decade.
Proteins and proteoglycans involved in migration during brain development appear to be differentially expressed between oligodendroglioma and astrocytoma tumors. For examples Vim, Id4, FABP7, Connexin 43, TrkA/B/C brevican, neurocan, tenascin-C, versican, Chi3L1, CD44, Gap43 are preferentially expressed in AG [60–65] whereas the phosphatase receptor rPTPbeta/zeta, Olig2 and PDGFRa are preferentially expressed by OG [64, 66]. It is likely that these different entities could give tumoral cells different ability to migrate along the vascular network, the nerve fibers or the subpial space.
Of special interest is CD44, a receptor for hyaluronic acid (HA, also called hyaluronan). Hyaluronic acid is an anionic, non-sulfated glycosaminoglycan widely distributed in neural tissues. Radotra and collaborators [67, 68] used a Matrigel system to study glioma cell migration and found that adding HA to the Matrigel increased migration in a dose-dependent manner. They further investigated the mechanisms by which glioma cells migrate in HA-enriched Matrigel and found that CD44 was involved in this process, as blocking CD44 with a specific antibody led to a significant reduction in glioma cell migration. This study was done in vitro using an anaplastic astrocytoma-derived cell line. Nevertheless, given the preferential migration of OG cells along HA-rich white matter fibers, it seems likely that CD44s and HA play a role on OG migration in vivo as well. As HA inhibits oligodendrocyte maturation [69], it can be hypothesized that HA participates in the dissemination of OG cells by maintaining them in an undifferentiated precursor-like state which is prone to migration.
In 2006, McDonald et al. used OG tumor sections from 177 patients to identify the genetic consequences of the 1p36 deletion observed in 60–80% of OGs [70]. They identified SHREW1 (also known as AJAP1) as the unique gene present in the deleted region and found that OG cells from 30 patients showed significantly reduced SHREW1 expression as compared to normal tissue. SHREW1 is a membrane protein involved in adherent junctions. The authors demonstrated that overexpression of SHREW1 in a GBM cell line (U251) inhibit cell adhesion and migration, and thus propose that loss of its expression in OD may promote cell dissemination.
Gap junctions and connexin protein are increasingly regarded as important players in high grade glioma dissemination and this probably applies in DLGG [71]. One remarkable discovery is the presence, in low and high grade astrocytomas, of a network of microtubes interconnecting tumoral cells [65]. These microtubes can be as long as 500 μm and in a mice model for astrocytoma can reach the contralateral hemisphere. Cells are interconnected by this microtubule network via gap junctions which express connexin 43. The growth cone associated protein Gap-43 appears to have a central role in the formation of these microtubules. Very interestingly, these protrusions infiltrate the normal brain at the invasive front and are used for movement of cell nuclei. This microtube network has been detected in low grade astrocytomas with an IDH1 R132H mutation whereas no such network was found in 1p19q deleted OG. This highlights the difference in astrocytomas and oligodendrogliomas and their mode of dissemination.
Another class of protein which are gaining interest in DLGG migration are galectins. Galectins is a family of mammalian lectins with specificity to beta-galactosides. They are involved in growth-regulatory mechanisms and cell adhesion of several cancers. In the normal brain, Galectin-3 maintains cell motility of precursor cells from the subventricular zone to the olfactory bulb [72]. Galectin-1 is preferentially expressed at the tumor margin and promotes glioblastoma cell invasion [73]. Very interestingly, Galectin-1 is also involved in glioma immune escape [74] by suppressing NK immune surveillance [75]. Using the Hs683 1p19q deleted oligodendroglioma cell line, Le Mercier et al. [76] demonstrated that loss of Galectin 1 reduce chemoresistance, neoangiogenesis, and migration of these cells suggesting that in addition to GBM, this lectin could also have an important role in DLGG dissemination.
The use of high throughput techniques combined with functional assays will certainly identify new proteins involved in DLGG migration. For instance, based on the observation that OGs with 1p19q are less aggressive than their non-deleted counterparts, Rostomily et al. [77] undertook the comparative proteomics of OGs with or without 1p19q deletion. They found that about 10% of the differentially expressed genes were involved in invasion/migration. The list of genes presented in their Appendix II could be used to decipher the differential migratory properties of OG with or without 1p19q deletion and open new lines of investigations on the invasive properties of OG. In 2015, high throughput sequencing in OG with 1p19q deletion [54], led to the identification of mutations in SASH3 and GDI1 genes. The protein encoded by SASH3 gene contains a Src homology-3 (SH3) domain and a sterile alpha motif (SAM), both of which are found in proteins involved in cell signaling and this protein may function as a signaling adapter protein. GDI1 genes codes for a Rab GDP dissociation inhibitor alpha which is expressed in neural tissues. Paradoxically, overexpression of SASH3 and GDI1 wild-type proteins in oligodendroglioma cell lines enhanced their migration in vitro compared to mutated proteins.
In addition to the endogenous mechanisms pertaining to tumoral cells in DLGG migration, one must also consider the influence of the extracellular matrix [ECM] and the interactions with neighboring tumoral and non tumoral cells. In fact, cells at the invasive edge in high grade and DLGG upregulate metalloproteases (MMP2, MT1-MMP) to degrade or modify their environment [78]. These cells also express proteins such as angiopoietin-2 which can impair formation of the blood-brain barrier [79] and secrete their own extracellular matrix protein such as laminin [78]. The mutated form of IDH1 found in the majority of DLGG may also have a direct impact on the structure of ECM as D-2-hydroxyglutarate produced by the mutant IDH1 leads to reduction of prolyl-hydroxylation of collagen, causing defect in its maturation and basement membrane aberrations [23]. Other component of the ECM such as CSPGs (glycosylated chondroitin sulfate proteoglycans) are also to be considered as central actors regulating dissemination. Silver et al. found that noninvasive lesions are associated with a rich matrix enriched in CSPGs, whereas these are essentially absent from diffusely infiltrating tumors [80]. CSPGs may induce migration of resident reactive astrocytes out of the tumor mass which results in encapsulation of noninvasive lesions, acting as a barrier to directly inhibit tumor dissemination.
With regards to DLGG cell interactions with non-tumor cells, very little is known. In the grey matter, OG cells closely interact with neurons and accumulate to generate the so-called peri-neuronal satellitosis [41, 81]. In high grade gliomas, neuron activity has been shown to release the synaptic protein neuroligin-3 (NLGN3) which acts as a mitogen for glioma cells [82]. If a similar signaling occurs in low grade OG remains to be fully demonstrated. In the white matter, OG are entrapped in a fibrillary background composed of axons and fibrillary reactive gliosis [81] suggesting close interactions between reactive astrocytes and glioma cells. Indeed, in a mouse model for glioma [83], Sin et al., reported that reducing coupling of reactive astrocytes with glioma cells by knocking down the gap junction protein Cx43, resulted in reduction of glioma spreading into the brain parenchyma [83]. Using a vitro model and the C6 glioma mouse cell line, Oliveira et al., also reported that heterocellular glioma cells/astrocytes interactions through gap junctions support tumor cell migration [84]. These results indicated an important role for reactive astrocyte in the formation of an invasive niche which could possibly manipulated to reduce migration.
One last interesting possibility to consider is that DLGG cell could change phenotype depending on their environment and can adopt a new gene expression profile adequate for migration in a given environment. Indeed, activation of BMP signaling induces astrocytic differentiation of clinically-derived oligodendroglioma cells [19]. Conversely, in a mouse model for glioma, PDGF autocrine stimulation could dedifferentiates astrocytes to induce oligodendrogliomas and oligoastrocytomas [85]. In addition, it appears that oncogenic signaling is dominant to cell of origin and can dictate astrocytic or oligodendroglial tumor development from oligodendrocyte precursor cells [61]. For instance, activation of K-Ras and Akt in oligodendrocyte progenitor cells led to astrocytic tumors when combined with p19(Arf) loss [61]. This interconversion between astrocytoma and oligodendroglioma might occur as a result of a switch between master transcription factors governing astrocyte or oligodendrocyte fate. Indeed, the mutual antagonism between Sox10 and NFIA transcription factors which regulates the diversification of glial lineages during development, may also control the formation of astrocytoma vs oligodendroglioma subtypes [86]. Further analysis is necessary to ascertain whether this phenotypic interconversion occurs in patient tumors.
7.5 4-Insights from Oligodendrocyte Progenitor Migration
Given the suspicion that OG tumors are derived from the tumorigenesis of oligodendrocyte precursor cells (OPCs) [87], it is likely that OGs share some common molecular properties with OPCs. So as to propose new potential targets worthy of investigation as potential OG migration drivers, we will list and review the main pathways known to direct OPC migration and propose how these data could be used and applied to research on OG invasion.
Several categories of molecules are involved in OPC migration: (a) long-distance signaling proteins play a chemoattractive or chemorepelling effect on OPCs, thus controlling their direction; (b) proteins of the extracellular matrix provide a beneficial or repulsive substrate to migrating cells; and (c) structural proteins expressed by the migrating OPC provide a favorable cytoskeleton configuration. A comprehensive review on OPC migration provides substantial information on the signals involved in this process [88, 89]. Of these signals, we present here the ones that are likely to be shared by OGs.
The first obvious signal involved in OPC migration and likely to play a role on DLGG survival is PDGF. In OPCs, PDGF-induced migration is mediated by Cdk5, involving the phosphorylation of the non-receptor tyrosine kinase Fyn [90]. Cdk5 phosphorylates the WAVE2 protein which forms a multiprotein signal transduction complex binding to receptor kinases and actin, with an effect on cell shape and motility [91]. Overexpression of a WAVE2 construct where the site of phosphorylation has been mutated leads to reduction of PDGF mediated migration of OPC. WAVE2 has been shown to be involved in cellular migration [92] and plays a pivotal role in melanoma cell migration [93] and other cancer metastasis [94]. Given the role of WAVE2 on OPC migration [90] and on several types of cancers, WAVE2 is likely to be involved in DLGG invasion. Comparative proteomics between OGs with or without 1p19q deletion identified WAVE3 as a protein significantly more expressed in tumor cells with deletion than in cells without deletion [77]. Proteins of the WAVE (or WASF) family could thus supply informative clues on the migratory properties of DLGG.
The best characterized chemotactic signals driving OPC migration during development are semaphorins (3F and 3A) and netrin-1 [95]. Indeed, semaphorin 3A was shown to have a repulsive effect on OPCs in explants, whereas semaphorin 3F and netrin-1 attract OPCs. One study investigated the expression of seven class-3 semaphorins, SEMA4D, VEGF, and the NRP1 and NRP2 receptors in 38 adult glial tumors and showed that Sema3A expression was similarly expressed in low-grade and high-grade gliomas [96]. Sem3A has been shown to promote dispersal of GBM cells [97] and it would be interesting to investigate its role on DLGG migration. Interestingly, Nasarre et al. showed that Sem3A can have either a chemorepellent or a chemoattractant effect on GBM cell lines depending on the present partners [98], thus making it a prime target for glioma migration investigations.
One important point to emphasize is the specific pattern of migration adopted by OPCs depending on their localization in the brain. Indeed, using heterotypic quail/chick xenografts, Olivier et al. [99] demonstrated that OPCs transplanted from the rostral to caudal brain domain and vice versa changed their migratory properties. Their neat approach identified several routes of migration followed by OPCs depending on their original location and emphasized the key role of environmental cues upon OPC migration. Based on these data, it is easily predictable that gliomas arising from OPC tumorigenesis might comply with the same rules. This hypothesis is consistent with a recent study on GBM [100] showing that SVZ derived or cortex-derived GBM cells (GBM6 and GBM9, respectively) present different invasive properties. It is thus necessary, when studying DLGG dissemination, to report precisely their site of appearance, so as to be able in the future to predict the more likely pattern of diffusion of a tumor based on its initial localization in the brain.
Finally, in 2016, Tsai et al. [101] demonstrated that during development, OPCs use the vasculature as a physical substrate for migration. They associate with the vascular endothelium surface of nearby blood vessels and can even jump between vessels. The Wnt-Cxcr4 pathways was involved in these OPC-endothelial interactions. Considering that glioma cells often reactivate developmental programs to grow and disseminate, it is likely that a similar process occurs in oligodendrogliomas.
7.6 Issues To Be Addressed
There are several issues that would deserve further explorations in order to clarify the means by which DLGG cells disseminate into the brain: (1) Identifying infiltrated tumoral cells in the normal tissue: Diffuse low-grade tumoral cells exhibit only a mild nuclear atypia so they are difficult to distinguishing from normal cells in infiltrated tissues. So until recently it was very difficult to delimit the actual dissemination of tumoral cells which probably extend far beyond MRI-defined abnormalities. Indeed, using serial stereotactic biopsies and MIB1/Olig2 staining, Pallud et al. [102] found that tumoral cells can be observed at sites up to at least 15 mm beyond brain imaging aberrations. A major advance in the field is the development of an antibody recognizing the mutated form of the IDH1 enzyme [R132H] which is commonly found in DLGGs [103]. This antibody allows the precise detection of isolated tumoral cells by immunohistochemistry. Using this tool, Sahm et al. analyzed the infiltration pattern of three anaplastic OGs and considering the widespread dispersion of tumoral cells concluded that gliomas should be addressed not as a focal but as a systemic disease [104]. Similar analysis performed on DLGGs would reveal the actual extends of the tumor. (2) Distinguishing migratory versus non-migratory glioma cells: The tumoral tissue is composed of cells in different states (for instance, proliferative vs. quiescent cells) and it is likely that in DLGG, only a fraction of the cells are actually invading the normal tissue. It will be very useful to uncover reliable markers to identify and purify these cells in order to analyze precisely the molecular components and active pathways involved in this process. Whereas such markers are starting to be uncovered for high-grade gliomas, thanks to differential microdissection of the tumor core versus invasive margin [105], such tools are not yet available for DLGGs. In addition, it appears that DLGGs exhibit two main patterns of spatial organizations: solid tumor plus a diffuse halo of infiltrating cells or dispersed cloud of cells with no solid bulk [42, 81]. The molecular and cellular mechanisms behind this heterogeneity of patterns are not documented and warrant further exploration. (3) Differential migration in the white and gray matters: Brain white and gray matters differ in many aspects (cellular composition, vascular density, organization, biochemical composition) so it is expected that glioma cells invade these two components in a different way. In particular, the white matter contains myelin which constitutes the insulating sheath for neurons but which is also a non-permissive substrate for neurite growth, attachment, and spreading of a lot of cell types. Interestingly, Amberger et al. [106] found that low-grade astrocytomas appeared to be strongly sensitive to inhibitors present in myelin, whereas OGs were able to spread and migrate on this substrate. Using the C6 glioma line which showed no spreading inhibition on myelin, this group went on to show that these cells expressed the MMP14 metalloprotease (MT1-MMP). This protease degraded the Nogo protein, a major inhibitor present in myelin [107] and allowed glioma cells to spread on this substrate [108]. It is anticipated that such a mechanism might operate for low-grade OG cell dissemination, but this remains to be formerly demonstrated. In the normal brain, oligodendrocytes are closely associated with nerve fibers, whereas astrocytes interact closely with vessels to form the blood–brain barrier. Therefore, spreading of OGs along nerve fibers and of astrocytoma cells along vessels could represent a pathological counterpart of the normal situation.
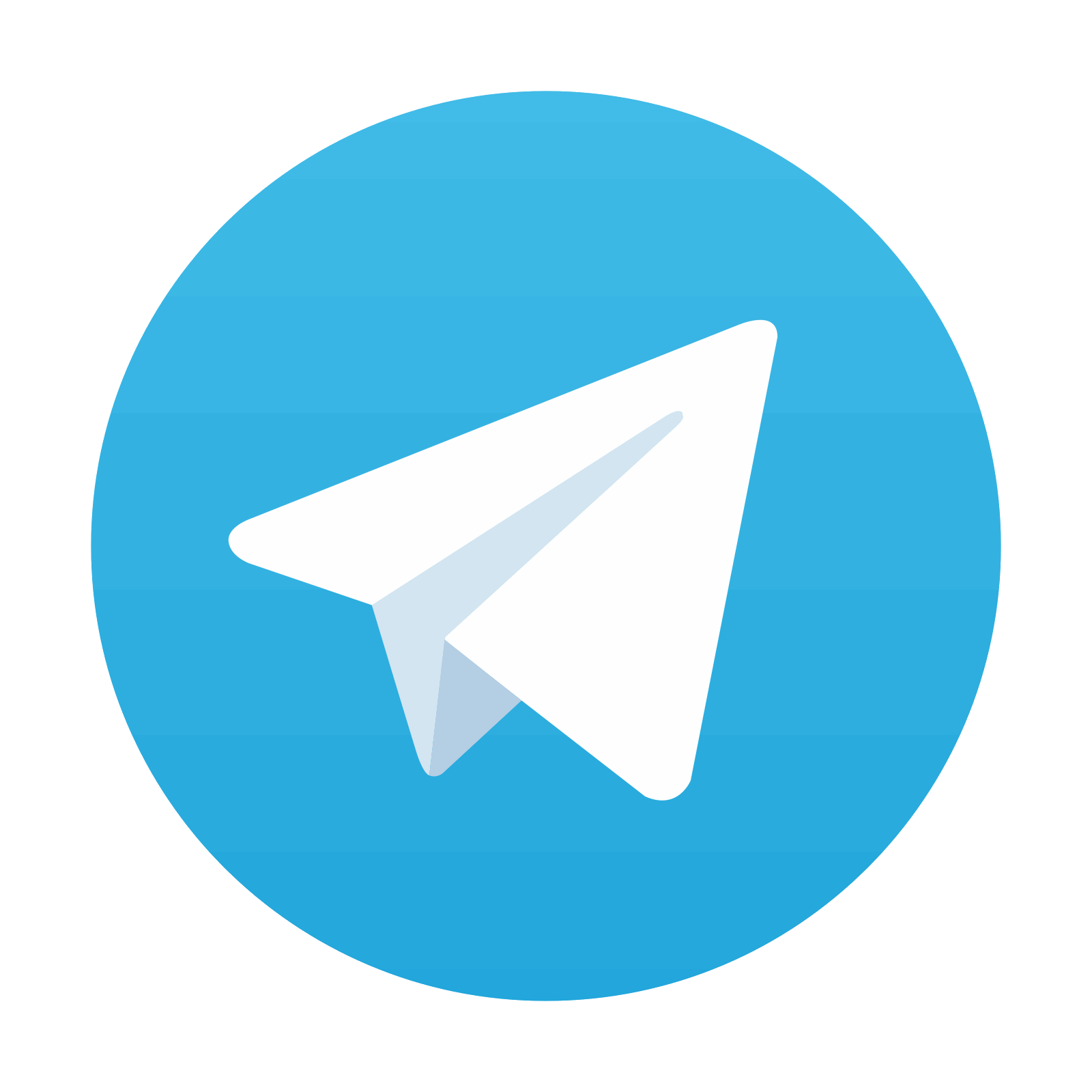
Stay updated, free articles. Join our Telegram channel

Full access? Get Clinical Tree
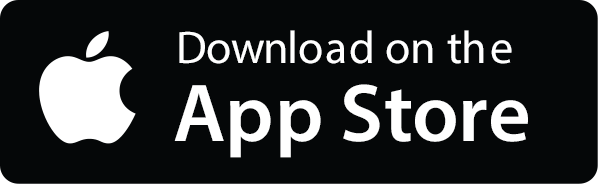
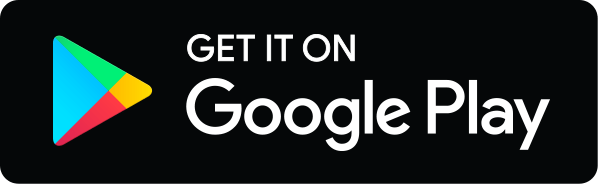