Roman Romero-Ortuno, K. Jane Wilson, Joanna L. Hampton
Disorders of the Autonomic Nervous System
This chapter focuses on the consequences of aging on autonomic cardiovascular control. The neurobiology of aging and the effects of aging on gastrointestinal and urinary tract function are detailed in other sections in this book.
The chapter first provides a brief summary of autonomic pathways involved in cardiovascular control, and the methods used to assess their function. The chapter then reviews the effect of aging on the different components involved in autonomic cardiovascular control, including alterations in afferent and efferent function and in end-organ responsiveness. The integrated effect of these changes on the response of older people to daily stresses of life (i.e., response to upright posture and to food ingestion) is discussed. The final part of the chapter outlines primary and secondary disorders of the autonomic nervous system that are clinically relevant in older people and reviews the clinical management of orthostatic hypotension.
Basic Concepts of Autonomic Physiology
Autonomic Pathways
Autonomic regulation depends on three main components. Afferent fibers continuously sense changes in blood pressure (baroreceptors), blood oxygenation, and other chemical signals (chemoreceptors), pain (sensory afferents), and cortical stimulation. These signals are integrated in brainstem centers that ultimately modulate sympathetic and parasympathetic outflows, which are transmitted to target organs via efferent fibers. The baroreflex provides an example of these pathways (Figure 63-1). This is a redundant system, with input from multiple independent afferent pathways that ensure maintenance of cardiovascular regulation even after partial damage.1,2 The afferent limb of this reflex includes pressure-sensitive receptors located in the walls of cardiopulmonary veins, the right atrium, and within almost every large artery of the neck and thorax, but particularly within the carotid sinus and aortic arch. Stimulated by stretch, these low- and high-pressure baroreceptors monitor venous and arterial pressures, respectively, and relay that information to brainstem centers. Information from the venous and aortic arch baroreceptors is carried centrally via fibers that course within the vagus nerve (X cranial nerve). Carotid sinus baroreceptor nerve activity is relayed centrally first via the carotid sinus (Hering) nerve, then through the glossopharyngeal nerve (IX cranial nerve) before arriving at the same brainstem centers.

Afferent fibers from these multiple baroreceptors have their first synapse in the nucleus tractus solitarius of the medulla oblongata.3 This nucleus inhibits sympathetic tone and is crucial to baroreflex function. Destroying it (e.g., by experimental lesion4 or neurologic damage)5 leads to loss of baroreflex function, resulting in episodes of hypertension and tachycardia.6 In addition to the afferent input arising from the baroreceptors, the nucleus tractus solitarius also receives modulating input from many other cardiovascular brain centers, such as the area postrema.7 The nucleus provides excitatory inputs to the caudal ventrolateral medulla, which in turn inhibits the rostral ventrolateral medulla,8,9 where the pacemaker neurons that produce sympathetic tone are believed to be located.10 Rostral ventrolateral medulla neurons project to the preganglionic sympathetic neurons in the intermediolateral column of the spinal cord that send fibers outside the central nervous system. Parasympathetic activity is also modulated by the nucleus tractus solitarius, through projections to preganglionic parasympathetic neurons in the nucleus ambiguus and the motor nucleus of the vagus (see Figure 63-1).
The importance of autonomic mechanisms in the regulation of blood pressure is most evident when they fail. Damage of baroreflex afferents (e.g., as a consequence of radiation or surgery), leads to labile blood pressure that is very difficult to control.6 At the other extreme, degeneration of central or efferent structures, as seen in patients with primary autonomic failure, leads to disabling orthostatic hypotension.11,12 In the most severe cases, patients are only able to stand for a few seconds before profound orthostatic hypotension causes loss of consciousness (i.e., orthostatic syncope). These disorders are described later in this chapter.
Methods Used to Test Autonomic Function
Posture (Orthostatic) Test
Perhaps the most informative and simplest autonomic evaluation is the posture test. The patient’s blood pressure and heart rate are measured after 5 to 10 minutes in the supine position and repeated after the subject stands motionless for 3 to 5 minutes. There is value in repeating measurements at each of these time points, as one single measurement may or may not be informative. Ideally, beat-to-beat plethysmography is the most informative, but if this is not available, then repetitive manual measurements of both heart rate and blood pressure will enable a clearer assessment and lead to a more accurate diagnosis, especially in cases where the autonomic failure is mild to moderate rather than severe.13
Virtually all patients with severe autonomic failure will have an immediate fall in blood pressure on standing. Other autonomic conditions associated with delayed orthostatic hypotension may require a 30-minute stand test to make the diagnosis,14 but they are usually not associated with widespread autonomic neuropathy. Older patients may find it extremely tiring to stand for 30 minutes unassisted and a passive tilt test, during which data is recorded continuously and the patient is supported physically, will increase the tolerance of completing the test.
Heart rate is crucial in interpreting blood pressure changes. Patients with severe autonomic failure characteristically have no or little (about 10 to 15 beats/min) increase in heart rate despite profound orthostatic hypotension. A greater increase in heart rate usually indicates that other conditions (e.g., volume depletion or medications) are contributing to orthostatic hypotension.
Orthostatic Hemodynamic Assessment With Sphygmomanometer
Routinely, clinicians use the auscultatory or oscillometric method with a sphygmomanometer. In 1996, a consensus committee of the American Autonomic Society and the American Academy of Neurology defined orthostatic hypotension as a drop of at least 20 mm Hg in systolic blood pressure and/or 10 mm Hg in diastolic blood pressure within the first 3 minutes of orthostasis.15 This definition was intended primarily for clinical situations where orthostatic blood pressure changes are measured with a sphygmomanometer or automatic oscillometric blood pressure monitors.16,17
Orthostatic Hemodynamic Assessment With Beat-to-Beat Monitoring
The introduction of new noninvasive beat-to-beat finger arterial blood pressure monitors led to concerns that the consensus definition of orthostatic hypotension, originally intended for the sphygmomanometer,15 may lack clinical relevance when applied to beat-to-beat data.18,19 Some orthostatic hypotension definitions based on continuous hemodynamic assessment include initial orthostatic hypotension and can only be measured with continuous noninvasive monitoring.20 Initial orthostatic hypotension is defined as a transient blood pressure decrease, within 15 seconds after standing, of more than 40 mm Hg in systolic blood pressure and/or more than 20 mm Hg in diastolic blood pressure, with symptoms of cerebral hypoperfusion.21
The advantage of using continuous noninvasive measurement of finger arterial blood pressure over the conventional sphygmomanometer or the oscillometric measurement method is that the former provides continuous patterns of response that can be visualized and analyzed, not only for blood pressure but also for derived hemodynamic parameters. As a result, three different orthostatic response patterns have been recognized and studied in adults and older people, based on the morphology of the blood pressure recovery after standing20,22,23; these three patterns are the quick recovery pattern, which is the normal physiologic response; the slow recovery pattern, which is known to occur in pathologic conditions such as carotid sinus denervation24 or carotid sinus hypersensitivity25–27; and the failure to recover pattern, which is classically observed in patients with autonomic failure.28 These three “morphologic” patterns of orthostatic blood pressure response have been characterized in research studies using a cluster analysis technique.29–31
Noninvasive Autonomic Tests
Heart rate responses to deep breathing (i.e., respiratory sinus arrhythmia) and to the Valsalva maneuver are simple yet informative autonomic tests. They require real-time monitoring of the heart rate. Respiratory sinus arrhythmia is assessed during controlled breathing at a rate of six deep breaths per minute (Figure 63-2). The expiratory/inspiratory (E/I) ratio is calculated by dividing the longest R-R interval by the shorted R-R in inspiration. This E/I ratio decreases progressively with age. Subjects younger than 40 years usually have a ratio less than 1.2 (Figure 63-3). Having the subject blow against a 40 mm Hg pressure for 12 seconds induces a Valsalva maneuver. A 5- to 10-mL syringe can be used as a mouthpiece, which is connected to a sphygmomanometer to monitor pressure. A small leak should be introduced into the system to ensure the subject uses thoracic effort. The increase in intrathoracic pressure produces a transient fall in blood pressure with narrowing of the pulse pressure during phase II (strain), whereas the blood pressure overshoots above baseline values during phase IV (after release) (Figure 63-4). In autonomic failure, the blood pressure continues to fall during phase II and the normal overshoot is absent during phase IV. Thus, appropriate evaluation of the Valsalva response requires continuous recording of blood pressure, which can be accomplished noninvasively with finger plethysmography (Finapres, Portapres, or Task Force Monitor) or tonometry of the radial artery (Colin). Even if the blood pressure cannot be monitored, heart rate responses are useful. The blood pressure changes described previously produce reciprocal baroreflex-mediated changes in the heart rate. The heart rate increases during the hypotensive phase II of the Valsalva maneuver and decreases during the blood pressure overshoot of phase IV. Valsalva ratio is defined as the maximum heart rate during the maneuver divided by the lowest heart rate obtained within 30 seconds of the peak heart rate.32 As with the E/I ratio, the Valsalva ratio decreases with age and results should be interpreted accordingly (see Figure 63-3). Normal reference ranges have been suggested.33
Spectral Analysis of Heart Rate and Blood Pressure
Blood pressure and heart rate are kept within a relatively narrow range because of autonomic baroreflex mechanisms. Within this narrow range, however, blood pressure shows substantial variability. Most of this variability is not random but, rather, follows natural rhythmic patterns, which can be studied using spectral analysis techniques. Importantly, these patterns are modulated by the respiratory frequency. In particular, respiration frequency influences heart rate variability, and this interaction is under baroreflex control via the vagus nerves. The “respiratory peak” of heart rate variability, evident in the high-frequency spectrum, can be used to assess cardiac parasympathetic function.
Respiration also modulates blood pressure, but this is mediated through mechanical events and does not reflect autonomic mechanisms and is not affected by autonomic blockade.34 In contrast, blood pressure exhibits a lower frequency rhythm (Mayer waves). This is mediated in part by sympathetic modulation of vascular tone. There is substantial interindividual variability in the spectral analysis of heart rate and blood pressure, making these methods less suitable for the diagnosis of individual patients with less than severe autonomic impairment. Nonetheless, population studies have shown that impaired heart rate variability as shown by spectral analysis of heart rate is an independent predictor of mortality in patients after myocardial infarction35 and in patients with diabetes mellitus. Independent of disease status, heart rate variability has been found to be impaired in people with physical frailty.36
Assessment of Baroreflex Function
Several methods can be used to quantify the changes in heart rate (or R-R interval) produced by unit change of blood pressure. These methods require the simultaneous monitoring of blood pressure and heart rate. Baroreflex function can be assessed by measuring the reciprocal changes in blood pressure and heart rate that occur spontaneously, or during the phase IV of the Valsalva maneuver. Blood pressure can be increased with phenylephrine or decreased with nitroprusside and the gain of the baroreflex expressed as the change in R-R interval per unit of blood pressure (expressed in msec/mm Hg) during the linear portion of this relationship. Each of these methods provides slightly different normative values of baroreflex gain. It is important to note that changes in blood pressure affect all baroreflex afferents, including carotid sinus and aortic high-pressure receptors and low-pressure receptors located in the venous circulation. The carotid sinus reflex can be selectively investigated by producing positive and negative pressure to the neck, to simulate decreases and increases in intracarotid pressure, respectively. All of these methods rely on instantaneous changes in heart rate, which depend exclusively on the parasympathetic limb of the baroreflex. The sympathetic limb of the baroreflex can be assessed by relating changes in blood pressure to reciprocal changes in muscle sympathetic nerve activity (MSNA).
Biochemical Assessment of Sympathetic Function
Plasma norepinephrine levels provide a useful measure of sympathetic activity. It is particularly useful when measuring acute changes to standard stimuli. For example, upright posture doubles the plasma level in norepinephrine. Patients with autonomic impairment have a blunted response. Basal norepinephrine, however, varies depending on the underlying pathology. It is low in patients with primary autonomic failure and normal or slightly decreased in patients with multiple system atrophy (MSA) (see later discussion). In contrast, patients with volume depletion have an enhanced norepinephrine response to upright posture.
Estimation of Norepinephrine Spillover
Despite the usefulness of plasma norepinephrine measurements, it is noteworthy that only a small percentage of the norepinephrine released by noradrenergic nerves actually reaches the circulation. Most of it is taken back into nerve terminals by the norepinephrine transporter (i.e., reuptake) or is metabolized. Norepinephrine clearance can be measured by infusing a known amount of titrated norepinephrine. During steady-state infusion, it is assumed that clearance of titrated norepinephrine reflects clearance of endogenous norepinephrine. Once clearance is calculated, the norepinephrine appearance rate into the circulation (spillover) can be estimated. A comprehensive review of the advantages and limitations of this technique is beyond the scope of this chapter and can be found elsewhere.37
Muscle Sympathetic Nerve Activity
Nerve activity can be recorded directly by introducing a recording electrode into an accessible peripheral nerve. Afferent and efferent fibers can be recorded using this technique. Sympathetic efferent activity can be selectively recorded by careful placement of the electrode. The peroneal nerve at the level of the knee is commonly used to measure postganglionic sympathetic nerve activity. Although there is considerable interindividual variability in MSNA, baseline recordings expressed as sympathetic bursts per minute are highly reproducible in a single individual between different recording sites and when measured on different occasions. MSNA effectively monitors central sympathetic outflow and is tightly modulated by the baroreflex. Stimuli that increase blood pressure by activating central sympathetic outflow produce an increase in MSNA. Conversely, stimuli that increase blood pressure directly, such as an injection of phenylephrine, will produce baroreflex-modulated suppression of MSNA. This recording is also exquisitely sensitive to sympathetic withdrawal. For example, sympathetic activity disappears during neurogenic syncope.38
Effects of Aging on Components of Autonomic Cardiovascular Control
Baroreflex Function
There is a progressive decline in baroreflex sensitivity with aging39 as a result of both vascular and neural deficits. Cardiovagal baroreflex gain (i.e., the reciprocal heart rate changes produced by changes in arterial pressure) declines with age, but the ability of the cardiopulmonary baroreflex to inhibit sympathetic nerve traffic has been reported to be well preserved with age in healthy adults. Hence vagal, but not sympathetic, baroreflex gains vary inversely with individuals’ age and their baseline arterial pressures.33 There is no correlation between sympathetic and vagal baroreflex gains.40
Cardiac Parasympathetic Function
Cardiac vagal innervation decreases with age, as clearly shown by a progressive reduction in respiratory sinus arrhythmia (see Figure 63-3). Experimental evidence suggests that long-term physical activity attenuates the decline in cardiovagal baroreflex gain by maintaining neural vagal control,41 but among older adults, the level of fitness does not prevent the decrease in cardiac vagal function, suggesting that age-related decline in cardiac vagal function cannot be completely prevented by physical activity.42
Systemic Sympathetic Function
MSNA increases progressively with age, likely because of increased central nervous system drive (Figure 63-5). Sympathetic nerve traffic increases in a region-specific manner; outflow to skeletal muscle and the gut increases but decreases to the kidney.43 The increase in central sympathetic outflow results in higher levels of plasma norepinephrine with age, but reduced norepinephrine clearance appears to play a role as well.44,45 It is suggested that age-related elevations in whole-body and abdominal adiposity can explain why basal MSNA increases with age in healthy humans.46 The relation between body fat and MSNA is observed in both young and older populations.47 Tanaka, Davy, and Seals46 showed that although body mass index (BMI) was similar in groups of younger and older subjects, both total body fatness and abdominal adiposity were greater in the older subjects and were directly related to baseline levels of MSNA. Data indicate that circulating concentrations of leptin are related to both adiposity and MSNA.48 Thus, age-associated elevations in total and abdominal adiposity may be linked to increases in MSNA, at least in part, via elevations in leptin levels. In contrast to sympathetic neuronal activity, adrenaline secretion from the adrenal medulla is greatly reduced with age, and adrenaline release in response to acute stress is attenuated in older men. Plasma adrenaline concentration remains normal, however, because of reduced plasma clearance.
End-Organ Responsiveness
The number of β-adrenergic receptors in lymphocytes remains steady in older age,49 and there are higher neurotransmitter levels. However, β-adrenergic responses to norepinephrine are blunted with progressive aging, probably because of β-adrenergic receptor downregulation in response to higher circulating levels of norepinephrine, a defect in G protein receptor complexes and reduced adenyl cyclase activity.50,51 Depressed β1 responses lead to impaired cardioacceleration and reduced cardiac contractility. Reduced β2 responses produce increased vascular tone because α1 vasoconstriction ability remains unchanged. The combination of age-related vascular stiffening and depressed β-adrenergic function results in reduced arterial baroreflex sensitivity52 in older subjects.53,54 Blood pressure in older subjects is sustained by increased peripheral vascular tone, despite depressed cardioacceleration. Because of a higher reliance on vascular resistance, dehydration and vasodilator medications pose a high risk for hypotension and syncope in older subjects. Vasovagal syncope is commonly seen in older adults whose symptoms include presyncope, falls, or syncope.55 Postjunctional α-mediated vasoconstriction is also impaired in older adults.56
Vascular Changes
Aging stiffens blood vessels57 and alters vasomotor function. In older patients, coronary vasodilation capacity is reduced because of reduced nitric oxide release by the senescent endothelium. Conversely, endothelin release by the endothelium is increased in older people, promoting vasoconstriction.58 These alterations increase susceptibility to myocardial ischemia, particularly during increased demand stresses such as tachyarrhythmias,59 and can also impair cerebral autoregulation, increasing susceptibility to syncope. Other age-dependent cardiovascular alterations that can increase predisposition to syncope are increased left ventricular afterload and myocyte hypertrophy. These alterations lead to impaired diastolic filling and chronic ischemia that may predispose patients to cardiac arrhythmias and decrease ventricular volume that may manifest as syncope. Decreased preload volume, precipitated by vasodilators, dehydration, or blood pooling can dramatically reduce cardiac output and precipitate syncope. Susceptibility to atrial fibrillation increases with age because of reductions in pacemaker cells, progressive fibrosis of the cardiac conduction system, and concomitant cardiovascular diseases that alter atrial morphology. In older patients, impaired diastolic filling and a reduction of up to 50% in cardiac output can develop during atrial fibrillation and lead to syncope and unexplained falls.60
Neuroendocrine Changes
Plasma renin and aldosterone levels fall with age,61,62 and atrial natriuretic peptide increases fivefold to ninefold.63 The vasopressin response to hypotension may also be reduced.64 These changes make sodium and water conservation less effective and intravascular volume depletion more frequent, thus increasing the tendency for syncope. In addition, many older people have an impaired thirst response to increases in osmolality and do not consume sufficient fluids to prevent hypovolemia.
Effects of Aging on Autonomic Response to Stress
The most frequent autonomic stress is the cardiovascular adaptation to upright posture and other physiologically induced changes in intravascular volume. Vascular and neurogenic dysfunction and a host of medications can cause orthostatic hypotension in older patients. In the Cardiovascular Health study,65 the prevalence of orthostatic hypotension was 18% in subjects aged 65 years or older, although only 2% reported dizziness on standing. There was a modest association with systolic hypertension when supine, with carotid stenosis greater than 50%, and with the use of oral hypoglycemic agents; a weak association with the use of β-blockers; and no association with other antihypertensive drugs. In other reports, however, as expected, the use of antihypertensive medications was significantly related to postural hypotension in older people,66 and discontinuing antihypertensive medications led to an improvement of orthostatic hypotension.67
Orthostatic Hypotension
Older people have impaired defenses against the fluid shifts that normally accompany upright posture; therefore, they have a lower threshold for developing symptomatic orthostatic hypotension compared with younger people. A variety of symptoms develop with a reduction in blood flow to the brain. Typically, patients complain of visual disturbances (e.g., blurring, tunneling, or darkening of vision), dizziness, light-headedness, giddiness, feeling faint, and a dull neck and shoulder ache (coat hanger pain). When orthostatic hypotension is pronounced and cerebral blood flow decreases below a critical level (approximately 25 mL/min per 100 g), syncope (i.e., loss of consciousness) may occur.
A decrease in baroreceptor sensitivity is probably involved in the mild, frequent postural hypotension seen in older people. One study, for example, showed a diminished response to tilt (a baroreceptor-mediated response) but not to non–baroreceptor-mediated stimuli, such as the cold pressor test or isometric exercise.68 The reduced baroreceptor response in older people (when compared with younger controls) was seen in both hypertensive and normotensive subjects. Insults that would be compensated for in the young may induce symptomatic hypotension in older people. For example, drug-induced orthostatic hypotension is the cause of recurrent dizzy spells or syncope in 12% to 15% of older patients and should always be suspected.69 Diuretics, calcium antagonists, angiotensin-converting enzyme (ACE) inhibitors, and nitrates are frequently prescribed for older patients for the management of hypertension, congestive heart failure, or ischemic heart disease. Other pharmacologic agents frequently associated with orthostatic hypotension include phenothiazines, antidepressants (including selective serotonin reuptake inhibitors), treatments for Parkinson disease (PD), antipsychotic agents, sedatives, and narcotics. It is also important to note that the more types of these drugs an older person is taking, the greater is the risk of orthostatic hypotension.67
Similarly, prolonged bed rest is a common complication of ill health in older people and an important cause of cardiovascular deconditioning. Several mechanisms contribute to decreased orthostatic tolerance and syncope after prolonged bed rest.70 Bed rest reduces extracellular fluid volume, and the skeletal muscle wasting impairs the lower limb muscle pump that usually facilitates venous return in the upright posture. Under normal conditions, important mechanical adjustments to counteract orthostatic pooling of the blood are the muscle and respiratory pumps. Skeletal muscle tone has critical bearing on the volume of blood displaced into the legs when standing. Because intramuscular pressure is decreased after prolonged bed rest, venous pooling occurs and venous return to the heart is easily compromised in the standing posture. Thus, skeletal muscle atrophy caused by prolonged bed rest should be considered as a primary or aggravating factor in any patient with symptoms of light-headedness on standing or documented orthostatic hypotension. If the problem persists after adequate measures are taken, a pathologic impairment of autonomic function should be considered. The occurrence of orthostatic hypotension in older people is predictive of mortality.71 A study of 3522 Japanese-American men, aged 71 to 93 years, found that orthostatic hypotension, defined as a decrease in systolic blood pressure by 20 mm Hg or a decrease in diastolic blood pressure by 10 mm Hg, was present in 7%, increased with age, and was an independent risk factor for all cause mortality.71
Frailty in older adults is characterized by cumulative decline in many physiologic systems leading to decreased physiologic reserve and vulnerability to stressors.72 As such, studies have suggested that orthostatic hypotension could be a marker of frailty. A study showed that the physical frailty phenotype was associated with impaired orthostatic heart rate response and a tendency toward lower systolic blood pressure recoverability during the 30-second period after standing.73 Using a frailty index approach, Rockwood, Howlett, and Rockwood suggested that orthostatic hypotension might be a marker of the system dysregulation seen in frailty.74
Postprandial and Heat-Induced Hypotension
Postprandial hypotension and heat-induced hypotension are common causes of falls and syncope in older people.75,76 In normal subjects, eating, especially carbohydrates, leads to splanchnic vasodilation and hot weather produces cutaneous vasodilation, but there is little change in arterial pressure because of a compensatory increase in sympathetic vasoconstrictor outflow. In older people, as in patients with autonomic failure, both eating and hot weather significantly lower blood pressure (even in the supine position) because these patients cannot compensate for the vasodilation with an appropriate increase in sympathetic outflow.77,78 Among older residents of nursing homes, 24% to 36% have a 20 mm Hg or greater fall in systolic blood pressure within 75 minutes after eating a meal.79 In patients with autonomic failure, postprandial hypotension occurs within 30 minutes of meal ingestion, lasts about 1.5 to 2 hours, and can be profound; blood pressure can fall 50 to 70 mm Hg. Thus, it is important to consider the timing of meals when measuring blood pressure in these patients. The initial syncopal episode in patients with chronic autonomic failure is frequently triggered by postprandial hypotension.
Disorders of the Autonomic Nervous System in Older People
Neurally Mediated Syncopal Syndromes
The most frequent cause of hypotension and syncope in otherwise normal subjects is neurally mediated syncope, also known as reflex syncope. This umbrella term encompasses carotid sinus hypersensitivity, vasovagal syncope, and a number of benign syncopal syndromes, which are triggered by specific actions such as swallowing, voiding, defecating, laughing, weightlifting, and brass instrument playing.13 Syncope triggered by anxiety or emotion (e.g., fainting at the sight of blood) also fits into this category, as does syncope after vigorous exercise. It is very important, however, to distinguish between syncope during exercise and syncope after exercise, as the former should not be considered a reflex syncope (and needs full cardiac workup). Neurocardiogenic syncope is generally, although not exclusively, observed in patients with no evidence of structural heart disease. Prodromal symptoms include dizziness, blurred vision, nausea, an increasingly hot feeling, and diaphoresis. This syncope results from acute vasodilation and bradycardia.
Neurally mediated syncope is an acute hemodynamic reaction produced by a sudden change in autonomic nervous system activity.80 The normal pattern of autonomic outflow that maintains blood pressure in the standing position (increased sympathetic and decreased parasympathetic activity) is acutely reversed. Parasympathetic outflow to the sinus node of the heart increases, producing bradycardia, while sympathetic outflow to blood vessels is reduced, resulting in profound vasodilation.
Classic neurally mediated syncopal syndromes are triggered after compression of carotid baroreceptors in the neck (carotid sinus syncope),38 following rapid emptying of a distended bladder (micturition syncope)81 or distention of the gastrointestinal tract.82 Glossopharyngeal or trigeminal neuralgia can induce syncope by a similar mechanism,83,84 but both are rare. In several clinical types of neurally mediated syncope, the trigger locus is easily identified, but frequently neurally mediated syncope occurs with no obvious trigger. Although in these cases the source of abnormal afferent signals was believed to be sensory receptors in the heart (i.e., neurocardiogenic or “ventricular” syncope),85,86 neurally mediated syncope has recently been induced in patients with heart transplants in whom the ventricle is likely to be denervated.87 Perhaps sensory receptors in heart transplant patients are in the arterial tree rather than the ventricle. Similarly, the threshold to trigger neurally mediated syncope can be lowered by a reduction in cardiac preload caused by reduced intravascular volume or excessive venous pooling. Intravascular volume depletion is common in older people because conservation of sodium and water is less effective, renin and aldosterone levels fall, atrial natriuretic peptide increases, and the vasopressin response to hypotension may be reduced. Moreover, many older adults have an impaired thirst response to increases in osmolality and are prone to hypovolemia, particularly during febrile illnesses. Excessive venous pooling occurs postprandially in the splanchnic circulation, in the skin during exposure to heat, and in the lower limbs because of muscle atrophy when standing after prolonged periods of bed rest, significantly increasing susceptibility to syncope.
Despite the diverse trigger mechanisms of these different types of neurally mediated syncope, the efferent reflex response is remarkably similar. There is an increase in parasympathetic efferent activity to the sinus node, producing bradycardia or even a few seconds of sinus arrest, and a decrease in sympathetic activity responsible, at least in part, for the fall in blood pressure. Bradycardia is not the only or even the main cause of hypotension because neither atropine nor a ventricular pacemaker (both of which prevent bradycardia) is able to prevent hypotension and syncope. Blood pressure falls mainly because of vasodilation. The mechanisms responsible for vasodilation are not completely understood. Studies using microneurography and measurements of circulating norepinephrine have shown that sympathetic efferent activity decreases.88–90 Sympathetic “withdrawal,” however, seems an incomplete explanation for profound vasodilation. Norepinephrine fails to increase, but vasopressin, endothelin-1, and angiotensin II vasoconstrictor peptides, important to maintain blood pressure (which should partially compensate for the fall in sympathetic activity), increase normally during neurally mediated syncope.89 To explain the profound fall in blood pressure, β-mediated vasodilation induced by a rise in adrenaline has been postulated.91 Nitric oxide–mediated vasodilation due to a rise in cholinergic activity may be involved.80 In summary, current understanding of neurally mediated syncope shows inappropriate reduction in sympathetic nerve activity and norepinephrine release. There is an appropriate increase in epinephrine, angiotensin II, vasopressin, and endothelin release, and preliminary evidence suggests that nitric oxide synthesis is activated.
Baroreflex Failure
The most common cause of baroreflex failure is iatrogenic damage during neck surgery or radiation therapy of neural structures that carry afferent input from the baroreceptors. Atheroma can also cause baroreceptor failure.92 Neurologic disorders involving the nucleus tractus solitarius, where these afferents have their first synapse, can also produce baroreflex failure. In a few cases, the underlying cause is not found. Baroreflex function is impaired in essential hypertension and can be transmitted as a genetic trait.93 It is not clear if these cases of baroreflex failure of unknown cause represent the extreme of the spectrum of baroreflex impairment in essential hypertension. Ketch and coworkers describe “four faces of baroreflex failure”: hypertensive crisis, volatile hypertension, orthostatic tachycardia, and malignant vagotonia,94 the most common of which is volatile hypertension or severe labile hypertension. Wide fluctuations of blood pressure are observed, with systolic blood pressure ranging from 50 to 280 mm Hg. Other symptoms that may be observed include hypotension, headache, diaphoresis, and emotional instability. The hypertensive crises are accompanied by tachycardia and are to the result of sympathetic surges, as documented by notable increases in plasma norepinephrine. Treatment with sympatholytics may provide some benefit, attenuating these surges of hypertension and tachycardia, but adequate blood pressure regulation is seldom achieved in the absence of functional baroreflexes. Of interest is that virtually all reported cases are due to bilateral lesions, whereas unilateral lesions are usually clinically silent. This clinical observation underscores the redundancy of the baroreflex system and its importance in cardiovascular regulation.
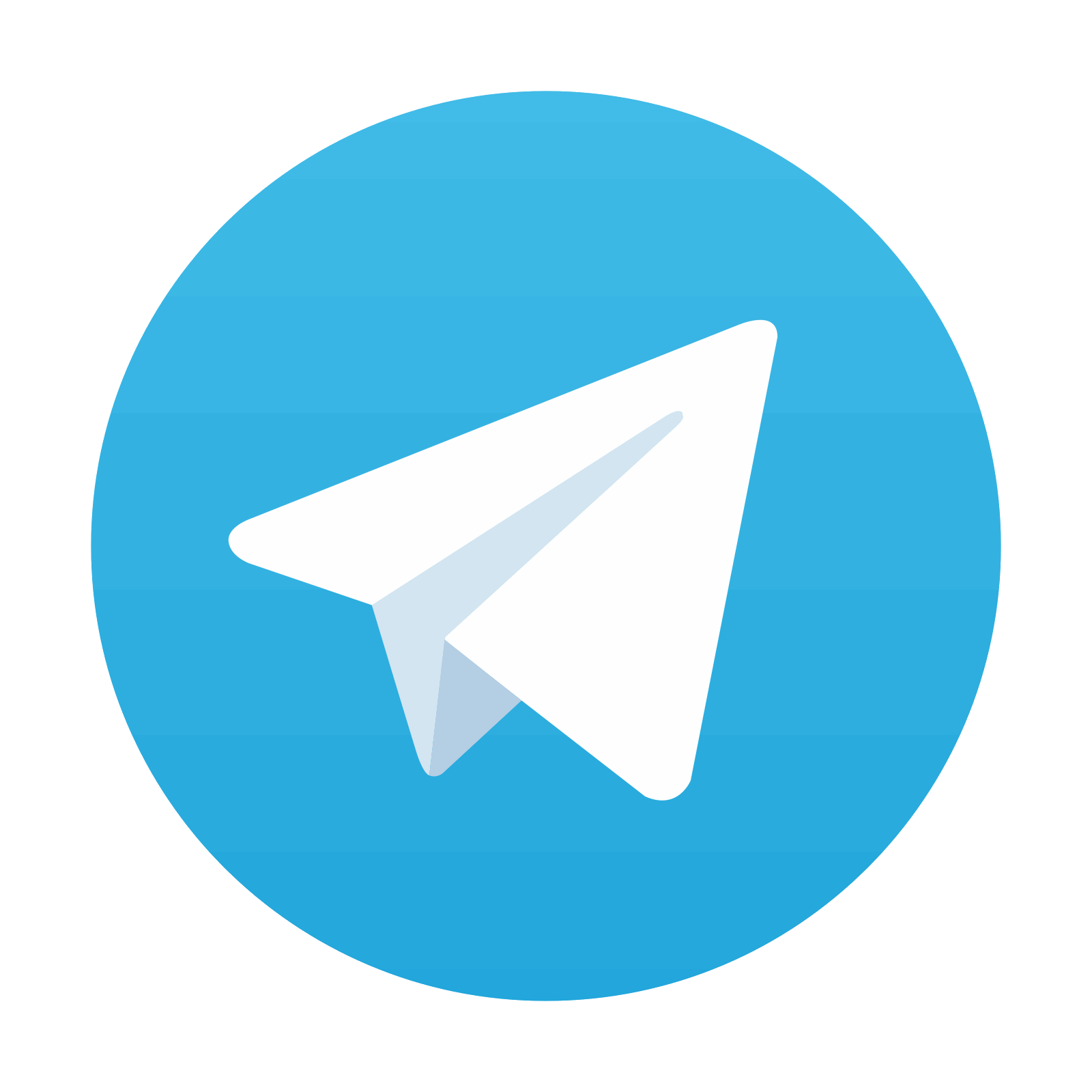
Stay updated, free articles. Join our Telegram channel

Full access? Get Clinical Tree
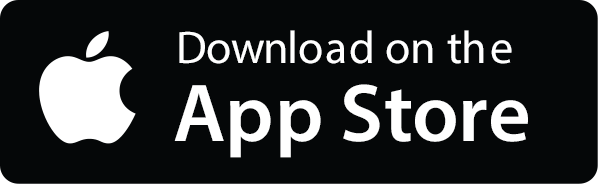
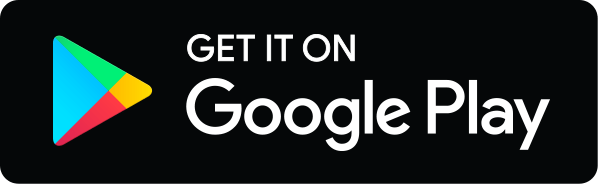