13
DIAGNOSTIC IMAGING
13.1 RADIOGRAPHY AND FLUOROSCOPY
13.1 RADIOGRAPHY AND FLUOROSCOPY
Why is there a significant difference in image contrast between a general radiograph and a portal image (or film) of the same patient and same anatomy?
Question 2
What is the general range of kVps used for diagnostic imaging?
Question 3
For all diagnostic X-ray imaging, where does the depth of maximum dose (Dmax) reside?
Question 4
Assuming no other changes, as the image receptor is moved farther away from the patient, what happens to the anatomy in the image?
The greater contrast in the general radiographic image results from lower energy photons (kilovoltage) having a larger fraction of photoelectric interactions. This is especially apparent when bone is imaged. The photoelectric effect is proportional to (Z/E)3; therefore, high-Z and low-E result in very large absorption, hence increased contrast. In comparison, portal images in radiation therapy use the megavoltage (MV) energy treatment beam. For MV energy photons, Compton scattering is the dominant interaction with tissue. Compton interactions are dependent on electron density, which varies minimally between tissue types.
Answer 2
The typical operational range of kVps for diagnostic imaging is between 60 and 120 kVp. The kVp used for a given exam will depend on the anatomy to be imaged and the desired image quality.
Answer 3
Dmax resides at the skin surface for diagnostic energy X-ray beams; there is essentially no buildup depth for kilovoltage X-rays.
Answer 4
As the image receptor is moved away from the patient with no other changes in geometry, the anatomy in the image will be magnified. The magnification factor will be equal to the source to image distance/source to object distance (SID/SOD).
In an automatic exposure control (AEC) or automatic brightness control (ABC) image acquisition, moving the patient as close to the image receptor as possible will have what effect on patient skin entrance dose?
Question 6
For X-ray-based angiography, what are the two primary factors contributing to blurring of the vessels in the image?
Question 7
What are the patient dose limits for radiographic and/or fluoroscopic procedures?
Question 8
For all modern fluoroscopic units (manufactured after 2006), the cumulative air kinetic energy released per unit mass (kerma) is required to be displayed. Is the cumulative air kerma equivalent to the patient skin dose?
Reducing the distance between the patient and the image receptor for an AEC or ABC image acquisition will reduce the patient skin entrance dose. Dose reduction will be proportional to (SSDinitial/SSDfinal)2 (inverse square law), where SSD is source to skin distance.
Answer 6
The two primary factors contributing to blurring of vessels in an image are the focal spot size and the location of the anatomy in relation to the image receptor (greater distance results in greater geometric blur).
Answer 7
There are no regulatory limits for patient radiation dose for radiographic or fluoroscopic procedures. However, the Joint Commission does define a sentinel event for fluoroscopic procedures resulting in a cumulative skin dose of 15 Gy, or more, to a single field.
Answer 8
The displayed air kerma on fluoroscopes is an air kerma calculated to a reference plane. The reported air kerma is the sum of all radiation output, in all projections. Therefore, this value does not directly relate to a skin dose since it does not account for the geometric dose distribution. Additionally, the reported air kerma can substantially deviate from the skin dose because it does not account for the accuracy of the ion chamber (which may deviate by ±35%), attenuation factors of the table and pad (which can exceed 30%), tissue back scatter factors, and f-factors based on photon energy.
For imaging performed with image intensifier-based fluoroscopes, where in the image field of view should the anatomy of interest be placed?
Question 10
What is the conversion sequence of the image signal, starting with the X-rays exiting the patient and ending with the output phosphor, in an image intensifier?
Question 11
For fluoroscopic imaging, when the field of view (FOV) is changed by increasing the magnification, how is the air kinetic energy released per unit mass (kerma) rate at a fixed location affected?
Question 12
State-of-the-art fluoroscopic systems use dynamic copper (Cu) filtration. What is the purpose of these filters and how do they affect image quality?
All image intensifiers have some geometric distortion that typically increases near the periphery of the image; therefore, the anatomy of interest should be placed in the center of the image.
Answer 10
The X-rays leave the patient and pass through the grid, where some are absorbed. They then reach the input phosphor, where the X-rays are converted to light. Directly behind the input phosphor, the light photons are converted to electrons by the photocathode. The electrons are accelerated across the evacuated tube and strike the output phosphor, converting the electrons back to light.
Answer 11
When the FOV is decreased (by increased magnification) the air kerma rate will increase. For image intensifier-based systems, the increase will be proportional to the square of the ratio of beam areas (A2/A1)2. For digital image receptors, generally the air kerma rate increases; however, the increase is not necessarily related to the beam area.
Answer 12
The Cu filters are used to harden the X-ray beam, thereby reducing the skin entrance dose. However, as an X-ray beam is hardened there is a subsequent loss of contrast in the image.
Assuming that other factors do not change; does reducing the X-ray field size by collimation reduce the air kerma (kinetic energy released per unit mass) rate, the air-kerma-area-product rate (AKAP) or both?
Question 14
If the air-kerma-area-product rate (AKAP; kerma: kinetic energy released per unit mass) is measured free-in-air 50 cm from an X-ray source and then again at 100 cm from the same X-ray source, how does this measurement change?
Question 15
Which image receptor has the highest potential spatial resolution: an image intensifier, a digital flat panel detector, or traditional film?
13.2 MRI
Question 1
In T2-weighted MRI of the prostate, a tumor is visibly darker than the surrounding normal tissue. Why?
The AKAP rate will be reduced, but the air kerma rate will remain unchanged. Air kerma is normalized to mass (just like dose), so the field size will not affect the air kerma. However, AKAP is the product of the air kerma and the field size, therefore a change in the beam area will have a direct effect.
Answer 14
AKAP is not impacted by the plane of measurement; therefore, the values will be identical. Even though the air kerma decreases by the inverse square law with distance from a radiation source, the area of the beam increases by the same proportion.
Answer 15
The spatial resolution capabilities for film are much greater than for any other current image receptor. The primary benefit of using a digital detector is the ability it offers the operator to adjust the window and level, providing dynamic contrast adjustment.
Answer 1
The T2 relaxation time of the tumor tissue is less than that of the surrounding tissue. This shortened T2 results in greater transverse dephasing yielding decreased signal and a darker appearance in the image.
Question 3
What effect does increasing the strength of the slice-select gradient have on the image thickness?
Question 4
If MRI is used to scan an anatomic region with a high proportion of fat, what common methods of fat suppression (pulse sequences) are available?
Question 5
How do most contrast agents used in MRI work?
The atomic mass number (A) must be odd (an odd number of either protons or neutrons) for there to be a net magnetic moment of the nucleus, which is required for MRI to function.
Answer 3
For a fixed radio frequency (RF) bandwidth, the image slice thickness will decrease when the slice-select gradient strength is increased. This improves spatial resolution and reduces volume averaging in the slice thickness dimension.
Answer 4
The use of an inversion recovery pulse sequence such as a short tau inversion recovery (STIR) with an appropriate time of inversion (TI), Dixon technique (phase suppression), and chemical shift saturation (spectral suppression) are common techniques that suppress fat signal.
Answer 5
T1 relaxation agents are the primary type of contrast agent used in MRI. These agents speed up the spin-lattice energy transfer (reducing the local T1 relaxation time), thereby increasing the T1 signal.
How do contrast agents used in MRI differ from contrast agents used in computed tomography (CT) or other X-ray-based imaging modalities?
Question 7
What is a typical in-plane spatial resolution and an estimate of the highest resolution possible for clinically approved MRI units?
Question 8
What are a few of the benefits of increasing the primary magnetic field strength (B0) (eg, why use 3T or greater versus 1.5T)?
Question 9
What are a few of the detriments of increasing the primary magnetic field strengths?
Contrast agents used in X-ray-based imaging modalities have a higher atomic number than the surrounding tissue and therefore absorb photons via photoelectric effect, making the contrast agent directly visible in the image. Most MRI contrast agents affect the local T1 relaxation times of the tissue environment in which they are present. This enhances image contrast on a T1-weighted protocol; the contrast agents are not directly visible in the image, but their effects are.
Answer 7
Typical in-plane resolution is similar to that of CT, with a pixel size between 0.5 and 1.0 mm. With a small field of view (FOV) and a high-strength gradient on a surface coil, pixel size can be as small as 0.1 mm.
Answer 8
Assuming all other parameters are unchanged, a larger B0 provides a greater signal to noise ratio (SNR). If an SNR equivalent to that seen with a lower field strength is acceptable; the image slice thickness can be reduced, which decreases partial volume averaging, or the same slice thickness can be used with a reduced number of signal averages, reducing the acquisition time. A larger B0 also allows for better discrimination in MR spectroscopy.
Answer 9
T1 relaxation is dependent on the primary magnetic field strength; a higher B0 requires higher radio frequency (RF) and greater power, which deposits more energy (heat) into the tissue. Higher RF waves result in reduced penetration, which presents image quality issues especially for deep tissue imaging. Many artifacts, such as chemical shift, are exacerbated by increasing B0.
13.3 MAMMOGRAPHY
Question 1
Why is a relatively low kVp used in mammography (23–35 kVp) versus the kVp used in other X-ray examinations (abdominal X-ray = 80 kVp; chest X-ray = 120 kVp)?
Question 2
Breasts are compressed during mammographic imaging, typically at 15 to 25 lbs of pressure. Why is this done?
Question 3
What are the factors that allow calcifications to be highly visible at the X-ray energies used in mammographic imaging?
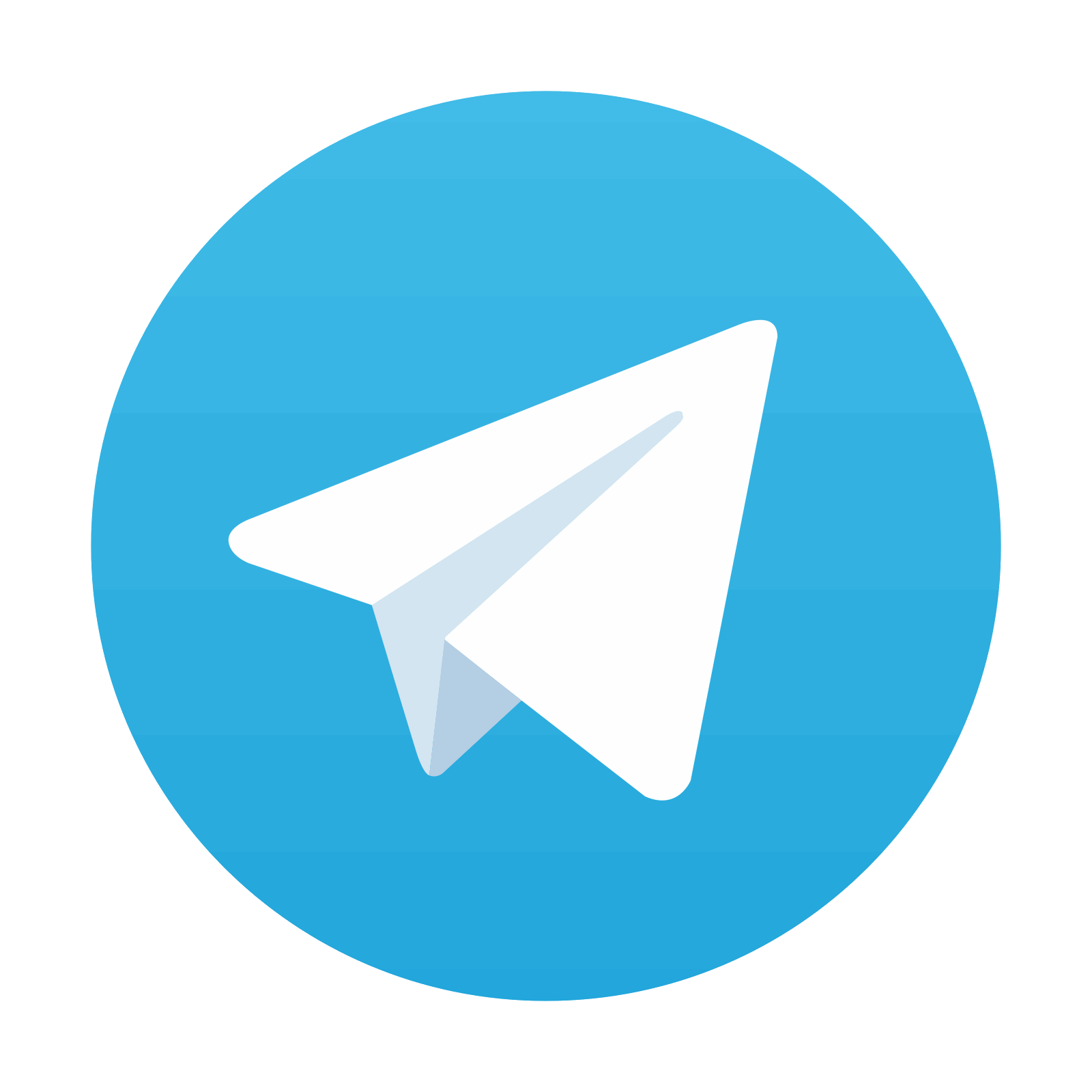
Stay updated, free articles. Join our Telegram channel

Full access? Get Clinical Tree
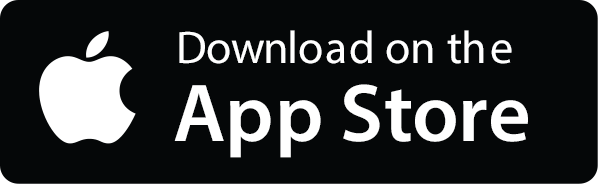
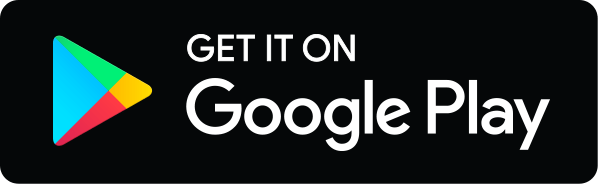