Fig. 17.1
(a) Schematic diagram showing folate-targeted nanoparticle (FNP) containing siRNA. (b) Transmission electron microscopy showing that HuR-FNPs are uniform in size and shape and do not show aggregation
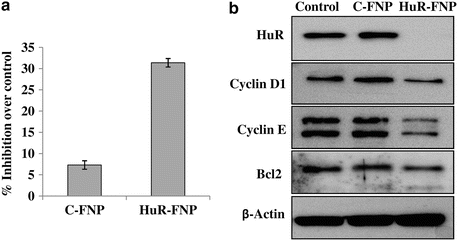
Fig. 17.2
(a) H1299 lung cancer cells were treated with C-FNP or HuR-FNP. Cells that did not receive any treatment served as control. Cell proliferation was determined at 48 h after treatment. HuR-FNP treatment showed marked inhibition of cell proliferation when compared to C-FNP. (b) Western blot showed HuR-FNP treatment reduced expression of HuR protein and HuR-regulated oncoproteins at 48 h after treatment. Beta actin was used as loading control
Since our in vitro studies established that the HuR-FNP exhibited specificity toward FRα-positive lung tumor cells resulting in antitumor activity, we next conducted pilot in vivo studies to determine if a similar antitumor activity was observed. For this purpose, subcutaneous lung tumor xenografts were established in nude mice by injecting H1299 lung tumor cells expressing the luciferase (luc) marker gene. The expression of luciferase by the tumor cells allows for monitoring tumor growth by noninvasive molecular imaging. When the tumors were about 50–100 mm in size, mice were injected intravenously with HuR-FNP via the tail vein. The HuR siRNA was fluorescently tagged with DY647 dye such that we could noninvasively monitor the nanoparticle biodistribution and accumulation in the tumor and other organs over time using the IVIS Spectrum (PerkinElmer, Waltham, MA) molecular imaging system. As shown in Fig. 17.3a, colocalization of fluorescently tagged HuR siRNA within the luciferase-positive H1299 tumor showed efficient accumulation of the particles in the tumor. The results also revealed that the FNPs were selectively internalized by the tumors compared to the other surrounding organs. From our preliminary study results, we can speculate that the nonspecific uptake of the particles by RES is significantly reduced and that pegylation of the NPs facilitated in reducing the RES-mediated clearance of the NPs.
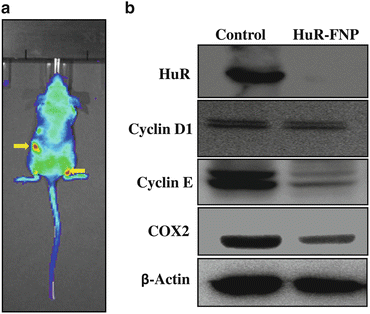
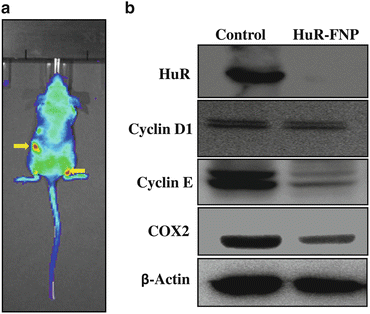
Fig. 17.3
(a) Nude mice bearing subcutaneous H1299 lung tumors and stably expressing luciferase were injected with HuR-FNP intravenously via the tail vein. HuR siRNA contained in the FNP was tagged with fluorescent dye DY647. Mice were imaged using IVIS Spectrum imaging system (PerkinElmer). Colocalization of HuR-FNP in the tumors was observed at 24 h after HuR-FNP injection. Arrows indicate HuR-FNP accumulation in the luciferase-positive H1299 tumors. (b) HuR-FNP-treated tumors showed reduced HuR, cyclin D1, cyclin E, and COX-2 protein expression compared to control tumors. Beta actin was used as loading control
To evaluate whether the accumulation of HuR-FNP in the tumor caused HuR gene silencing, tumors were harvested and analyzed for HuR protein expression. Western blotting showed that HuR protein expression was significantly reduced in tumors harvested from mice that received HuR-FNP treatment compared to HuR expression in tumor harvested from control mice that did not receive any treatment (Fig. 17.3b). Associated with the reduction in HuR protein expression was the reduction in cyclin D, cyclin E, and COX2 protein expression in the tumors from HuR-FNP-treated mice. Our preliminary in vitro and in vivo study results demonstrated that lung tumor-targeted delivery of HuR siRNA using FNPs suppresses cell proliferation and induces cell cycle arrest by global knockdown of HuR-regulated proteins resulting in an antitumor activity. While our study results are interesting, it is to be noted that additional in vitro and in vivo studies are warranted. In vivo studies that are currently underway in the laboratory are focused on determining HuR-FNP biodistribution, pharmacokinetics, and efficacy. The results of these ongoing studies are expected to be available in the future.
Conclusions
The application of siRNA technology for cancer therapy is exciting and offers an alternate cancer treatment strategy in situations where conventional therapy has failed. While several new nanoformulations are being tested, lipid-based nanoparticles continue to hold their position in drug and gene delivery with several of them being tested in the clinic. While several advantages exist in using lipid-based nanoparticles, there is still room for improvements that can be made especially in areas of size, charge, half-life, etc. The use of appropriate tumor-targeting ligand is another important aspect to consider. While several ligands have been identified for tumor-targeted siRNA delivery, it is pertinent that we use the most appropriate ligand relevant to a particular cancer type or histology for drug delivery. The use of such strategies will provide better therapeutic outcomes.
In the present chapter, we have given a brief account of siRNA-based gene silencing using tumor-targeted nanoparticles for cancer treatment. As a proof of concept, we have demonstrated that the delivery of HuR siRNA using FNP produced an inhibitory effect not only on HuR but also on HuR-regulated proteins. While targeting HuR alone is interesting, it is important to realize that combinatorial therapy or cocktail therapy might be more efficacious in reducing the tumor burden. Finally, incorporating molecular imaging agents in conjunction with HuR siRNA into FNP will provide an opportunity to monitor response to therapy in real time and facilitate important treatment decisions based on response to treatment. Finally, it is important to consider the challenges in producing clinical-grade FNP in large scale under good manufacturing practice (GMP) and ensuring that the FNPs meet the Federal Drug Administration (FDA) guidelines for approval in clinical testing.
Acknowledgments
This study was supported by the National Cancer Institute grant R01 CA167516 (RR), by the National Institutes of Health (NIH) grant P20 GM103639-02 (AM) from the COBRE Program of NIH, by the Oklahoma Tobacco Research Center (OTRC) seed grant (RR), and by the Jim and Christy Everest Endowed Chair in Cancer Developmental Therapeutics (RR). We thank the Stephenson Cancer Center at the University of Oklahoma, Oklahoma City, OK, and the Institutional Development Award (IDeA) from the National Institute of General Medical Sciences of the National Institutes of Health under grant number P20 GM103639 for the use of Molecular Imaging Core, which provided small animal imaging service. R.R. is an Oklahoma TSET research scholar and holds the Jim and Christy Everest Endowed Chair in Cancer Developmental Therapeutics.
References
Abigail KR, Jean L, Langer R et al (2011) Five years of siRNA delivery: spotlight on gold nanoparticles. Small 7:1932–1937CrossRef
Alam MR, Dixit V, Kang H et al (2008) Intracellular delivery of an anionic antisense oligonucleotide via receptor-mediated endocytosis. Nucleic Acids Res 36:2764–2776PubMedCentralPubMedCrossRef
Cheng K, Qin B (2009) RNA interference for cancer therapy. In: Lu Y, Mahato RI (eds) Pharmaceutical perspectives of cancer therapeutics. Springer, New York, pp 399–440CrossRef
Galban S, Kuwano Y, Pullmann R Jr et al (2008) RNA-binding proteins HuR and PTB promote the translation of hypoxia-inducible factor 1 alpha. Mol Cell Biol 28:93–107PubMedCentralPubMedCrossRef
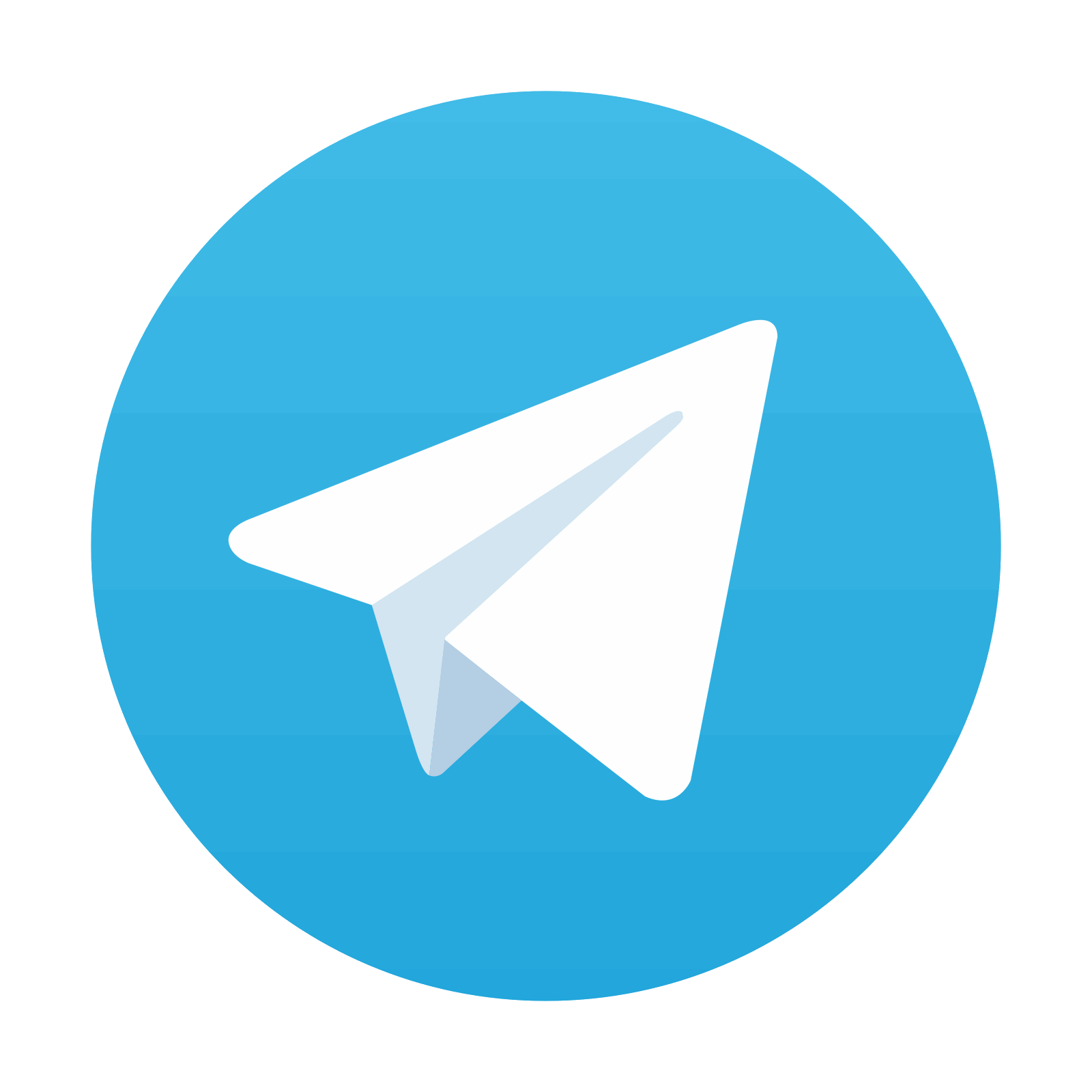
Stay updated, free articles. Join our Telegram channel

Full access? Get Clinical Tree
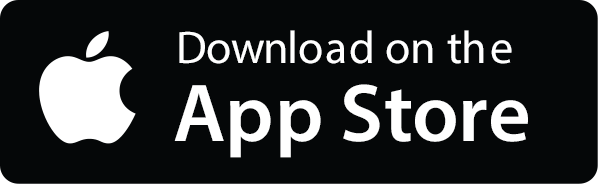
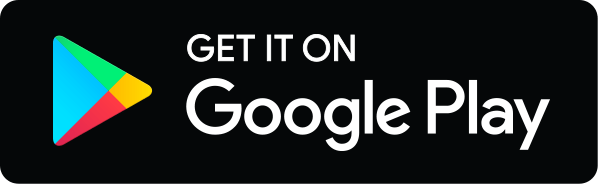