Fig. 27.1
Picture of cranial immobilization in the prone position using an aquaplast mask face cradle
An SSD or SAD setup can be used. SSD setup can facilitate the use of extended source to surface distance to increase field size. The SAD setup places isocenter at appropriate depth within the patient, and though field size is limited, this method can facilitate ease of setup and shifting between fields, as straight longitudinal shifts from the cranial field isocenter to the spine field isocenters can be used.
For the matching of multiple spine fields, the divergence from the upper and lower spine fields is matched at the anterior spinal canal (Fig. 27.2). When matching multiple spine fields in this way, the gap length between the two fields at the skin surface (often between 10 and 20 mm) is given by the following equation, where S = separation, L = the length of the PA spine field, Depth = depth at which the two fields superimpose (or the anterior spinal canal), and SSD = the source to surface distance of the PA spine field (Fig. 27.3a):

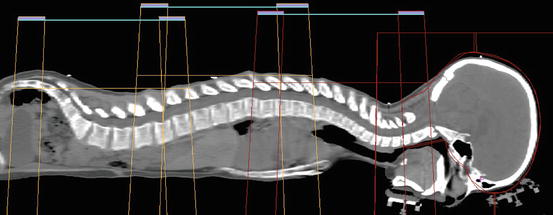
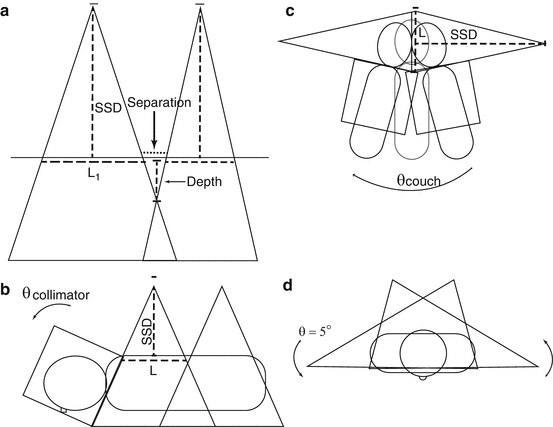

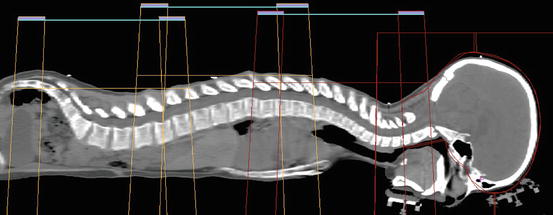
Fig. 27.2
Picture of craniospinal field matching where the divergence from the upper and lower spine fields is matched at the anterior spinal canal
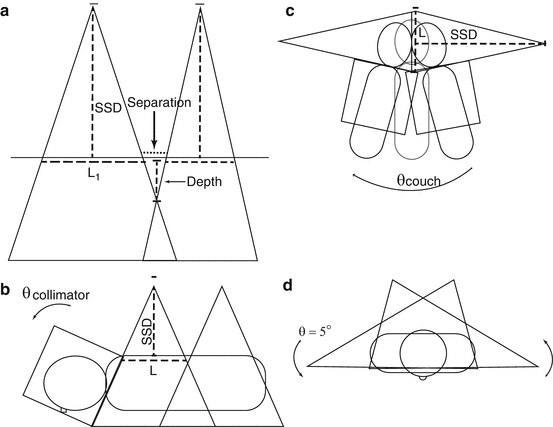
Fig. 27.3
Illustration of craniospinal field setup, including the gap calculation to allow for field matching (a), collimator rotation to match the cranial field with the superior divergence of the spine field (b), couch kick to avoid divergence from the whole brain field into the spine field (c), and a gantry rotation to create a light posterior oblique field in order to spare the contralateral lens (d)
The lower border of the cranial field should be placed in the lower cervical spine superior to the shoulders so as to avoid interference and should be collimated to match the divergence of the upper spine field. The angle of collimation can be found by the following equation (Fig. 27.3b):
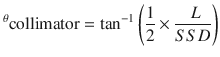
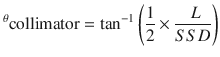
The lower cranial field and the superior spine field should nearly abut with a 0–5 mm gap. A modified table angle can additionally be used to account for caudal divergence of the craniocervical fields. This is done by rotating the couch towards the gantry by the following angle, where SAD = source to axis distance of the lateral cranial fields (Fig. 27.3c):
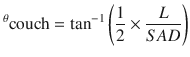
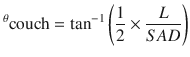
Additionally, the gantry angle of the cranial fields can be rotated in an anterior-posterior fashion by approximately 5° to create a light posterior oblique field in order to spare the contralateral lens in younger patients (Fig. 27.3d). The junctions between matching spine fields and the matching superior spine and cranial field should be feathered by 5–10 mm, approximately every 9 Gy or five fractions. Accordingly, the lower border of the cranial field and the size of each spine field must be able to accommodate repeated decrease or increase in field length as the borders are moved with feathering. The largest field size at a standard SSD should be 40 cm or less to allow for feathering.
27.4.2 Advanced Photon Techniques
The use of 3DCRT with treatment field overlaps or gaps described above can provide significant dose heterogeneity throughout the target volume as well as considerable dose delivered to organs anterior to the vertebral body. The use of more advanced photon treatment modalities, such as IMRT (Cao et al. 2012; Parker et al. 2007; Pai Panandiker et al. 2007), volumetric modulated arc therapy (VMAT) (Bedford et al. 2012; Lee et al. 2012), and helical tomotherapy (TOMO) (Sharma et al. 2009; Bauman et al. 2005), has also been described. These techniques can be incorporated into CSI treatment planning in multiple ways. IMRT can be used with the standard matched PA spine fields, to modulate the dose delivered to the planning target volume according to the varying depth and target shape, thereby improving conformality and reducing dose delivered anterior to the spinal canal (Parker et al. 2007). Further, these techniques can facilitate the use of treatment planning optimization to create a uniform dose distribution over an overlap region between two fields, and eliminate the need for field matching and feathering (Cao et al. 2012). The use of these techniques has been shown to improve dose conformality, reduce heterogeneity, and reduce the dose delivered to anterior structures such as the heart and liver as well as the total integral dose (Parker et al. 2007; Pai Panandiker et al. 2007; Bauman et al. 2005).
27.4.3 Electron Therapy
The use of electrons has also been proposed in effort to reduce acute effects of CSI by reducing the anterior depth of the treatment volume. In 1985, Maor et al. described the use of 15–17 MeV electrons prescribed such that the 90% isodose line covered at least 3 mm anterior to the estimated location of the spinal cord after correction for bone heterogeneity (Maor et al. 1985). A later comparison between patients treated with this technique and photon therapy at MD Anderson Cancer Center demonstrated that radiation dermatitis and weight loss were similar between electron and photon treated patients, while patients treated with electrons experienced higher rates of leukopenia and thrombocytopenia (Chang et al. 2002).
27.4.4 Proton Therapy
Proton radiation therapy (PRT) is an alternative to the photon therapy techniques described above. Due to the unique dosimetric properties of PRT, characterized by reduced entrance dose and minimal to no exit dose, PRT has been demonstrated to deliver equivalent target volume coverage while significantly reducing the dose delivered to all structures anterior to the vertebral bodies as well as the dose received to critical intracranial structures following boost treatment (Miralbell et al. 1997b; St Clair et al. 2004; Kirsch and Tarbell 2004) (Fig. 27.4). This reduced radiation dose delivered to normal tissues is expected to reduce multiple treatment related sequelae of craniospinal irradiation (St Clair et al. 2004; Chung et al. 2013; Merchant et al. 2008; Brodin et al. 2011; Fossati et al. 2009). Based on this dosimetric data and the promising clinical data (Yock et al. 2016; Sethi et al. 2014), the number of pediatric medulloblastoma patients treated with proton therapy is steadily increasing in the United States (Andrew 2014).
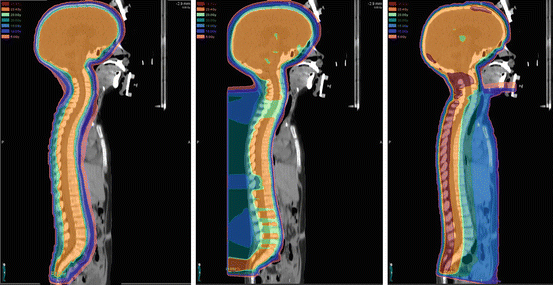
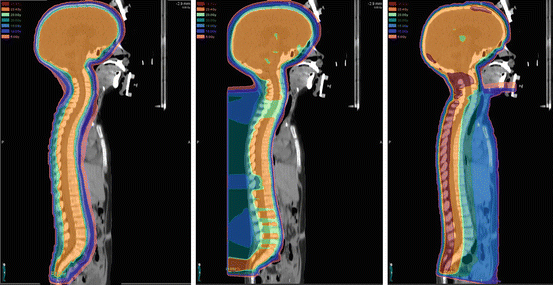
Fig. 27.4
A comparison of the dose distribution with pencil beam scanning proton therapy (left), passive scattered proton therapy (middle), and three-dimensional conformal photon therapy (right) in the sagittal plan. Note the significant sparing of structures anterior to the vertebral bodies with the two proton therapy plans as compared to photon therapy and the improved dose homogeneity and skin sparing provided by the pencil beam scanning CSI plan
Treatment setup and delivery of passively scattered three-dimensional conformal PRT are similar to that described above for 3DCRT, though subtle differences do exist. The angle of divergence for PRT beams is less than that for photon therapy, and thus the equations listed above for photon therapy do not apply. Spine fields are likewise matched at the anterior thecal sac, and this is setup visually using the simulation CT. Skin gaps, if using the prone technique, can then be directly measured from the CT to be used for daily treatment setup. The aperture of the cranial field is designed to match the minimal divergence from the superior spine field, and no collimator rotation is used. A table kick is also not required to match divergence between the cranial and spine fields. Additionally because of the absence of exit dose, a posterior-anterior oblique on the cranial fields is typically used to avoid the contralateral lens (Cochran et al. 2008). Also, the extension of the chin is of lesser concern as there is no risk for exit dose from the spine fields through the mouth and therefore chin is positioned to maximize airway safety and minimize skin folds at the back of the neck.
Intensity modulated proton therapy with pencil beam scanning (PBS) further improves the dose distribution with the use of protons by eliminating the need for field matching and improving dose homogeneity and conformality (Fig. 27.4) (Timmermann et al. 2002; Lin et al. 2014). Similar to the IMRT capabilities described above, PBS allows for treatment planning using gradient dose optimization to create a uniform dose distribution in overlap regions without requiring field matching or feathering (Lin et al. 2014; Yom et al. 2007). With the use of PBS, the cranium can be treated with two lateral or oblique fields, or with a single PA field. In addition to improving dose homogeneity and easy of treatment setting, the use of PBS as opposed to passive scattering protons may have additional benefits such as reduced skin dose, as well as reduced hardware costs and reduced neutron scatter dose (Lomax et al. 2004).
27.4.5 Treatment Setup Verification
X-ray imaging, kV or MV, is imaging which is typically used to ensure accurate alignment of bony anatomy of the cranial field prior to treatment. The spine can then be set up based on the cranial field with the use of additional kV imaging when treating in the supine position or with the use of direct visualization of light fields on the patient’s skin in the prone position. Daily imaging of the spine can also be used to ensure adequate daily setup. Using daily MV cone beam CT, Gupta et al. have analyzed the three-dimensional setup errors with the use of IMPT for CSI delivery and have demonstrated a distinct trend towards increasing inaccuracy from the brain towards the lumbar spine, with 3D vector displacement of 2.28, 1.45, and 3.15 mm for the brain, upper spine, and lower spine, respectively (Gupta et al. 2015). This study and other similar analyses highlight the need for accurate immobilization techniques, PTV margin expansions, and the use of image guidance with the delivery of CSI (Stoiber et al. 2011; Al-Wassia et al. 2013).
27.4.6 Treatment Delivery and Toxicity Monitoring
Current COG protocols for medulloblastoma and SPNET recommend the initiation of CSI within 31 days of surgery. Analysis of the HIT-SIOP PNET 4 trial demonstrated worse prognosis among patients for whom RT was delayed more than 7 week from surgery (Lannering et al. 2012), while multiple single institution retrospective reviews have failed to identify a direct relationship between clinical outcomes and time between surgery and RT initiation (del Charco et al. 1998; Paulino et al. 2003). However, effort should also be made to minimize treatment interruptions for completion of therapy within 45 days, as the prolongation of radiation treatment has been correlated with worse disease control (del Charco et al. 1998; Paulino et al. 2003). Patients receiving craniospinal irradiation with or without concurrent chemotherapy are at risk for acute hematologic and non-hematologic toxicity. Those treated with concurrent non-vincristine chemotherapy such as daily carboplatin or who have been treated with chemotherapy prior to radiation are at increased risk of leukopenias. Complete blood counts with differential should be monitored weekly during treatment. If ANC is ≤500/μL, the use of growth factors or switching to the involved field boost part of treatment should be considered. However, a break in the radiation therapy simply for uncomplicated low blood counts should be avoided to minimize the overall duration of radiation therapy (del Charco et al. 1998; Paulino et al. 2003). CSI should be resumed when the ANC has risen to >750 μL or increased by 10% on 2 consecutive days. Platelets should be transfused when <30,000/μL and clinically indicated, and CSI should be restarted when >50,000/μL or when platelet counts have increased >10% on two consecutive tests. Red blood cell transfusions may be recommended when the hemoglobin falls below 9 mg/dL. In effort to minimize treatment delays, if CSI must be held, RT can be continued by switching to the boost field.
Gastrointestinal toxicity is a common non-hematologic acute effect. Patients may be treated with ondansetron for nausea. Additional second-line medications including dexamethsone, lorazepam, antihistamines, or marinol can be used in combination with ondansetron for severe nausea. Patients who have significant postoperative residual nausea and vomiting often have significant trouble with the CSI. Severe cases have responded well to aprepitant. Nutrition should be closely monitored and patients should be weighed weekly and received nutritional counseling as appropriate. Appetite stimulants such as cyroheptadine or marinol may be of value. Enteral or parenteral support should be considered for a >10% weight loss. When delivered with chemotherapy, pneumocystis prophylaxis with trimethoprim/sulfamethoxazole given 2 consecutive days per week is often recommended for patients during and 2 months following completion of radiation therapy.
Patients who received craniospinal irradiation are at risk for multiple late treatment related sequelae, the risk of which may be dependent upon the dose received to particular tissues as well as the patient age and the receipt of additional therapies. Long-term follow-up should focus on late effect monitoring and management. COG recommendations for late effect monitoring are listed in Table 27.1.
Table 27.1
COG long-term follow-up guidelines for patients who received craniospinal irradiation
Late effect | Recommendations |
---|---|
Secondary malignancy
![]() Stay updated, free articles. Join our Telegram channel![]() Full access? Get Clinical Tree![]() ![]() ![]() |