Chapter 13
Congenital Heart Disease
David Hopkins
Introduction
Congenital heart disease in infancy and childhood refers to structural abnormalities that arise in the four main chambers of the heart itself and/or the great vessels that lead to or from the heart. The type, size and combination of lesions determines the ability of the heart and lungs to respectively pump and oxygenate blood and this in turn affects the workload and utilisation of energy in these organs. Not all cardiac lesions put an infant or child at risk of faltering growth. Some lesions, however, will result in an increase in cardiorespiratory workload that affects the amount of surplus energy available for growth. In addition some patients have defects that lead to the development of congestive heart failure (CHF), which describes a set of symptoms and clinical signs where cardiac output is inadequate to meet the metabolic demands of the body.
A glossary is given, describing cardiac anomalies and corrective procedures (p. 305) .
Incidence
The incidence of clinically relevant moderate to severe forms of congenital heart disease (CHD) is between 6 and 8 in every 1000 live births [1, 2] and forms the largest single group of congenital abnormalities. Eight lesions make up 80% of cases, the most common of which are ventricular septal defect, persistent ductus arteriosus, atrial septal defect and tetralogy of Fallot. Children with certain syndromes have a higher incidence of congenital heart defects, e.g. 50% of infants with Down’s syndrome have cardiac defects. This percentage is even higher in children with Noonan syndrome, 80%, who present mainly with pulmonary stenosis or hypertrophic cardiomyopathy [3].
Screening and diagnosis
Some congenital cardiac anomalies are frequently detected on ultrasound at routine antenatal screening. The ability to identify these anatomical defects will depend both on the skill of the operator and the timing of the antenatal ultrasound check. Some defects are discovered during the postnatal check up within the first 24 hours of birth. For example, coarctation of the aorta (narrowing in the aortic arch) may be identified by absent femoral artery pulses and ventricular septal defect (VSD), atrial septal defect (ASD) and persistent ductus arteriosus (PDA) may initially be suspected on detection of a heart murmur. Occasionally if a lesion remains undetected the child may present acutely with cardiogenic shock and elevated blood lactate levels. Alternatively a lesion may only come to light when an infant or child presents with faltering growth. A child with faltering growth whose diet history indicates a normal or above normal intake warrants a cardiac assessment as part of further clinical investigations. This is particularly so in any child who fails to gain adequate weight following optimisation of nutritional intake and the dietitian’s role may be key in highlighting suspicion. Once a suspected case has been referred to a paediatric cardiac centre diagnosis is confirmed on echocardiography (ultrasound scan) or, in very rare cases, by cardiac catheterisation.
Factors associated with poor growth in congenital heart disease
Underlying physiology and energy expenditure
Energy expenditure in patients with congenital heart defects depends on both the type and severity of the lesion. Average daily total energy expenditure (TEE) in infants without cardiac defects appears to be between 60 and 70 kcal (250–290 kJ)/kg/day [4–6] and if energy intake is at the estimated average requirement (EAR) of 100–115 kcal (420–480 kJ)/kg/day then 30–55 kcal (125–230 kJ)/kg/day should be available for growth.
The most plausible explanation for why TEE should be elevated in patients with cardiac defects is the extent to which the type and severity of the lesion affects respiratory effort [6–8]. In a normal circulation blood is pumped to the lungs by the right ventricle under pressure that is about a third of that of the left ventricle. This is because the pulmonary vascular resistance of the lungs is considerably less than that of the rest of the body. Therefore a cardiac anomaly that results in a ‘hole’ between the left and the right sides of the circulation tends to result in a greater than normal volume of blood being pumped to the lungs. This is termed a ‘left to right shunt’. If the amount of blood flow in the shunt is high, the elevated blood pressure present in the lungs is known as pulmonary hypertension [7]. This results in fluid building up in the airspaces of the lungs leading to inefficient gas exchange across the capillaries. In these cases the patient is acyanotic with adequate oxygenation of blood, but at the expense of high energy expenditure due to the increased respiratory effort. As a result patients with acyanotic lesions have a tendency to become wasted [9, 10] although length/height may also be affected if the effect is prolonged. Examples of acyanotic lesions are moderate to large VSD, PDA and atrioventricular septal defects (AVSD). If the left to right shunt is significant, congestive cardiac failure (CHF) is seen and energy expenditure is further elevated [7]. Other acyanotic lesions, however, may not cause cardiopulmonary changes that are large enough to affect energy expenditure and growth. One example is an isolated ASD where the primary repair may be carried out later in childhood with negligible effect on growth.
The presence of cyanosis appears to exert an additional independent effect resulting in stunting [11, 12]. The mechanism for this is uncertain but it has been suggested that suboptimal tissue oxygenation may be a factor [13]. Varan et al. [11] demonstrated that children with complex cardiac defects that cause a combination of both pulmonary hypertension and cyanosis appear to be at the greatest nutritional risk, with stunting occurring in addition to wasting.
Examples of a cyanotic and an acyanotic lesion and tetralogy of Fallot are shown in Figs 13.1, 13.2 and 13.3. Children with cardiomyopathy represent a smaller separate group of patients who tend to have high energy requirements.
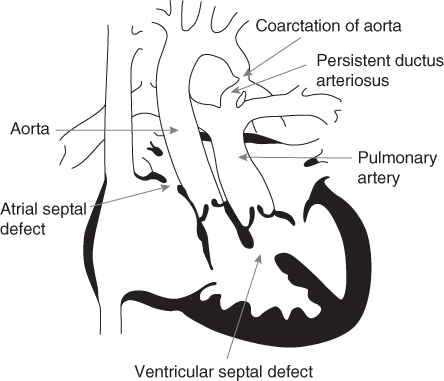
Figure 13.1 A complex cyanotic lesion.
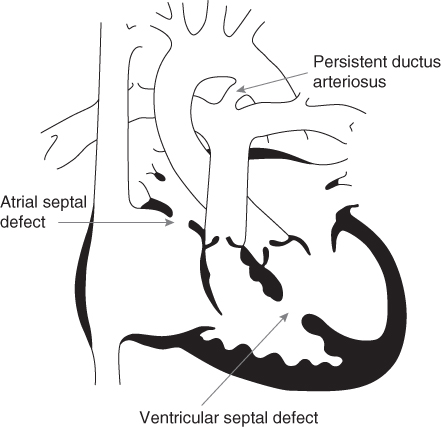
Figure 13.2 A complex acyanotic lesion.
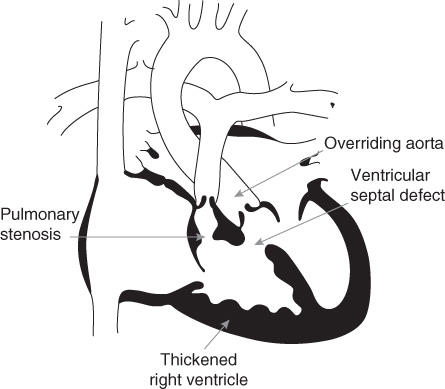
Figure 13.3 Tetralogy of Fallot.
The doubly labelled water technique has been used to calculate the energy expenditure of infants with a variety of cardiac lesions [6, 7, 10, 14]. Pre surgical infants without CHF have been shown to have an elevated TEE of 77–80 kcal (322–334 kJ)/kg/day, about 10% above normal [7]. Lesions with a large degree of left to right shunt, CHF and some cyanotic lesions appear to cause an even greater TEE of 87–94 kcal (364–393 kJ)/kg/day [6, 7, 14] with expenditure as high as 157 kcal (656 kJ)/kg/day in one individual case [10]. The effect of CHF in elevating metabolic rate may be quite marked even in infants as young as 1 month of age [15]. The more TEE is elevated, the less energy is available for accretion of new tissue resulting in the lower body mass index (BMI) and slower growth seen in these patients [6, 9, 11, 16, 17]. Malnutrition itself is also known to reduce ventricular mass and cardiac output, which may exacerbate an already compromised circulation [17].
Other proposed mechanisms by which basal metabolic rate may become elevated are hypertrophy of the cardiac muscle, increases in sympathetic nerve activity, haemopoietic tissue production, episodes of pyrexia and metabolic stress [8].
Age and timing of repair
Cardiac lesions can additionally be subdivided into those that undergo primary repair in early infancy and those that require a staged repair with further operations in later infancy or childhood. Defects such as an isolated coarctation of the aorta or transposition of the great arteries (TGA), which require repair in the neonatal period, usually have limited or no effect on long term nutritional intake and should not require dietetic intervention. Infants with several or complex cardiac abnormalities, requiring staged repair with a series of operations over time, may be at higher risk of compromised growth. Growth of infants with operated TGA has been compared with those with hypoplastic left heart syndrome (HLHS) where a staged repair involving three separate operations is usually carried out over 3–4 years [18]. Those with HLHS weighed significantly less at 1 month, 6 months and 12 months of age, took twice as long to achieve a target energy intake of 100 kcal (420 kJ)/kg/day and had a far higher incidence of feeding difficulties than the TGA group (48% vs. 4%).
In a mixed group of 150 hospitalised infants, children and young adults with CHD a third were found to have wasting (<90% weight for length/height) and 64% were stunted (<90% of mean height for age) [19]. Infants were at greatest risk due to their smaller nutritional reserve with 79% wasted and 82% stunted. Older children also need to have continuing monitoring of their nutritional status as ongoing cardiac support and intervention was required in 84% of toddlers, 58% of school-age children and 38% of adolescents [19]. Nutritional deficits and secondary growth disturbances that may ultimately put children at increased surgical risk should be corrected wherever possible [20]. Both malnutrition and growth retardation are associated with more complex CHD in infancy [9, 11, 21–24] and childhood [19].
Endocrine effects
Hormone levels may also play a role in affecting the growth of children with cardiac abnormalities. Serum concentrations of insulin-like growth factor 1 (IGF-1) are reduced in children with a VSD, with lower levels correlating with increasing size of the left to right shunt and reduced BMI [25]. Hypermetabolism is thought to be the reason for this, compromising nutritional status by reducing IGF-1 synthesis, further slowing linear growth and weight gain [25].
Substrate metabolism
Lundell et al. [26] have looked at lipid metabolism in two groups of infants with CHD. One group had VSD; the other group had cyanotic CHD. The cyanotic group all had transposition of the great arteries and a mean oxygen saturation of 68%. Both groups were given an intravenous (IV) lipid load. Severe growth retardation in infants in both groups was correlated to higher peak levels of linoleic acid in the plasma free fatty acids after IV lipid load. The peak levels of linoleic acid in the triglyceride fraction were positively correlated to weight standard deviation score. Peak glycerol levels were higher in the most growth retarded infants, indicating faster intravascular lipolysis. They concluded that lipid metabolism is disturbed in infants with CHD with infants with cyanotic lesions having a decreased ability to oxidise fatty acids [26].
Feeding behaviour
Increased breathlessness and fatigue may cause some cardiac patients to feed repeatedly for short periods of time. However, there is uncertainty as to whether actual energy intakes of infants with cardiac lesions are affected by this type of feeding behaviour. Energy intakes between 82% and 88% of those recommended have been demonstrated in some children with CHD [10, 27] but others have demonstrated near normal energy intakes [6, 7, 14, 24, 25]. Children with cardiac defects that result in both pulmonary hypertension and cyanosis have been reported as having intakes that are significantly lower than those with pulmonary hypertension or cyanosis alone [11]. Early satiety, vomiting and anorexia, all of which may be interlinked, lead to low energy intake.
Factors affecting feeding and digestive ability
Diarrhoea
Nutritionally compromised infants are more susceptible to infections and if these are frequent the use of antibiotics may affect gut flora. Patients who develop infected postoperative sternal wounds may have antibiotic therapy that results in diarrhoea which may transiently affect absorptive capacity.
Gastro-oesophageal reflux
Infants with congenital cardiac defects have been found to have delayed gastric emptying [28] and are therefore more likely to have problems with gastro-oesophageal reflux (GOR). Positioning and feed thickeners may be used as a first line treatment if there is a suspicion of GOR (p. 136) . In the intensive care setting there is often a clear correlation between deterioration in cardiac function and reduced feed tolerance, which resolves if cardiac function improves. Additional drug treatment or a resort to nasojejunal feeding may be needed to control more severe vomiting. It is therefore also worth considering other factors that may cause vomiting, e.g. cow’s milk protein allergy. A trial of cow’s milk free formula can be indicated if no other underlying cause is identified or the infant’s vomiting becomes protracted (Table 7.6).
Pain
Pain is thought to affect both the severity and length of the catabolic response to surgery. If pain is not sufficiently controlled the catabolic response following surgery might be prolonged with detrimental effects on healing [29]. In addition ongoing experience of pain might delay progression to oral feeding following surgery.
Investigations
Investigations requiring patients to be nil by mouth, such as cardiac catheterisation or computed tomography (CT) scans, will interfere with feeding regimens. Often there is limited scope to catch up with additional feeds when the patient returns to the ward and repeated investigations will compromise nutritional intake.
Faltering growth in CHD is therefore multifactorial, but from the available evidence it appears that the major factor is that of elevated energy expenditure affecting the surplus of energy that should be available for growth. An understanding of the primary cardiac anatomy helps to identify those patients who are at risk. Table 13.1 categorises the various cardiac lesions in terms of potential nutritional risk. Although most patients have improved growth postoperatively, some continue to exhibit growth failure even after haemodynamic correction [16].
Table 13.1 Cardiac defects and likelihood of dietetic intervention
Low | Variable | High |
Coarctation of aorta | Pulmonary atresia* | Ventricular septal defect (VSD) moderate to large |
Patent ductus arteriosus (PDA) if early surgery performed | Tetralogy of Fallot* | Atrioventricular septal defect (AVSD) |
Atrial septal defect (ASD) | Hypoplastic left heart* | |
Cor triatriatum | Truncus arteriosus* | |
Transposition of the great arteries (TGA)* | Aortopulmonary window | |
Total anomalous pulmonary venous drainage (TAPVD)* | PDA if large and if surgery is delayed | |
Pulmonary stenosis* | Tricuspid atresia* | |
Ebstein’s anomaly* | ||
Partial anomalous pulmonary venous drainage (PAPVD)* |
* Cyanotic lesions.
Nutritional management
If sufficient energy is provided appropriate weight gain and linear growth can be achieved [30–32]. Yahav et al. [30] found a good correlation between energy intake and weight gain, but in the most severely nutritionally depleted infants a consistent weight gain was observed only when energy intake exceeded 170 kcal (710 kJ)/kg/day. Barton et al. recommended intakes of 150 kcal (630 kJ)/kg/day in order to achieve adequate growth [10]. Nevertheless the studies measuring TEE seem to indicate that an intake of approximately 30 kcal (125 kJ)/kg/day above the EAR for energy should be sufficient to compensate for the elevated energy expenditure and achieve adequate growth in most infants [6, 7, 14, 33]. A reasonable starting point with an infant who has faltering growth would be 120–130 kcal (500–545 kJ)/kg/day, with further increase in energy intake should adequate weight gain not be achieved.
The use of growth charts is essential to plot weight and length measurements so that energy requirements can be assessed. Whilst some may require increased energy intakes to ensure adequate growth, others may be at risk of disproportionate weight gain if they continue to be given even normal energy intakes. The author’s experience is that energy intakes as low as 70 kcal (230 kJ)/kg/day are necessary to prevent excessive weight gain in some stable long term ventilated infants with cardiac defects. In these cases it is important to ensure protein, vitamin and mineral requirements are met with appropriate supplementation. Vitamin and mineral intakes of young children with CHD have been shown to be lower than those of the normal population but, with the exception of vitamin D, they still appear to meet the reference nutrient intake (RNI) [27].
Types of feed
Breast feeding or expressed breast milk, as with most infants, is the feed of choice for infants with CHD. The mechanism of breast feeding may cause less cardiorespiratory stress resulting in lower oxygen desaturation episodes [34] and has even been attempted within 1 week of heart transplant [35]. If there is ongoing fluid restriction (less than 100 mL/kg/day) beyond the first week following surgery or inadequate weight gain, fortification of the breast milk is necessary. This may be done by using one of the commercially available breast milk fortifiers for the premature infant or by adding 2%–5% infant formula powder (see Table 1.14). Addition of a commercial fortifier has been shown to have a negligible effect on delaying gastric emptying [36]. If glucose polymer is used alone to fortify breast milk or is used in combination with a fat supplement, care should be taken to ensure that the protein:energy ratio of the feed is not compromised as it may lead to a disproportionate accretion of fat at the expense of lean tissue.
If breast milk is not available or is insufficient, a suitable infant formula should be provided. Infants with CHD who have elevated energy requirements may be offered a formula with a higher energy and nutrient density:
- Infatrini 100 kcal (420 kJ)/100 mL 2.6 g protein/100 mL
- Similac High Energy 100 kcal (420 kJ)/100 mL 2.6 g protein/100 mL
- SMA High Energy 91 kcal (380 kJ)/100 mL 2.0 g protein/100 mL
Table 13.2 shows a comparison of the energy and protein obtained from standard infant formula and nutrient dense infant formula fed at increasing volumes, illustrating how nutritional requirements for growth may be met.
Table 13.2 Comparison of standard infant formula and nutrient dense infant formula
A 3-month-old infant with a congenital cardiac defect resulting in faltering growth, | ||||
weight 3.9 kg (<0.4th percentile) | ||||
Feed volumes 100, 120 and 150 mL/kg | ||||
Energy | Protein | |||
(kcal) | (kJ) | (g) | ||
Feeding 100 mL/kg | ||||
Total fluid intake 360 mL | ||||
Feeding 45 mL × 3 hourly × 8 feeds | ||||
(a) 360 mL standard infant formula | 245 | 1025 | 5 | |
per kg = | 70 | 293 | 1.4 | |
(b) 360 mL of 1 kcal/mL formula | 360 | 1505 | 9.4 | |
per kg = | 100 | 418 | 2.7 | |
DRV | per kg = | 100 (EAR)* | 420 | 2.1 (RNI)† |
Initial aim | per kg = | 120+ | 500+ | 3+ |
Feeding 120 mL/kg | ||||
Total fluid intake 440 mL | ||||
Feeding 55 mL × 3 hourly × 8 feeds | ||||
(a) 440 mL standard infant formula | 299 | 1250 | 6.6 | |
per kg = | 85 | 355 | 1.9 | |
(b) 440 mL of 1 kcal/mL formula | 440 | 1840 | 11.4 | |
per kg = | 125 | 525 | 3.3 | |
DRV | per kg = | 100 | 420 | 2.1 |
Initial aim | per kg = | 120+ | 500+ | 3+ |
Feeding 150 mL/kg | ||||
Total fluid intake 520 mL | ||||
Feeding 65 mL × 3 hourly × 8 feeds | ||||
(a) 520 mL standard infant formula | 341 | 1425 | 7.8 | |
per kg = | 97 | 405 | 2.2 | |
(b) 520 mL of 1 kcal/mL formula | 520 | 2175 | 13.5 | |
per kg = | 150 | 620 | 3.9 | |
DRV | per kg = | 100 | 420 | 2.1 |
Aim if growth falters | per kg = | 140+ | 590+ | 3.5+ |
DRV, dietary reference value.
* Estimated average requirement (dietary reference values).
† Reference nutrient intake (dietary reference values).
A high to very high energy feed may be needed to achieve adequate weight gain and appropriate catch-up growth in some infants with CHD. If there are doubts regarding an infant’s tolerance of commercial nutrient dense formulas then standard infant formula may be used initially, gradually increasing the concentration to 15%–17% to provide 72–90 kcal (300–375 kJ)/100 mL depending on the brand, before progressing to the nutrient dense formula. The strength and volume of feed can be increased as shown in Table 13.3.
Table 13.3 Increasing feed strength and fluid volume using a staged approach
Fluid | Feed | Energy | |||
mL/kg | kcal (kJ)/100 mL | kcal/kg | kJ/kg | ||
Day 1 | 100 mL | 15% standard infant formula | 72–78 (300–325) | 72–78 | 300–325 |
Day 2 | 120 mL | SMA High Energy | 91 (380) | 109 | 455 |
Day 3 | 120 mL | Infatrini or Similac High Energy | 100 (420) | 120 | 50 |
Day 4 | 140 mL | Infatrini or Similac High Energy | 100 (420) | 140 | 585 |
Day 5 | 150 mL | Infatrini or Similac High Energy | 100 (420) | 150 | 630 |
If weight gain is unsatisfactory on 1 kcal (4 kJ)/mL feed fed at 150 mL/kg, and it is not possible to increase the volume of feed, then a further gradual increase in energy density of the feed should be undertaken with the addition of glucose polymer or Duocal (fat and carbohydrate) in 1% daily increments up to a maximum of 4% until weight gain is achieved. At this level of fortification a reasonable protein:energy ratio of 9% is maintained. The final feed provides an energy density of 1.2 kcal (5 kJ)/mL. Alternatively if the protein:energy ratio needs to be better preserved then addition of infant formula powder in 1% increments up to 4% may be considered, achieving a protein:energy ratio of approximately 10%. As these infants are often at risk of GOR [28] a fine balance has to be made between giving a feed of sufficient energy density to achieve adequate growth whilst limiting vomiting, malabsorption or, if feeding via nasogastric tube, occurrence of large gastric aspirates.
The patient in the paediatric intensive care unit
Energy expenditure
Following cardiac surgery the majority of patients are sedated, paralysed and mechanically ventilated whilst in the paediatric intensive care unit (PICU). Unfortunately predictive energy expenditure equations have been found to be inadequate in calculating nutritional requirements for these patients [37, 38]. One study using indirect calorimetry in infants and young children in the PICU found an average energy expenditure of 68 kcal (284 kJ)/kg/day [37]. Cyanosis did not appear to have any effect on postoperative metabolic rate [37] but those patients who underwent cardiopulmonary bypass appear to have had higher postoperative energy expenditure (74 kcal (309 kJ)/kg/day) than those who did not (58 kcal (242 kJ)/kg/day). Avitzur et al. [39] found lower levels of postoperative energy expenditure, but again no difference between acyanotic and cyanotic patients, 62 kcal (259 kJ) vs. 59 kcal (247 kJ)/kg/day. Infants with HLHS in the immediate 3 days following their first complex Norwood stage 1 operation have been shown to have even lower energy expenditure, 39–43 kcal (163–180 kJ)/kg/day [40], although energy intakes failed to meet even these requirements.
Energy requirements during the first few postoperative days therefore appear to be close to or even below normal. However, once the patient comes off the ventilator, changes in metabolic rate will depend on any residual lesion that affects cardiopulmonary function.
Fluid restrictions
There is a need to limit the amount of fluid in the circulatory system postoperatively as cardiopulmonary bypass causes oedema of all the body’s tissues which compromises organ function. Keeping the lungs relatively dry also helps to wean the patient off the ventilator. As a result there is often a limited fluid volume for feeding in the first day or so after operation. In small infants with a large number of IV drug infusions, e.g. 5–6 mL/hour, there is usually limited space for meaningful volumes of feed until at least an 80% fluid allowance is reached and intensive dietetic intervention may not be feasible (see example below). As energy intake is limited during this period, blood sugar monitoring is required with correction being made if they are found to be low. The permitted amount of fluid in the days immediately following surgery varies between units, but inevitably results in insufficient volume to achieve adequate nutrition. However, fluid restrictions are usually temporary and there is opportunity to improve intake as the feed volumes increase.
Fluid allowances on cardiac intensive care units are often expressed in terms of a percentage and are calculated cumulatively as follows:
- 4 mL/kg/hour for the first 10 kg in weight
- 2 mL/kg/hour for the second 10 kg in weight
- 1 mL/kg/hour for every kilogram above 20 kg
These volumes provide a patient with a ‘100%’ fluid allowance. This may not, however, equate to normal fluid requirements, particularly for an infant or small child, and nutrition can easily be compromised.
Table 13.4 illustrates how fluid allowances might be increased following surgery depending on whether the patient has undergone cardiopulmonary bypass or not. Volumes of intravenous drug infusions are included in the total fluid allowance leaving limited room for feed in the days immediately after surgery.
Table 13.4 Fluid allowance during admission to the paediatric intensive care unit after cardiac surgery
Day post surgery | Cardiopulmonary bypass | Non cardiopulmonary bypass |
1 | 50% | 80% |
2 | 60% | 90%–100% |
3 | 70% | 120% |
4 | 80% | Free fluids |
5 | 90% | |
6 | 100% | |
7 | 120%–135% | |
8 | 150% |
Infants and children without cardiac defects are known to be at risk of developing malnutrition following admission to the PICU, with those admitted from wards and other hospitals being at greatest risk [41], and aggressive nutritional therapy may improve some biochemical markers of nutritional status (p. 72) . Should the clinical condition and/or fluid balance of the patient result in fluid restrictions continuing beyond the expected time frame steps should be taken to ensure nutritional requirements are met. Less than a third of children undergoing cardiac surgery have been shown to achieve their estimated energy requirement whilst on the intensive care ward and have significant falls in weight during their stay [42].
Following discharge from the PICU the fluid allowance of patients is often increased and provides an opportunity to improve feed intake. Infants provided with an 80 kcal (334 kJ)/100 mL feed on day 1 of discharge from the PICU, increasing to 100 kcal (420 kJ)/100 mL feed on day 3, have demonstrated significant improvements in weight gain at discharge compared with controls [43]. Increases in the energy density of feeds may be well tolerated by some but may also result in vomiting necessitating use of anti-reflux treatments.
Methods of feed administration
The majority of patients admitted to the PICU are fed via nasogastric tube for the first few days following cardiac surgery. However, once discharged to the ward most should have transitioned or be in a position to transition to oral feeds. On discharge from hospital the majority of cardiac infants should be taking feeds orally, but some may fail to complete feeds and others may require ongoing tube feeds. This may be due to fatigue brought on by the effort of sucking, anorexia or early satiety. Factors that have been shown to have a detrimental effect on oral feeding capability are vocal chord injury, long postoperative ventilation and poor weight at surgery [44].
If an infant is regularly failing to complete feeds one of the following strategies should be employed:
- offer smaller more frequent feeds orally
- complete feeds via nasogastric tube
- give small frequent bolus feeds via nasogastric tube
- top up small frequent daytime feeds with continuous feeds overnight via an enteral feeding pump
- give feeds continuously over 20–22 hours via an enteral pump, allowing the gut to rest for a short period and gastric pH to return to normal
Feeding an infant or young child overnight via a nasogastric tube, whether in hospital or at home, requires a risk assessment. There have been a number of studies looking at the efficacy of methods of feed administration in infants with CHD. Children with complex congenital heart lesions who fail to achieve adequate weight gain with oral fortified feeds have been shown to have greater energy intakes and weight gain using the same feeds given as continuous nasogastric infusions [20]. It has been suggested that continuous feeding causes a smaller rise in basal metabolic rate and heart rate than occurs after bolus feeds [45]. Feeding 1 kcal (4 kJ)/mL formulas orally; or as oral daytime feeds plus 12 hours overnight continuous nasogastric feeding; or as 24 hours continuous nasogastric feeds has been compared in a group of infants with CHD and CHF [46]. During the 5 months of the study only the group of infants receiving 24 hours continuous nasogastric feeds achieved target intakes in excess of 140 kcal (585 kJ)/kg/day. However, others have demonstrated improvements in energy intake and body weight with nutritional counselling alone [31].
Improved standard deviation scores (SDS) for weight has been demonstrated in 37 patients with cardiac defects who had undergone percutaneous gastrostomy placement [47]. Comparison with nasogastric fed controls is difficult, however, due to differences in starting SDS for weight. Patients who require long term tube feeding may benefit from a gastrostomy as it may present less of an impediment to the development of oral feeding skills. The timing of weaning off gastrostomy feeds to allow for the development of independent feeding skills should be addressed for each individual child.
Other nutritional issues
Sodium supplementation of infant feeds
Some infants with CHD fail to gain weight on an energy intake in excess of 140 kcal (585 kJ)/kg. The use of diuretics makes electrolyte depletion a possibility. If this is suspected a 24 hour urine sodium balance should be done to establish urinary sodium losses and sodium supplementation should be considered. An infant feeding 150 mL/kg of a standard infant formula receives 1.0–1.3 mmol Na+/kg, depending on the formula, compared with the RNI for sodium for an infant aged 0–3 months of 1.5 mmol Na+/kg. Infants feeding 150 mL/kg of one of the commercially available higher energy formulas will receive between 1.4 and 1.65 mmol Na/kg.
Anticoagulation and the vitamin K content of feeds
Children undergoing Blalock–Taussig (BT) and Glenn shunts, total cavo-pulmonary connection (TCPC or Fontan operation), pulmonary artery reconstruction and some operations requiring tissue homografts are given either aspirin or warfarin postoperatively for anticoagulation. Vitamin K has an antagonistic effect on some of the anticoagulation drugs and may be given in some instances to counter excessive anticoagulation, the dose being 300 µg/kg/day. While most cardiac infants tolerate whole protein feeds there may occasionally be a need to use a hydrolysed protein formula (Table 7.6), some of which have high vitamin K content, e.g. Pregestimil. An infant weighing 5 kg feeding 150 mL/kg/day of such a formula may receive an additional 60 µg of vitamin K per day. If a patient is anticoagulated the international normalised ratio (INR) should be monitored in the days following the introduction of such formula.
Outcome of patients with specific cardiac lesions
Advances in surgical expertise in recent years has meant that increasing numbers of infants with severe cardiac lesions are surviving and being discharged into the community. The nutritional status of infants and children with CHD depends on both the type of lesion and the number of operations that they require. The more complex the lesion and the greater the number of planned operations, the greater the nutritional risk to the patient. The following two lesions, however, present a relatively low nutritional risk in spite of their complexity and for two separate reasons.
Transposition of the great arteries (TGA)
With TGA the two main arteries exit from alternate ventricles from those they would normally use. The aorta exits from the right ventricle rather than the left and the pulmonary artery exits from the left ventricle. The patient is able to survive because blood can mix through a patent foramen ovale (PFO) and a PDA. The patient is almost always given prostaglandin to try to keep the PDA open. In about 25% of patients the PFO is enlarged, using a cardiac catheter in a procedure called a balloon atrial septostomy, and this allows for better mixing of blood. The patient is then allowed to recover before an operation is carried out at about 1 week of age in which the arteries are switched over to their correct position; the hole between the atria is repaired and the PDA is closed. Unlike most infants with congenital heart defects, those with TGA tend to have normal birth weights [12, 16] although the reason for this is uncertain. As they undergo the repair of this complex defect within the first few weeks of life they are able to be discharged, feeding normally, usually with no need for dietetic intervention.
Tetralogy of Fallot
There are four separate aspects to this complex cyanotic defect: a ventricular septal defect; an overriding aorta in which the aorta straddles the two ventricles; obstruction of pulmonary blood flow from the right ventricle (sub pulmonary stenosis) and right ventricular hypertrophy (Fig. 13.3). A ventricular septal defect on its own will usually cause a left to right shunt with excessive blood flow to the lungs and the patient being at risk of developing pulmonary hypertension and associated faltering growth. However, with tetralogy of Fallot the right ventricular outflow tract obstruction protects them from excessive lung blood flow. Due to the obstruction the workload of right ventricle is increased resulting in it becoming hypertrophied but this does not appear to affect growth. Rarely, if the patient is very blue (cyanosed) a Blalock–Taussig (BT) shunt operation is initially performed (p. 305) early in life to try to normalise pulmonary blood flow.
Once stabilised or after recovery from the shunt operation the infant is able to be discharged home after a short inpatient stay and most cases achieve adequate weight gain. However, those who have undergone a BT shunt may be at risk of developing a degree of excessive pulmonary blood flow thereby increasing respiratory effort, which may require nutritional support. At about 4–6 months of age when the infant has had a chance to grow the reparative operation is carried out with the VSD being closed, the outflow obstruction repaired and (if necessary) the BT shunt being taken down. On discharge they usually require no further nutritional support.
Hypoplastic left heart syndrome
In contrast to the above two conditions, patients who have undergone the first stage of repair (the Norwood operation) for HLHS constitute a group that is at particular nutritional risk. Almost a third of patients with HLHS have been shown not to meet normal energy requirements during their hospital stay with almost 60% not regaining their birth weight by discharge following their first operation [48]. Introducing standardised management protocols, including home surveillance programmes, has been shown to improve intraoperative survival rates and poor weight gain is used as one of the indicators for earlier surgical intervention [49, 50]. Within this group of patients, a gastrostomy has been linked to improved survival independently of weight gain [50]. It may be that improved survival is linked to reduced use of nasogastric tubes with these being a route for hospital acquired infection.
Complications of congenital heart disease affecting nutritional status
Chylothorax
Normal transport of chyle
After digestion in the intestinal lumen dietary fats are absorbed into the mucosal cells as glycerol and fatty acids. Long chain fatty acids of 12 or more carbon atoms are re-esterified to triglycerides and pass into the thoracic lymph duct as chyle. Sixty to seventy per cent of absorbed dietary fat passes through the lymphatic system at a concentration of between 5 and 30 g/L. Flow increases markedly after ingestion of a high fat meal with lesser but definite increases after mixed meals of protein, carbohydrate and fat [51]. The principal function of the thoracic lymph duct is to transport chyle to where it enters the venous system near the junction of the left internal jugular and subclavian veins [52] and these vessels then drain into the superior vena cava. Figure 13.4 shows the anatomy of the thoracic lymph duct.
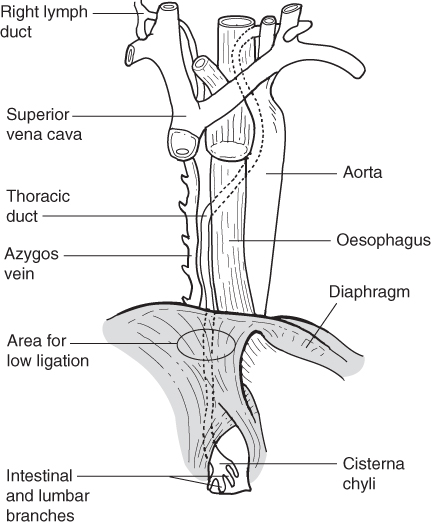
Figure 13.4 Anatomy of the thoracic duct, (Source: Merrigan et al. [57].)
Chylothorax is caused by a fistula between the thoracic lymph duct and the pleural cavity resulting in the accumulation of chyle in the pleural cavity itself. Chyle contains lymphocytes, immunoglobulin, enzymes, triglycerides, cholesterol and fat soluble vitamins the loss of which, if unchecked, would invariably lead to serious metabolic deficit.
Fistulas
There are three types of fistula.
- Traumatic fistula: the thoracic duct drains into the vessels close to where some cardiac surgical procedures are carried out. As the thoracic duct is difficult to see there is an increased risk of disruption and damage to the duct during surgery, resulting in a traumatic fistula.
- Obstructive fistula: these result from increased pressure in the thoracic lymph duct itself which causes its walls to become leaky. This may be secondary to increased venous pressure due to sluggish blood flow or clots in the veins distal to where the thoracic duct empties. Patients with right-sided obstructive cardiac lesions require venous to pulmonary artery shunt operations to achieve longer term oxygenation. Theoretically procedures that affect venous return on the right side of the heart are more likely to result in obstructive chylothorax. The Glenn and TCPC shunt operations are two examples where the venous return is plumbed directly into the pulmonary vasculature to try to achieve adequate oxygenation. Under these circumstances the blood flow in the lungs is increased resulting in ‘back pressure’ into the pulmonary arteries and the newly connected systemic veins. As a result the lymph does not drain well into the veins and the lymphatic vessels themselves become engorged and leaky, with chyle passing into the thoracic cavity. However, it appears that the increased risk of developing chylothorax only occurs with the larger TCPC shunt [53]. An exacerbating factor may be the development of either a chest infection or a postoperative pleural effusion and chylothorax may occur some days after operation. A list of cardiac defects that may require these operations is shown in Table 13.5.
- Congenital fistula: some children may develop congenital chylothorax [54], with children having Noonan syndrome being at higher risk.
- Obstructive fistula: these result from increased pressure in the thoracic lymph duct itself which causes its walls to become leaky. This may be secondary to increased venous pressure due to sluggish blood flow or clots in the veins distal to where the thoracic duct empties. Patients with right-sided obstructive cardiac lesions require venous to pulmonary artery shunt operations to achieve longer term oxygenation. Theoretically procedures that affect venous return on the right side of the heart are more likely to result in obstructive chylothorax. The Glenn and TCPC shunt operations are two examples where the venous return is plumbed directly into the pulmonary vasculature to try to achieve adequate oxygenation. Under these circumstances the blood flow in the lungs is increased resulting in ‘back pressure’ into the pulmonary arteries and the newly connected systemic veins. As a result the lymph does not drain well into the veins and the lymphatic vessels themselves become engorged and leaky, with chyle passing into the thoracic cavity. However, it appears that the increased risk of developing chylothorax only occurs with the larger TCPC shunt [53]. An exacerbating factor may be the development of either a chest infection or a postoperative pleural effusion and chylothorax may occur some days after operation. A list of cardiac defects that may require these operations is shown in Table 13.5.
Table 13.5 Conditions requiring Glenn and/or total cavo-pulmonary connection (TCPC) shunt operations (see p. 305 for definitions)
Heart defect | Operation |
Ebstein’s anomaly | TCPC* |
Pulmonary atresia with/without intact ventricular septum | Glenn shunt and TCPC |
Tricuspid atresia | TCPC |
Single ventricle physiology | Glenn shunt and TCPC |
Hypoplastic left heart | Norwood stage 2 and 3† |
* TCPC is one of a number of surgical options in Ebstein’s anomaly.
† The second stage of the Norwood procedure may be similar to a Glenn shunt or TCPC and the third stage may be a TCPC operation.
Diagnosis
As chyle contains fat from the intestinal lacteal system it has a characteristically milky appearance that often raises suspicion when it appears in post surgical pleural chest drains. A chest drain sample with a triglyceride content of >1.1 mmol/L, a total cell count >1000/μL with a predominance of lymphocytes or the presence of chylomicrons has been used to confirm diagnosis [53, 55] although others use a combination of these [56]. As the concentration of triglycerides in chyle is higher than that of plasma this has also been used to differentiate chylous pleural effusions from those of other origin [57].
Incidence and duration
The incidence of chylothorax is between 1.34% and 9.2% following cardiac surgery for CHD [53, 55, 58]. Low body weight, longer cardiopulmonary bypass time and secondary chest closures are all associated with increased risk of developing chylothorax [58]. A higher incidence of chylothorax has been noted in patients undergoing repair of tetralogy of Fallot, the TCPC procedure and heart transplantation [53].
A median duration of 9–15 days is expected before chest drain output ceases [53, 58] although up to a quarter of cases have continued outputs beyond 30 days [53]. The amount of chylous drainage varies between 2 and 40 mL/kg in most cases [55, 58, 59] with high outputs >100 mL/kg/day being indicative of dietary treatment being unsuccessful [58].
Treatment options
Chylothorax can be treated conservatively using a minimal long chain triglyceride (LCT) diet [53, 55, 58, 60] or total parenteral nutrition (TPN) [55]. However, it has been proposed that some suspected cases of chylothorax may be self-limiting with a median resolution time of 1 week following presentation [56].
The aim of the minimal LCT diet is to reduce the lymph flow and so allow the fistula to heal. Dietary fat is mainly composed of LCT. Normally medium chain triglycerides (MCT), with 6–10 carbon atoms in the fatty acid chain, do not constitute more than a minor proportion of dietary fats. However, as they are absorbed directly into the portal vein as ‘free fatty acids’ bound to albumin (Fig. 13.5) they constitute a useful additional source of energy in a diet that may otherwise be low in energy. Restriction of free water intake during the treatment period may have a beneficial effect on outcome.
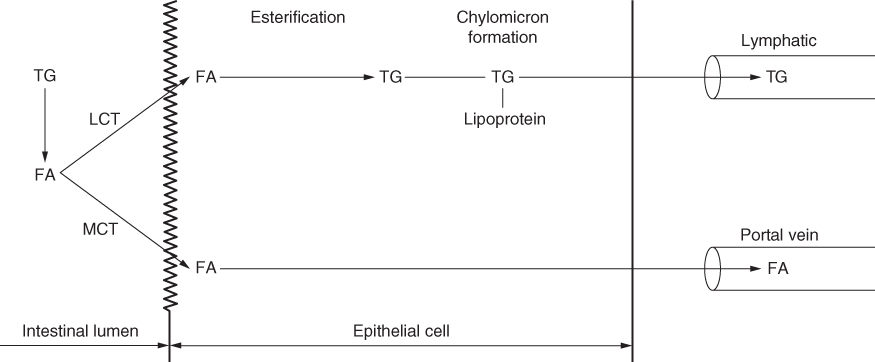
Figure 13.5 Scheme of the pathways of absorption of long chain triglyceride (LCT) and medium chain triglyceride (MCT). FA, fatty acid; TG, triglyceride.
There have been individual case reports of the hormone somatostatin or an analogue octreotide, both of which have antisecretory and antidiarrhoeal properties, being used in isolation or as an adjunct to a minimal LCT diet or PN to treat refractory chylothorax. A dose of 1–4 µg/kg/hour is recommended [61, 62] although benefit has been noted on smaller doses [63]. Despite some promising case reports [61–64] results appear to be inconsistent [55]. Surgical ligation of the thoracic lymph duct is usually reserved for patients who do not respond to 3–4 weeks of dietary and medical treatment [55, 65].
Prognosis
The conservative dietary approach has a success rate of between 70% and 80% [53, 58, 60]. A useful algorithm for the management of chylothorax has been produced by Cormack et al. [60] and is shown in Fig. 13.6. However, development of chylothorax has been shown to almost triple the duration of hospital stay [53].
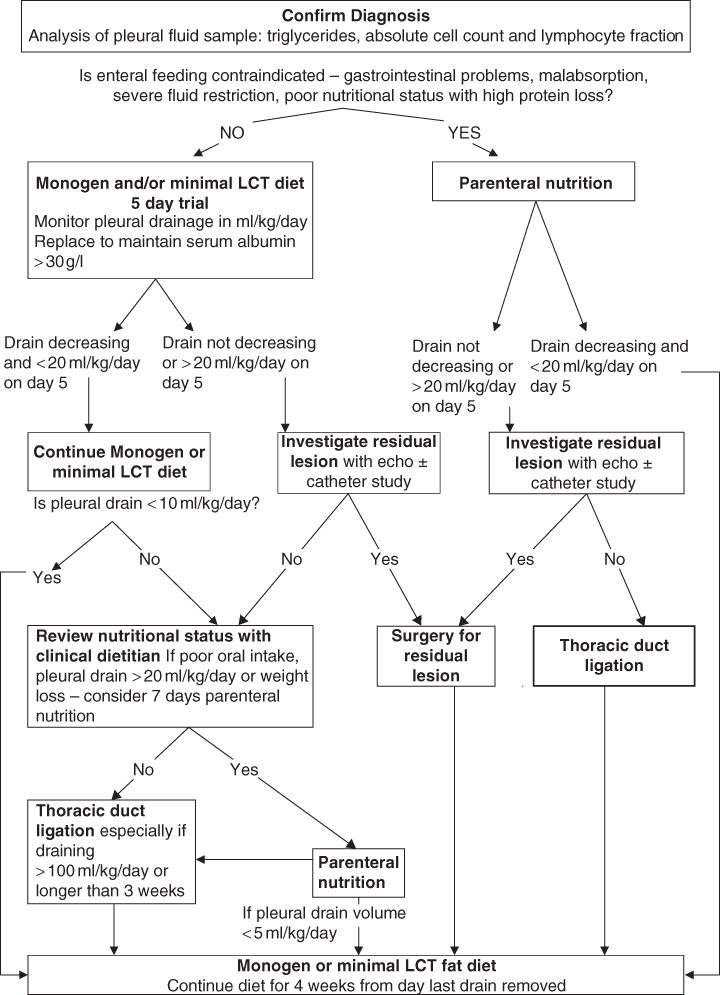
Figure 13.6 Chylothorax algorithm.
Dietary treatment of chylothorax
A general guide has been to give not more than 1 g LCT per year of life up to a maximum of 4–5 g LCT/day. The origin of this practice is Diets for Sick Children, 4th edition, 1987, Dorothy Francis. However, the evidence base for this is poor and the guidance is often breached, particularly in older infants and younger children where 1–2 g LCT/day is very difficult to manage.
Minimal fat feeds for the infant with chylothorax
- Monogen
- MCT Pepdite
- Minimal LCT modular feed based on Pepdite Module 767
Monogen
Monogen is a whey protein based infant formula with low LCT content. The fat sources are fractionated coconut oil and walnut oil. Monogen was previously thought to contain 0.21 g of LCT per 100 mL formula at standard dilution (17.5%). However, recent analysis by the manufacturer has revealed that the amount of LCT is closer to 0.38 g/100 mL (Table 13.6).
Table 13.6 Nutritional content of standard Monogen per 100 mL
Energy | 74 kcal/310 kJ |
Protein equivalent | 2.2 g |
Carbohydrate | 12 g |
Fat | 1.9 g |
MCT | 1.52 g |
LCT | 0.38 g |
If the infant has previously been tolerating normal or concentrated feeds prior to the development of chylothorax, it should be possible to introduce Monogen at full strength despite the relatively high carbohydrate content (12%). If the baby has had limited enteral nutrition then, as a precaution, Monogen may be introduced at half strength, i.e. 9% dilution in the first 12–24 hours. Standard dilution provides 74 kcal (310 kJ)/100 mL. In cases of severe fluid restriction it has previously been advocated to gradually increase the concentration of the feed in order to meet requirements. In view of the current higher LCT content of the feed, it might be prudent to increase energy density using either glucose polymer (in 1% increments) or MCT emulsion (in 1%–2% increments) up to an energy density of 90 kcal (375 kJ)/100 mL. This allows an increase in energy density whilst maintaining a protein:energy ratio close to 10%. As Monogen is a complete infant formula supplementation with essential fatty acids (EFA) should not be needed. However, falls in EFA status have been noted following commencement on Monogen feeds (personal communication).
In order to achieve adequate energy intake to facilitate growth the total LCT intake may be greater than the 1 g per year of life recommendation. As long as chest drain output is unaffected then a higher LCT intake should be allowed to continue. If chest drains are not in situ then clinical signs such as increased respiratory effort can be used to monitor tolerance to increasing amounts of LCT. A chest x-ray may confirm re-accumulation of chyle in the pleural cavity.
MCT Pepdite
If whole cow’s milk protein is contraindicated, e.g. where the infant has a proven cow’s milk protein allergy, Monogen cannot be used. MCT Pepdite has been used on some units (personal communication) but it has a higher LCT content: 0.68 g/100 mL (25% of total fat) compared with 0.38 g/100 mL (20% of total fat) in Monogen. An infant weighing 3.5 kg feeding 150 mL/kg MCT Pepdite will receive in excess of 3.5 g LCT/day. In some cases this may provide too much long chain fat and a minimal LCT modular feed may be required to reduce chyle flow adequately.
Minimal LCT modular feed
A modular feed can be designed using Pepdite Module 767 as a minimal fat protein source. It is free of cow’s milk protein. To produce a nutritionally adequate infant feed it is necessary to add carbohydrate, a suitable fat source, electrolytes, minerals and vitamins. An example of this feed is shown in Table 13.7.
Table 13.7 A modular feed for treating chylothorax
A 3-month-old infant develops a chylothorax post cardiac surgery. Whole cow’s milk protein is contraindicated | ||||||
Weight 4 kg, fluid volume 150 mL/kg | ||||||
Na+ | K+ | Energy | CHO | Protein | MCT | |
(mmol) | (mmol) | (kcal/kJ) | (g) | (g) | (g) | |
16 g Pepdite Module 767 | 55/230 | 13.8 | ||||
60 g glucose polymer | 0.5 | neg | 232/970 | 58 | ||
24 mL Liquigen | 0.3 | 108/450 | 11.8 | |||
17 g Paediatric Seravit | 0.15 | 51/215 | 12.8 | |||
5 mL KCl (2 mmol/mL) | 10 | |||||
4 mL NaCl (1 mmol/mL) | 4 | |||||
+ water to 600 mL | ||||||
Totals | 4.9 | 10 | 446/1865 | 70.8 | 13.8 | 11.8 |
per 100 mL | 0.8 | 1.6 | 74/311 | 11.8 | 2.3 | 2 |
per kg | 1.2 | 2.5 | 111/466 | 3.4 | ||
Day 1 | Day 2 | Day 3 | ||||
4 g Pepdite Module 767 | 8 g Peptide Module 767 | 12 g Pepdite Module 767 | ||||
15 g glucose polymer | 30 g glucose polymer | 45 g glucose polymer | ||||
6 mL Liquigen | 12 mL Liquigen | 24 mL Liquigen | ||||
6 g Paediatric Seravit | 12 g Paediatric Seravit | 17 g Paediatric Seravit |
Aims in constructing feed: 3+ g protein per kg; 74 kcal (310 kJ) per 100 mL; 12% carbohydrate concentration.
The feed concentration should be gradually increased daily as shown to achieve the full strength.
Once the full strength feed is tolerated, weight gain should be reviewed. It may be necessary to increase the energy density of the feed to facilitate weight gain. This should be done by the incremental addition of glucose polymer and MCT emulsion, e.g. Liquigen. A total of 12% carbohydrate as glucose polymer and 4% fat as MCT emulsion should be tolerated. If sufficient quantities of a vitamin and mineral preparation, e.g. Paediatric Seravit, are added to the modular feed there should be no need for additional supplementation with the exception of sodium and potassium. If the infant consistently takes <150 mL/kg then the need for supplementation should be assessed.
Essential fatty acids
EFA in phospholipids are important for maintaining the function and integrity of cellular and subcellular membranes. They also participate in the regulation of cholesterol metabolism, being involved in its transport, breakdown and ultimate excretion. Long chain polyunsaturated fatty acids (LCP) are important in the growth and development of term infants and their addition to the diet in appropriate quantities is safe [66]. A deficiency state arising from an inadequate intake of linoleic acid has been demonstrated in children [67]. Although a specific deficiency state arising from inadequate dietary α-linolenic acid has not been demonstrated in healthy humans, it is regarded as a dietary essential [67]. It has been recommended that the neonate requires at least 1% of energy intake from linoleic acid (C18:2) and at least 0.2% of energy from α-linolenic acid (C18:3) [68]. On re-analysis it appears that Monogen has slightly over the required percentage energy from linoleic acid whilst having slightly less α-linolenic acid. Table 13.8 gives a comparison of EFA content of feeds used in the treatment of infants with chylothorax.
Table 13.8 Percentage energy derived from linoleic and α-linolenic acids in minimal LCT feeds
Energy from C18:2 (%) | Energy from C18:3 (%) | |
Monogen | 1.1 | 0.17 |
MCT Pepdite | 5.1 | 0.75 |
Minimal LCT | 0 | 0 |
modular feed |
EFA supplementation of feeds
If EFA need to be added to a feed this may be done using walnut oil; the fatty acid content provides linoleic and α-linolenic acids in an acceptable quantity and ratio. Walnut oil contains 55 g linoleic and 12 g α-linolenic acids per 100 mL (Table 19.4). The addition of 0.15 mL of walnut oil to 100 mL of the minimal LCT Pepdite Module 767 based feed will provide EFA (and at the same time contribute a minimum amount of LCT) to a minimum of 1% energy from linoleic acid and 0.2% energy from α-linolenic acid. As the energy content of the feed is increased it will be necessary to increase the EFA supplementation proportionately.
Minimal LCT weaning diet
The following foods contain minimal LCT and can be introduced as weaning solids:
- puréed vegetables, e.g. potato, carrots, swede, green beans
- puréed fruit, e.g. pears, apples, banana, peaches
- puréed boiled rice mixed with minimal fat milk
- baby rice reconstituted with minimal fat milk
- tins and jars of baby foods containing less than 0.2 g LCT per 100 g may be included in the diet (mainly the fruit based tins and jars). Other commercial baby foods may contain too much LCT and need to be avoided
Even when solids are introduced into the diet of the infant, a minimal LCT formula will continue to form a major part of the nutritional intake providing energy, protein, vitamins and minerals and is an essential part of the diet until at least 1 year of age. A sample day’s menu for the toddler on a minimal LCT diet is given in Table 13.9.
Table 13.9 Sample day’s menu for a toddler on a minimal LCT diet
Minimal fat milk (MFM) | |
60 g skimmed milk powder | Provides 500 kcal (2090 kJ) |
35 mL Liquigen | 22 g protein |
30 g glucose polymer | 0.8 g LCT |
8 g Paediatric Seravit | |
+ water to 600 mL | |
Use throughout the day for drinks and mixed with appropriate foods | |
Breakfast | 10 g Rice Krispies + 60 mL MFM |
Glass MFM (100 mL) | |
Mid morning | Glass MFM (100 mL) |
2 MCT biscuits | |
Lunch | 80 g baked beans + 50 g MCT chips |
50 g very low fat fromage frais | |
Glass fruit squash + glucose polymer | |
Mid afternoon | Glass MFM (100 mL) |
Meringue or Rice Krispie cake | |
Supper | 40 g white fish steak fried in MCT oil |
Fat free mashed potatoes plus 2 teaspoons Liquigen | |
40 g carrots | |
50 g very low fat ice cream | |
Jelly | |
Bedtime | Glass MFM (100 mL) |
Total LCT intake for the day 2 g |
A minimal fat milk may replace the minimal LCT formula from 1 year if nutritional intake is not compromised by doing so. This minimal fat milk can be flavoured with Nesquik powder, fruit Crusha syrups or low fat chocolate flavour topping. It can also be used on breakfast cereals, to make custard or in cereal puddings. The quantities of the ingredients may be varied to taste if needed, to provide 70–90 kcal (230–375 kJ)/100 mL. Extra energy can be added to the diet by increasing the concentration of glucose polymer in the milk or by using a 10%–15% solution of glucose polymer to make up fruit squash. Increased energy intake can be achieved by the addition of Liquigen to suitable foods such as mashed potatoes or other root vegetables.
Feeding the older child and adolescent with chylothorax
Keeping the LCT intake to a minimum necessitates the exclusion of many foods including animal and vegetable fats such as butter, lard, margarine and vegetable oils. It also makes it necessary to strictly limit or exclude protein foods that also have a high fat content such as meat, fatty fish, full fat milk, cheese and eggs. All cakes, pastry and biscuits made with LCT fats must also be avoided. An important part of the diet is skimmed milk fortified with carbohydrate and MCT as a source of energy, protein, minerals and vitamins.
MCT can be used in the diet in the form of MCT oil or MCT emulsion. MCT oil allows fried foods to be included, such as fried fish, chips and crisps. Both MCT oil and MCT emulsion can be used in baking, cakes, biscuits, pastry; all these foods are valuable sources of energy and can greatly enhance the palatability of the diet to the patient. The final diet may contain 40–70 g MCT, depending on the age and energy requirement of the patient. This should be gradually introduced over a period of 7–10 days in order to avoid abdominal discomfort. Table 13.10 lists foods containing minimal LCT, which can be used freely in the diet.
Table 13.10 Free foods for minimal LCT diet
All fruits fresh, tinned or frozen (except olives and avocado pear) |
All vegetables, fresh, tinned or frozen |
Sugar, honey, golden syrup, treacle, jam, marmalade |
Jelly and jellied sweets such as Jelly Tots, Jelly Babies, wine gums or fruit pastilles |
Boiled sweets, mints (not butter mints) |
Fruit sorbets, water ices, ice lollies |
Meringue, egg white, Rite-Diet egg replacer |
Spices and essences |
Salt, pepper, vinegar, herbs, tomato ketchup, most chutney, Marmite, Oxo, Bovril |
Fruit juices, fruit squashes, Crusha fruit flavouring syrups, Nesquik fruit flavouring powder, chocolate flavour topping, bottled fruit sauces |
Fizzy drinks, lemonade, cola, Lucozade |
The weights and fat contents of foods which can be used to construct a day’s meals containing minimal fat for the child or adolescent with chylothorax are given in Table 13.11. A review of some suitable low fat foods indicates that there may be up to a threefold variation in fat content of some meat and fish products depending on the brand. To make the diet more manageable the family can be taught to calculate minimal LCT exchanges as follows:
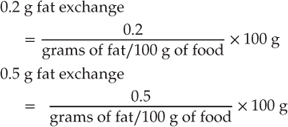
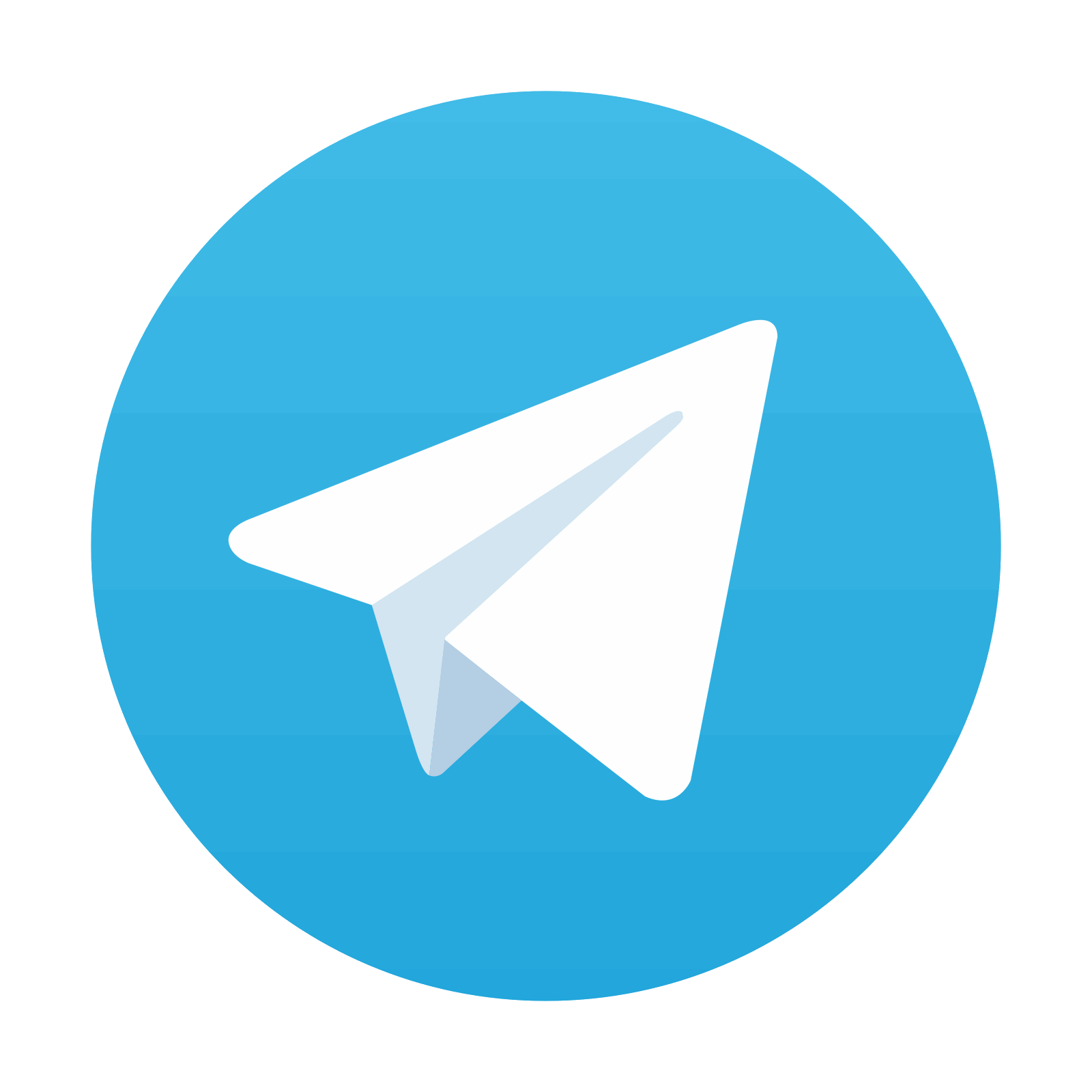
Stay updated, free articles. Join our Telegram channel

Full access? Get Clinical Tree
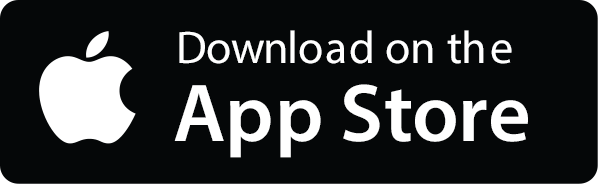
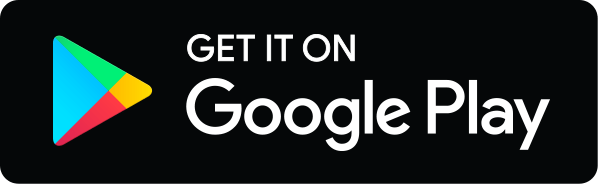