Fig. 26.1
Constitutive and activation-induced co-stimulatory members of the TNF and TNFR families expressed on T cells, NK cells, and antigen-presenting cells (APCs). Schematic representation of an immune synapse between effector T cells (Teff), regulatory T cells (Treg), and NK cells with an APC. TRAF adaptor usage and reference to the agonist monoclonal antibodies under clinical development are provided for each TNFR co-stimulatory molecule
26.2 Preclinical and Clinical Experience with Antibody Agonists of Co-stimulatory Receptors of the TNFR Family
To invigorate or reinvigorate at will an immune response in an antigen-specific fashion has been a long-term elusive goal in pharmacology. The objectives behind vaccination have been only successful in a prophylactic setting when able to elicit neutralizing antibodies to the infectious agents or their toxins prior to exposure but largely unsuccessful when the goal is to induce a protective cellular immune response. In cancer we often find the situation in which there is already an ongoing baseline immune response [27, 28]. Such immune response is often too weak as to cause the eradication of the malignancy, but multiple lines of evidence in mouse and human indicate that it is actively controlling its progression [29]. In a way there is an ongoing equilibrium [30] of immunity and cancer that mainly manifests itself in the tumor microenvironment and potentially in the tumor-draining lymph nodes. In light of these concepts, it is conceivable to pharmacologically act on receptors of immune cells present in this microenvironment scenario to decisively potentiate the ongoing baseline immune responses, empowering them to impact the malignancy. Immunology research during the late 1990s found in a serendipitous way that co-stimulation via TNFR members could lead to tumor eradication in mouse models [31, 32]. We will review knowledge on the targeting of the main receptor of this family whose effects are under clinical investigation.
26.3 CD137 (4-1BB)
CD137 (4-1BB or TNFSR9) was originally discovered on activated T lymphocytes and found to favor proliferation and IL-2 production by T cells [33, 34]. Indeed, its presence is readily detected on CD8- and CD4-activated T cells (including Tregs) following antigen-mediated activation [35]. Its expression has also been reported on activated NK cells [36], B cells, and myeloid cells [37]. Experiments in knockout mice indicate that this molecule is important for optimal antiviral and alloreactive CTL responses [38, 39] and for the induction of long-lived memory T cells as demonstrated following virus infections [40, 41].
Only one transmembrane ligand (CD137L) has been identified, and this belongs to the TNF family [42]. The ligand is termed 4-1BBL, CD137L, or TNFSF9. The system is quite conserved in mouse and human. Ligand binding induces CD137 to productively signal using TRAF2 and TRAF1 as its main adaptors [43, 44]. The extracellular domain has been found to be kept to some extent pre-cross-linked by galectin-9 bound to its carbohydrates [45]. The resulting conformational features of such lectin-CD137 complex are likely important for signaling [46].
Early signaling by CD137 involves K-63 polyubiquitination reactions dependent on TRAF2 functions that ultimately result in activation of the NF-kB and MAP kinase pathways [47]. Preliminary evidence suggests that these pathways are actively regulated by specific de-ubiquitinases. The biochemical effects of TRAF1 in these complexes are far less clear [48], but a recent involvement of TRAF1 that has been discovered in the regulation of linear ubiquitin has been proposed in the contexts of other TNF family receptors [49]. It has been reported that CD8 T cells primed in the presence of CD137 agonists become favorably poised for future encounters with antigen. This is probably explained by epigenetic changes in miRNA expression and chromatin configuration (Angela Aznar, Submitted).
Agonist anti-CD137 mAb and the trimerized natural ligand have been reported to exert antitumor effects in mouse models [31]. The therapeutic effects elicited by agonist mAbs are mediated by a strong CTL response that more efficiently destroys the malignant tissue. A requirement for NK cells in some models has been reported [36]. The functional effects of CD137 ligation on Treg cells are controversial [50].
It is remarkable that multiple mouse tumor models are amenable to treatment with agonist anti-CD137 mAb [51]. In the resistant tumors, it is easy to find combination strategies with other therapies that ultimately result in synergistic, often curative, effects. These include combinations with vaccines [52], cytokines [53], and other immune-stimulatory mAbs [54, 55]. In addition, radiotherapy and chemotherapy have also been reported to be synergistic with anti-CD137 mAb [56, 57]. Agonist anti-CD137 single-chain mAb expressed on the plasma membrane of tumor cells results in powerful localized CTL- and NK-mediated antitumor immunity [58, 59]. Recent evidence suggests that the transcription factor eomesodermin partially explains this robust CTL phenotype [60].
In the case of other TNFR family members such as CD40, cross-linking of the receptor by the agonist antibodies is dependent on CD32 Fc receptors on neighboring cells [61, 62]. However, in our hands this is not the case with CD137 as the antitumor effects are intact in Cd32 (FcRγII)−/− mice possibly because of the baseline pre-cross-linking by galectin-9.
Bivalent 4-1BB agonistic aptamers also induce antitumor effects in tumor-bearing mice [63]. This strategy has also been used in combination with a novel approach that was described to enhance tumor antigenicity, in which tumor cell nonsense mRNA-mediated decay (NMD) was inhibited with aptamer-siRNA chimeras targeted to tumor cells. The combination of 4-1BB agonistic aptamer and targeted inhibition of NMD showed a synergistic antitumor effect [64].
The cytoplasmic tail of CD137 has been used to construct chimeric antigen receptors (CARs) encompassing a single-chain antibody CD3ζ and the CD137 cytoplasmatic tail. In these constructs, the CD137 part is critical to support the persistence and expansion of the transfected T lymphocytes [65, 66]. In fact, the anti-CD19 CARs encompassing CD137 have produced the best clinical results against leukemias with long-term engraftment of the transduced T cells [67, 68].
Another intriguing aspect of CD137 co-stimulation is its largely unexplained interplay with the TCR signaling machinery on T cells and how it behaves in immune synapses [69]. Recent evidence indicates that it can rapidly internalize following ligation but that it seems to keep signaling from endosomal compartments [47].
It is a yet unsatisfactorily explained paradox that the very same anti-CD137 antibodies, which successfully treat cancer in mouse models, also ameliorate mouse autoimmune disease mediated by CD4 T cells such as EAE, lupus, and collagen-induced arthritis [70, 71]. This effect seems to depend on an overactivation of CD4 lymphocytes that ultimately die off [50]. In contrast, treatment of mice with these antibodies results in mild liver inflammation composed of periportal polyclonal infiltrates dominated by CD8 T lymphocytes [72]. Liver inflammation is dependent on CD137 (absent in CD137 KO mice), IFNγ, and TNFα. Interestingly, anti-CD137 monoclonal antibodies inhibit humoral (antibody-mediated) immunity in mice [73]. This activity is due to actions on follicular T helper cells that in human are the main CD137 baseline expressing lymphocytes in healthy volunteers [74]. Abrogation or attenuation of neutralizing antibody responses has been exploited in mouse models to permit repeated administration of tumor virotherapy together with anti-CD137 mAb [53].
On human and mouse NK cells, CD137 becomes expressed following exposure to IgG-coated target cells subjected to antibody-dependent cellular cytotoxicity [75]. This function is mediated by the CD16 Fcγ receptor. Once expressing CD137, the agonist anti-CD137 mAb strongly upregulates ADCC function capabilities for subsequent encounters. Indeed, this powerful effect produces synergistic antitumor cytotoxicity with antibodies such as anti-CD20 [75], trastuzumab [76], or cetuximab [77]. Upregulation of CD137 on NK cells infiltrating human head and neck cancer has been seen in patients treated with cetuximab [78].
Two anti-CD137 mAb antibodies are currently undergoing clinical trials. Urelumab (IgG4), developed by BMS, showed an excellent preclinical safety profile including results in cynomolgus macaques. Phase I studies of urelumab demonstrated a tolerable safety profile for doses escalated to 15 mg/kg. However, in subsequent phase II studies, several patients developed liver inflammation that resulted in a temporary halt in development and led to two fatalities [79, 80]. New phase I trials were started that identified safe doses at 0.1 mg/kg (a flat dose of 8 mg/kg every 2 or 4 weeks), and the antibody is currently undergoing further clinical evaluation in combination regimens with rituximab (NCT01775631), cetuximab (NCT02110082), elotuzumab (NCT02252263), and nivolumab (SITC meeting 2016). Urelumab monotherapy produced signs of clinical activity including objective responses in melanoma (ASCO 2014), renal cell carcinoma, and lymphoma (SITC meeting 2016), but its value is mainly foreseen to be in combination regimens.
PFZ-05082566 (utomilumab) is an IgG2 anti-CD137 and also under development after showing bioactivity in vitro assays and safety in nonhuman primates [81]. A phase I clinical trial and its expansion are ongoing with escalating doses in combination with rituximab (NCT01307267) and pembrolizumab (NCT02179918). In the phase I trial, a number of patients with virally induced Merkel carcinomas are being treated with evidence of objective clinical activity, and no signs of hepatotoxicity have been observed in the dose levels reported thus far [82, 83]. Clinical responses suggest some degrees of at least additive clinical efficacy with pembrolizumab (ASCO 2016) that warrant expansions to explore combined immunotherapy strategies.
26.4 OX40 Co-stimulation
OX40 (also known as CD134 or TNFRSF4) is a type 1 transmembrane protein that belongs to the TNFR family and was first discovered in 1987 on the surface of activated CD4+ T cells in rats [84]. The original publication showed that an anti-OX40 antibody (MRC OX40) could increase T-cell proliferation [84], and subsequent studies demonstrated that OX40 is expressed on CD4+ and also on CD8+ T cells (albeit at lower levels) 24–72 h following TCR engagement [85, 86]. Treg cells constitutively express OX40 in mice [87], but in human Treg cells, OX40 expression is upregulated upon activation [86]. Other cells belonging to the innate immune system such as NK, NKT cells, or neutrophils can express OX40 as well [88]. Stimulation in these cells via OX40 has shown a pro-inflammatory and pro-survival effect, suggesting that OX40 modulation contributes not only to adaptive but also to innate immune responses [88, 89].
OX40 ligand (OX40L, CD252) is predominantly expressed on activated APCs, but other hematopoietic (NK, mast, and activated T cells) and non-hematopoietic cells (smooth muscle, vascular endothelial cells) can express OX40L as well [90, 91]. OX40 ligation is known to recruit TRAF2 and TRAF3 to the intracellular domain of OX40, leading to activation of both the canonical and noncanonical NF-κβ pathways which ultimately induce the expression of pro-survival molecules and increase cytokine production associated with enhanced T-cell expansion, differentiation, and the generation of long-lived memory cells [92, 93]. The importance of noncanonical pathway needs further studies, comparing OX40 co-stimulation properties with other TNFR members.
The co-stimulatory nature of OX40 has been confirmed in autoimmune mouse models where blocking OX40/OX40L interactions diminishes the clinical signs of autoimmunity [94]. By contrast, overexpression of the OX40L in transgenic mice leads to increased signs of autoimmunity [95]. Additionally, in patients, OX40L expression is upregulated in hosts with autoimmune diseases, and the majority of OX40L expression appears to be confined to the lesions, suggesting that OX40L expression is the limiting factor in regard to OX40 signaling in T cells [96]. Based on these findings, and preclinical evidence that OX40 engagement can expand T-cell populations and increase effector functions, OX40 agonists (mAbs and soluble forms of OX40L) have been investigated for cancer immunotherapy.
In preclinical tumor models, OX40 monotherapy with agonist mAbs was shown to be effective in eradicating primarily immunogenic tumors including MC303 sarcoma, CT26 colon carcinoma, SM1 breast cancer, and small B16 melanoma, among others [97–99]. However, OX40 failed to provide adequate antitumor immunity in poorly immunogenic tumors [96]. Therefore, different combinatorial strategies have been explored to increase OX40 agonist antitumor efficacy. In line with this, combinations with cytokines such as interleukin 12 (IL-12) or IL-2 have been successfully tested with or without vaccination [100, 101]. Furthermore, based on the experience that stimuli signaling through Toll-like receptors (TLR) induce OX40L expression on APCs, combination of TLR agonists with OX40 co-stimulation-based strategies has demonstrated synergistic effects [102, 103]. In addition, anti-OX40 antibodies have been combined with other immunostimulatory monoclonal antibodies to treat lymphomas, sarcomas, hepatic colon metastasis, and spontaneous hepatocellular carcinoma [55, 104–106]. Combining OX40 agonist with cancer vaccines, chemotherapy or radiation has also demonstrated an improvement in control of established tumors [107, 108] and enhanced expansion and prolonged survival of tumor-specific T cells [109].
It is believed that one of the main advantages of targeting OX40 is the abrogation of Treg-mediated immunosuppression by inhibition of the activity of Treg cells [110] or by direct depletion of these immunosuppressor cells [87, 111, 112]. Thus, combination of anti-OX40 mAbs with cyclophosphamide (chemotherapy that is capable of partially depleting Treg cells) showed a synergistic effect in a poorly immunogenic B16 murine melanoma tumor and was associated with a reduction of Treg at the tumor site [107]. Another mechanism reported to be associated with OX40 stimulation is the ability of OX40 engagement to trigger cytolytic activity in CD4+ T cells. Data in preclinical models show that anti-OX40 mAb is synergistic in combination with anti-CD137 [113] or in combination with cyclophosphamide and adoptive T-cell therapy [114], resulting in an increase in cytotoxic activity and in a preferential Th1 polarization.
Clinical development of OX40 agonists started in 2006 with a murine antihuman OX40 (anti-hOX40) mAb. This murine antibody was first tested in nonhuman primates [115], and subsequently 30 patients with solid tumors received three doses (days 1, 3, and 5) from 0.1 to 2 mg/kg in the first-in-human clinical trial [116]. The treatment was very well tolerated, and no maximum tolerated dose was identified. Even though slight tumor size reductions were observed in some patients, none of the patients showed an objective response by RECIST criteria. Elevated levels of neutralizing human anti-mouse immunoglobulin antibodies were considered the most important limitation in this clinical trial. Nonetheless, combinations of the murine anti-hOX40 with chemotherapy and/or radiotherapy in clinical trials are currently ongoing in different solid tumors (Table 26.1). The obvious next step was the development of fully human OX40 agonists to allow repeated dosing in cancer patients. Human Fc:OX40L has demonstrated promising results in preclinical development [117], and clinical testing may begin in the near future. Two humanized anti-OX40 mAbs, MEDI6383 (AgonOx, AstraZeneca) and MOXR0916 (Genentech, Roche), are now undergoing clinical development (Table 26.1) as single agents and in combination with PD-1 and PD-L1 blocking agents.
Table 26.1
Target | Name/alternative names (company) | Clinical trials.gov identifier | Phase | Disease | Single agent/combination |
---|---|---|---|---|---|
CD137 | Urelumab/BMS-663513 (Bristol-Myers) | NCT00309023 | I–II | Metastatic or locally advanced solid malignancies | Monotherapy |
NCT00351325 | I | Advanced solid malignancies | With chemotherapy | ||
NCT00612664 | II | Stage III–IV melanoma (2° line) | Monotherapy | ||
NCT00461110 | I | Non-small cell lung cancer | With chemoradiation | ||
NCT00803374 | I | Unresectable stage III–IV melanoma | With ipilimumab | ||
NCT02652455 | Pilot clinical | Metastatic melanoma | With nivolumab and ACTa | ||
NCT02658981 | I | Recurrent glioblastoma | Monotherapy or combination with anti-PD-1 | ||
NCT01775631 | I | B-cell malignancies | With rituximab | ||
NCT02420938 | II | Relapsed, refractory, or high-risk untreated patients with chronic lymphocytic leukemia. Small lymphocytic lymphoma | With rituximab | ||
NCT02845323 | II | Patients with cisplatin-ineligible muscle-invasive urothelial carcinoma of the bladder | With nivolumab | ||
NCT02110082 | I | Colorectal cancer and head and neck cancer | With cetuximab | ||
NCT02252263 | I | Multiple myeloma | With elotuzumab | ||
Utomilumab/PF-05082566 (Pfizer) | NCT02951156 | Ib/III | Diffuse large B-cell lymphoma | With avelumab/rituximab/azacitidine | |
NCTO1307267 | I | Advanced solid tumor/lymphoma | Monotherapy (solid tumors) and with rituximab (lymphomas) | ||
NCT02179918 | I | Advanced solid tumor | With pembrolizumab | ||
OX-40 | MEDI6469 (MedImmune) | NCT01644968 | I | Advanced cancer | Combination with KLHb or tetanus vaccine |
NCT01303705 | Ib | Progressive or metastatic prostate cancer after systemic therapy | With cyclophosphamide and radiation | ||
NCT01689870 | I/II | Unresectable or metastatic melanoma | With ipilimumab | ||
NCT01416844 | II | Metastatic melanoma | Monotherapy | ||
NCT02274155 | Ib | Locoregionally advanced, oral head and neck squamous cell carcinoma | Monotherapy | ||
NCT02559024 | I/IB | Metastatic colorectal cancer | With surgery/RFAc of metastases | ||
NCT01862900 | I/II | Progressive metastatic breast cancer | With stereotactic body radiation therapy | ||
NCT02205333 | Ib/II | Selected advanced solid tumors or aggressive B-cell lymphomas | Monotherapy or combined with durvalumab, tremelimumab, or rituximab | ||
MEDI6383 (AstraZeneca) | NCT02221960 | I | Selected locally advanced or metastatic carcinomas | Alone and in combination with MEDI4736 | |
MOXR0916 (Roche) | NCT02410512 | Ib | Metastatic or locally advanced solid malignancies | Atezolizumab with or without bevacizumab | |
PF-04518600 (Pfizer) | NCT02315066 | I | Selected locally advanced or metastatic carcinomas | Alone and in combination with utomilumab | |
INCAGN01949 (Agenus) | NCT02923349 | I/II | Advanced or metastatic solid tumors | Monotherapy | |
GSK3174998 (GlaxoSmithKline) | NCT02528357 | I | Selected advanced solid tumors | Monotherapy or with pembrolizumab | |
MEDI0562 (MedImmune LLC) | NCT02705482 | I | Selected advanced solid tumors | Combination with tremelimumab or durvalumab | |
GITR | TRX518/Anti-TNFRSF18 (Tolerx) | NCT01239134 | I | Unresectable stage III or stage IV malignant melanoma or other solid tumor malignancies | Monotherapy |
MEDI1873 (MedImmune LLC) | NCT02583165 | I | Selected advanced solid tumors | Monotherapy | |
GWN323 (Novartis) | NCT02740270 | I/Ib | Advanced solid tumors and lymphomas | Monotherapy or combination with anti-PD-1 | |
INCAGN01876 (Agenus) | NCT02697591 | I/II | Advanced or metastatic solid tumors | Monotherapy | |
CD27 | Varlilumab/CDX-1127 (Celldex Ther.) | NCT02335918 | I/II | Squamous cell carcinoma of the head and neck, ovarian carcinoma, colorectal cancer, renal cell carcinoma, glioblastoma | With nivolumab |
NCT01460134 | I | Selected refractory or relapsed hematologic malignancies or solid tumors | Monotherapy | ||
NCT02270372 | Ib | Advanced ovarian cancer or breast cancer | With ONT-10 | ||
NCT03038672 | II | Relapsed or refractory aggressive B-cell lymphomas | With nivolumab | ||
NCT02413827 | I/II | Patients with unresectable stage III or stage IV melanoma | Combination with ipilimumab and CDX-1401 | ||
NCT02386111 | I/II | Metastatic clear cell renal carcinoma | Combination with sunitinib | ||
NCT02924038 | Pilot study | WHO grade II low-grade glioma | IMA950 vaccine plus poly-ICLC |
26.5 GITR
Glucocorticoid-induced TNFR-related protein (GITR, TNFSFR18, CD357) belongs to the TNFR family. It was originally discovered in murine T-cell hybridomas treated with dexamethasone [118] thus explaining its name. Further studies concluded that glucocorticoid treatment has no effect on GITR expression in humans (and is not necessary in mice). In contrast, its expression is induced after TCR engagement in CD4+ and CD8+ T cells [119, 120]. GITR is expressed at low levels on resting CD4+ and CD8+ T cells, and it is upregulated 24–72 h after TCR engagement and remains expressed on the lymphocyte surface for several days. In contrast, Treg cells constitutively and brightly express GITR [121], where it is thought that GITR exerts an inhibitory activity on Treg suppressive functions [122]. Additionally, GITR expression has been found on NK cells, eosinophils, basophils, macrophages, and B cells, particularly upon activation [123].
GITR ligand (GITRL), as was the case with OX40L, is highly expressed on activated APC and endothelial cells. Upon ligation, GITR downstream signaling is exerted through a complex consisting of a single TRAF5 and two TRAF2 proteins, leading to NF-κβ and MAPK pathway activation [124]. GITR-mediated co-stimulation ultimately enhances T-cell proliferation and effector functions in part because of upregulation of IL-2Rɑ, IL-2, and IFNɣ [120, 125]. GITR ligation protects T cells from activation-induced cell death (AICD), leading to an increase in memory T cells. Initial studies demonstrated that GITR ligation could potentially overcome tolerance to self- and tumor-antigens, making it an attractive target for the development of cancer immunotherapies [126]. Subsequently, an antitumor effect of GITR stimulation has been demonstrated in different tumor models with an agonist anti-mGITR antibody (rat monoclonal DTA-1) or GITRL manipulation. DTA-1 has been shown to be effective in treating 8-day established Meth-A sarcomas [127], CT26 [128], and small-established B16 tumors [129]. Additionally, GITR agonists have demonstrated a synergistic antitumor effect when combined with vaccines [129, 130], TLR agonists [131], and other immunostimulatory monoclonal antibodies such as anti-CTLA-4 [132]. Recombinant forms of GITRL have also been successfully employed by means of DCs engineered to secrete a soluble GITRL fusion protein [133].
Different mechanisms might contribute to the antitumor effects of GITR modulation. Perhaps the best defined is the action of GITR agonists in the abrogation of Teff cell suppression by Treg cells. Some reports have shown that GITR agonists increase Teff function in the tumor microenvironment by directly targeting Treg cells impairing Treg expression of FoxP3 and consequently abrogating Treg suppressive function [129] or by targeting antigen-specific CD8+T, augmenting its resistance to Treg suppression [134, 135]. More recently it has also been reported that anti-GITR mAb (DTA-1) might directly deplete intratumor Tregs by activating myeloid cells through FcγRs as part of its mechanism [136]. In support of this hypothesis, most of the studies find increases in Teff/Treg ratios at the tumor site after treatment with DTA-1 that correlate with therapeutic benefit [129, 134, 135].
Several humanized agonist antihuman GITR mAbs are in clinical development (Table 26.1). TRX518 has been developed by Tolerx Inc. (now GITR Inc.), and a dose-escalation phase I clinical trial is ongoing this mAb at Memorial Sloan Kettering Cancer Center and other centers. More antibodies on the same specificity have also started clinical trials INCAGN01876 (Incyte) and MEDI1873 (Medimmune), and results are eagerly awaited (Table 26.1).
26.6 CD27
This molecule belongs to the TNFR family. A unique feature of CD27 among TNFR family members is its constitutive expression at significant levels on the majority of T cells (chiefly including naive T cells) [137, 138]. CD27 is also expressed on plasma cells, for which it is broadly accepted as a marker [139]. Due to the constitutive expression pattern of CD27, the expression of its only known ligand, CD70, has to be tightly regulated. In fact, CD70 is only transiently expressed on activated APC, T cells, and NK cells under physiological conditions [40, 140]. However, constitutive expression of CD70 has been documented during chronic inflammation [141].
The use of CD27 as a target in cancer immunotherapy is compromised by the inhibitory and co-stimulatory mechanisms related to the CD27-CD70 pathway in the different immune contexts. CD27 ligation by CD70 recruits TRAF2 and TRAF5 to the intracellular domain of CD27, activating the NF-κβ and c-Jun pathways, and, as a consequence, promotes cell survival, enhances T and B cell expansion, and increases effector functions [142]. By contrast, CD27 signaling has also been reported to lead to T-cell dysfunction in a context of a CD70 continuous expression, which can happen in the context of a chronic infection such as in the LCMV chronic infection model, in which blocking of the CD70/CD27 interaction has been shown to help to eradicate the infection [141].
Constitutive expression of CD70 has been documented in cancer [143]. It has been observed that constitutive CD70 expression on tumors or APCs improves antitumor immunity in murine lymphoma models, enhancing NK-mediated rejection [144]. In line with this observation, the administration of an agonistic anti-CD27 antibody has been shown to protect against intravenous injection of two different lymphoma cell lines [145] and to delay the growth of B16 subcutaneous melanoma [146]. However, intact CD27/CD70 signaling has been associated with decreased antitumor immune response and an increase in intratumoral Treg cells [147].
All in all, it seems that the effects of CD27 may depend on both the tissue context in which CD70 is expressed and also the duration of CD27-CD70 ligation. Clinical development of CD27-related agents will require a precise triggering of certain molecules in selected environments to avoid exaggerating tumor-induced immunosuppression. A fully human agonist anti-CD27 monoclonal antibody, CDX-1127 (Celldex Therapeutics, Inc., Needham, MA, USA), is being evaluated in clinical trials in selected hematological and solid tumors (Table 26.1).
26.7 Future Perspectives
The clinical success of ipilimumab [148], anti-PD1 [149, 150], and anti-PD-L1 [150–152] mAbs has validated the principle that modulation of the immune response can overcome immune evasion mechanisms of tumors and produce objective antitumor responses. However, different mechanisms to evade immune attack can be developed by each patient’s tumor, and now the challenge is to determine which mechanism is dominant and which is the most suitable individualized immunotherapy. Clinical experience has revealed that not all patients are sensitive to the CTLA-4 blockade or PD-1/PD-L1 pathway blockade. Elucidating the mechanisms that explain this primary resistance [153] and achieving a better understanding of the heterogeneity among patients’ tumor immune infiltration are essential if we are to implement personalized medicine and establish logical rationales for combination in the cancer immunotherapy arena.
Combination of blocking co-inhibitory molecules (so called checkpoint blockade) and co-stimulatory mAbs seems to be the next most promising approach following CTLA-4 and anti-PD-1 combinations [154]. In line with this, clinical trials combining anti-PD-1 and anti-CD137 have been conducted (NCT02253992 and NCT02179918). Because CD137 is expressed on activated NK cells through FcγRs-Fc binding, the combination of anti-CD137 mAbs with anti-EGFR, anti-CD20, or anti-HER-2 mAbs has been successfully tested in mouse tumor models, and phase I clinical trials in lymphoma, head and neck, and colorectal cancer patients are ongoing (NCT01307267, NCT01775631). Combinations of different TNFRSF members have been successfully tested in preclinical models [55, 113], but concerns exist regarding translation of this approach to the clinical setting due to the toxicity profile of agonist immunostimulatory monoclonal antibodies (ISMAB) [155]. Thus, different strategies focusing on more specific delivery of the antibodies to the tumor site or intratumoral administration in accessible lesions [156–159] are being proposed to overcome this limitation. Agonist monoclonal antibodies targeting co-stimulatory receptors of T and NK cells are definitively part of the new wave of immunotherapies, which are currently changing treatment of cancer paradigms.
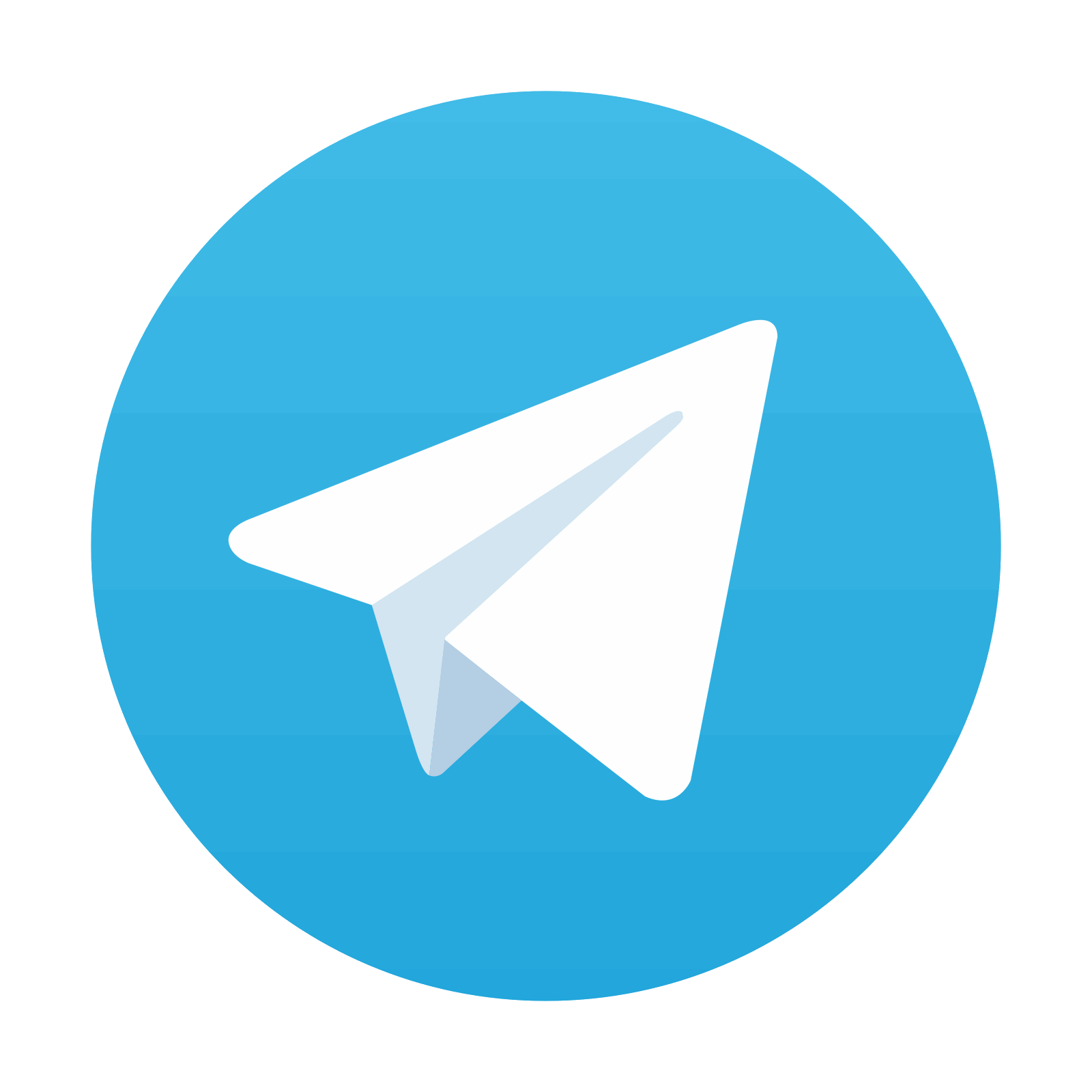
Stay updated, free articles. Join our Telegram channel

Full access? Get Clinical Tree
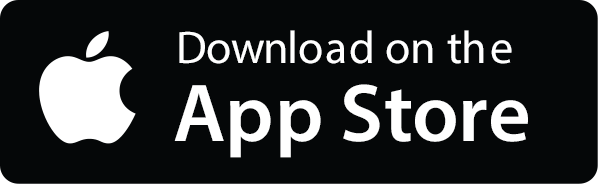
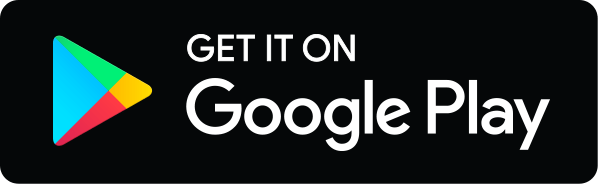