NCI risk (age/WBC)
Favorable genetics
Unfavorable characteristics
Day 8 PB MRD (%)
Day 29 marrow MRD (%)
% of B-cell patients
5-year EFS (%)
Low risk
SR
Yes
None
<0.01
<0.01
15
>95
Average risk
SR
Yes
None
≥0.01
<0.01
36
90–95
SR
No
None
<1
<0.01
High risk
SR
Yes
None
Any level
≥0.01
25
88–90
SR
No
None
≥1
<0.01
HR (age < 13 years)
Any
None
Any level
<0.01
Very high risk
SR
No
None
Any level
≥0.01
24
<80
HR
Any
None
Any level
≥0.01
HR (age ≥13 y)
Any
None
Any level
<0.01
SR or HR
Any
Yes
Any level
Any level
Special group
T-cell ALL
n/a
66–80
17.4.1 Cytogenetics
More than 60% of pediatric ALL cell lines have genetic abnormalities. Specific cytogenetic abnormalities have important prognostic value in ALL. Chromosomal translocations occur in approximately 15% of pediatric ALL. The oldest known is the t(9;22) BCR-ABL translocation that forms the Philadelphia chromosome. This translocation is found in up to 5% of B-cell precursor ALL and is associated with a higher risk of CNS involvement and overall poorer prognosis.
The t(12;21) translocation leads to the TEL-AML1 fusion gene and can be found in about 22% of cases. About 6% of ALL patients have the t(1;19) translocation that creates the E2A-PBX1 fusion gene. Rarer genetic abnormalities include the t(4;11) translocation associated with African-American children who have high WBC counts, the t(8;14) MYC defect in cases of mature B-cell ALL (2%), and t(11;14) associated with T-cell ALL with extramedullary involvement.
RUNX1 and ETV6 gene are juxtaposed in utero in TEL-AML1 containing cryptic t(12,21) translocation found in 15% of patients can lead to impaired hematopoietic differentiation. The MLL rearrangement tends to be found in infants and is associated with poor prognosis; it can arise from t(4;11), t(11;10), or t(9;11) translocations.
Twenty-five percent of ALL have hyperdiploid chromosomal changes and is associated with a more favorable outcome. The most common are trisomies 4, 10, and 17. Conversely, hypodiploidy of fewer than 45 chromosomes conveys a more unfavorable outcome.
One of the most recently identified genetic abnormalities is the IKZF1 deletion found in 15% of precursor B-cell ALL cases. These are associated with older patients who have higher WBC count at diagnosis, and, as such, are also associated with a high-risk prognosis. IKZF1 deletions are also associated with higher chance of a BCR-ABL1 abnormality (van der Veer et al. 2013; Dorge et al. 2013).
CRLF2 overexpression and JAK mutations are also associated with IKZF1 deletion and poorer prognosis. A CRFLF2 abnormality has been found to independently confer worse prognosis (Chen et al. 2012).
AML subtypes based on hematopoietic lineage in the French-American-British (FAB) classification system still have some practical usage and have distinct molecular correlations (Table 17.2).
Table 17.2
French-American-British (FAB) classification of acute myelogenous leukemia (AML) correlated with common cytogenetic abnormalities
FAB type | Cytogenetic finding |
---|---|
M0, M1 (Undifferentiated AML) | trisomy 11, t(10;11) |
M2 (Acute myeloid leukemia) | t(8;21) |
M3 (Acute promyelocytic leukemia) | t(15;17), t(11;17), t(5;17) |
M4 (Acute myelomonocytic leukemia with eosinophilia) | inv(16) |
M5 (Acute monocytic leukemia) | t(11;23) |
M6 (Acute erythroleukemia) | t(3;5) |
M7 (Acute megakaryocytic leukemia) | t(3;3), t(3;12) |
17.5 Acute Management
Aside from cranial neuropathies and leptomeningeal disease, pediatric CNS leukemias rarely cause medical emergencies due to mass effect in CNS space. In cases where neurologic symptoms do arise, glucocorticoids can serve an important part of acute management along with cranial radiation. The use of glucocorticoids can cause rapid leukemia or lymphoma cell lysis, which can hinder diagnostic workup in undiagnosed patients. In other parts of the body, mediastinal adenopathy can cause airway compression, and may sometimes be amenable to low-dose palliative RT (e.g., 4–6 Gy in 2–3 fractions).
AML patients can rarely develop neurologic symptoms from hyperleukocytosis. A small Robert Wood Johnson medical center study showed that whole brain radiation for 1–3 fractions to a total dose of 2–9 Gy lead to resolution of neurologic symptoms in majority of patients (Ferro et al. 2014).
17.6 Treatment
17.6.1 General Principles
Investigation into combined chemoradiation treatments for ALL began in the 1960s. The earliest chemotherapy trials led to significant improvement in survival rates but still had high CNS relapse rates. Subsequent addition of prophylactic cranial radiation significantly decreased CNS relapse. Clinical trials in the 1970s established that prophylactic cranial irradiation (PCI) was effective in conjunction with intrathecal chemotherapies and certain systemic therapies to control leukemic burden in the entire subarachnoid space, meninges, and CNS parenchyma. RT to the entire craniospinal axis is only required in setting of high-risk CNS relapse. Overall, the evolution of ALL treatments is a paradigm for how iterations of clinical trials can over time significantly improve outcomes, particularly with attention to matching therapy to prognostic factors. Most patients with ALL no longer require PCI. Patients who still require PCI receive lower radiation doses in combination with intensification of the systemic and intrathecal therapies. PCI doses for pediatric ALL are often 12 Gy (depending on the specifics of the systemic therapy program used) while overt CNS involvement with active chemotherapy often uses 18 Gy cranial irradiation.
In the evolution of ALL therapy, the specific phases of treatment include induction (of remission), consolidation, and post-remission therapies. Consolidation therapy includes CNS-directed therapy that may involve PCI depending on risk factors and the particular treatment protocol being followed. The optimal timing of PCI is not clear, but has generally been scheduled to minimize interactions with other chemotherapy agents, particularly high-dose methotrexate. Regardless, intrathecal chemotherapy is important. Post-remission therapy may include intensification and delayed intensification therapies. In addition, there is typically a protracted phase of low intensity maintenance chemotherapy which is delivered over several years once a firm remission is established.
The mainstay of ALL treatment is induction chemotherapy with the goal of rapid complete remission. The typical regimen is: dexamethasone, vincristine, and asparaginase. Additional daunorubicin as well as targeted tyrosine kinase inhibitor for Philadelphia chromosome positive disease are sometimes also included in induction regimen. Induction therapy typically lasts 4–6 weeks and leads to complete remission in over 90% of cases. The systemic steroid of choice is dexamethasone as several studies have shown that dexamethasone reduces CNS and systemic relapse in pediatric ALL compared to prednisone due to better CNS penetration (Bostrom et al. 2003; Jones et al. 1991).
Bone marrow biopsy at 2–4 weeks of induction therapy is essential in looking for residual lymphoblast burden as a marker of prognosis, chemotherapy sensitivity, and guide for further therapy. The finding of residual blasts constitutes minimal residual disease (MRD) designation and confers a worse prognosis (van Dongen et al. 1998). An associated molecular marker for a patient’s ALL improves the prognostic accuracy of MRD. Correspondingly, rapid response to induction treatment confers better prognosis compared to a slower induction of molecular remission (Gaynon et al. 1997). See Fig. 17.1.
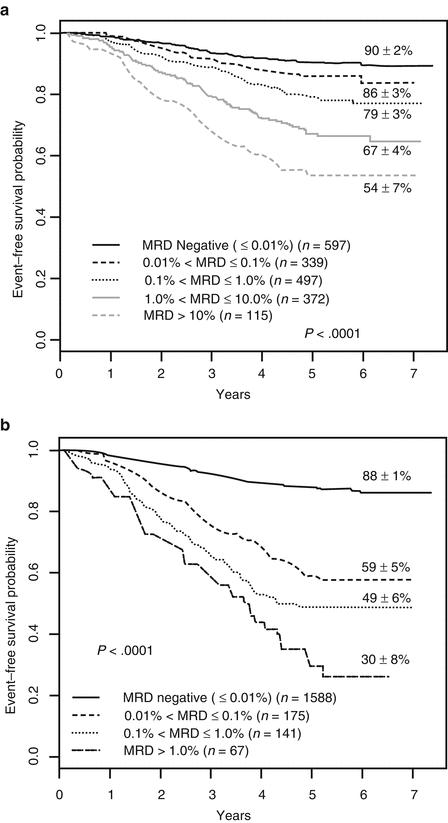
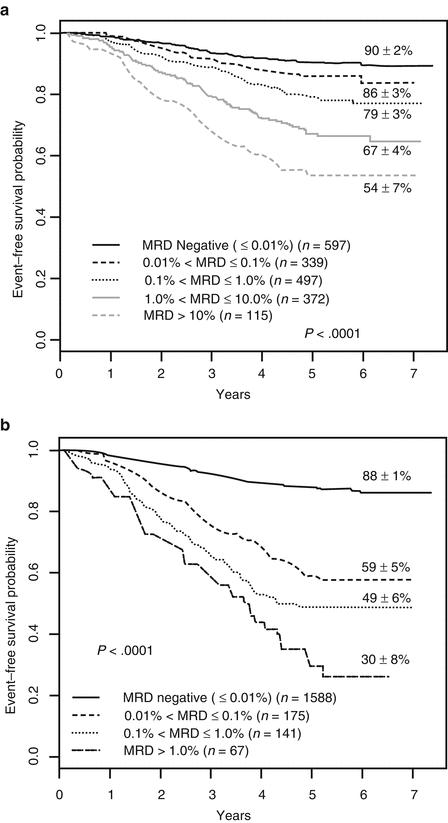
Fig. 17.1
The prognostic importance of minimal residual disease (MRD) after induction chemotherapy for ALL. From Borowitz M J et al. Blood 2008;111:5477-5485. Panel (a): EFS of ALL patients as a function of level of day-8 PB MRD. Panel (b): EFS of ALL patients as a function of level of day-29 marrow MRD
Consolidation after remission from induction therapy is tailored to prognostic factors and most commonly used chemotherapy using methotrexate alone, or with 6-mercaptopurine, asparaginase, and etoposide (or teniposide), or a combination of doxorubicin, 6-thioguanine, asparaginase, vincristine, ifosfamide, teniposide, or etoposide, and dexamethasone. There has been a push in recent decades to strengthen post-induction intensification regimens. Indeed, COG analysis of intensification trials have considered more aggressive treatments, including Ara-C, high-dose methotrexate, or l-asparaginase and have shown selected benefits (Schrappe et al. 2000a). Some cooperative groups have showed that a delayed intensification regimen after a period of maintenance therapy leads to improved outcomes in high-risk patients (Schorin et al. 1994). A recent multinational European trial, however, showed that further strengthening of delayed intensification regimens based on risk factors did not lead to improved outcomes at 5 years (Stary et al. 2014).
Recent examples of attempts to intensify post-induction chemotherapy include a 2013 trial of 111 high-risk patients who received three new chemotherapy blocks and then received allogeneic transplantation or further chemotherapy (Marshall et al. 2013).
Maintenance therapy for 2–3 years is given to nearly all patients without mature B-cell disease and is typically weekly methotrexate and daily oral 6-mercaptopurine, with pulses of vincristine and dexamethasone, and intermittent intrathecal methotrexate as well. Some protocols also include repeat induction 4 months into continuation therapy. In general, males require longer maintenance therapy than females for reasons that are unclear.
Consideration for allogeneic stem cell transplant is taken especially with minimal residual disease or when high-risk features, such as BCR-ABL, are present. The recently published BFM prospective study of allogeneic stem cell transplant in high-risk children after achievement of remission showed those receiving grafts from HLA identical siblings had similar relapse rates and mortality but faster engraftment rates and fewer acute side effects than those receiving transplantation from HLA-matched unrelated donors (Peters et al. 2015). Total body irradiation along with cyclophosphamide is a frequent conditioning regimen prior to allogeneic transplantation.
Relapsed ALL is generally treated with a second induction chemotherapy regimen consisting of a combination including vincristine, prednisone, l-asparaginase, an anthracycline with or without MTX, VP-16 or teniposide, and cytarabine. Intrathecal chemotherapy is also given along with radiotherapy if there is evidence of CNS disease upon relapse. CNS relapse even if initially isolated is treated with systemic and intrathecal therapy with the addition of CNS radiotherapy directed at the brain and selectively at the spine as well.
Second remission is expected in 70–90% of patients, and treatment for second relapse typically then involves either allogeneic transplantation or consolidative chemotherapy. Testicular relapses are now rare with improved systemic treatments and testicular irradiation is typically only indicated in cases of testicular relapse.
17.6.2 Role of RT: Initial Therapy
Combined treatment regimens first developed in the 1960s showed effectiveness of prophylactic cranial-spinal irradiation to reduce CNS relapse (Dahl et al. 1978). The earliest St. Jude Children Research Hospital (SJCRH) trials, Total V, VI, and VII, all incorporated either prophylactic craniospinal irradiation or cranial irradiation and lead to observation of reduced CNS relapse rates. For instance, CSI to a dose of 24 Gy in 15 fractions reduced isolated CNS relapse from 48–64% in SJCRH IV to 8% (Simone 1981). Similarly, the CNS relapse rate decreased from 67 to 4% in SJCRH studies V and VI, which utilized CSI to a dose of 24 Gy in 15–16 fractions (Aur et al. 1973). Total VI trial randomized pediatric patients after hematologic remission to CSI or salvage treatment after CNS relapse and noticed that 24 Gy CSI lead to increased event-free survival. SJCRH trial VII was the first to include intrathecal methotrexate and to directly compare craniospinal irradiation to cranial radiation only; the CNS relapse were comparable, approximately 8% (Simone 1981).
SJCRH study VIII patients received 24 Gy plus IT MTX, and then received either weekly IV MTX, oral MTX, 6-MP, or oral MTX, 6-MP, and cyclophosphamide, or everything plus Ara-C. The CNS relapse rates were 1.5–20% but 55% of patient in the weekly IV MTX and cranial radiation group developed leukoencephalopathy. Therefore, oral MTX and 6-MP after cranial irradiation along with IT MTX became the standard regimen. Furthermore, it is now accepted that intravenous MTX and cranial irradiation should not be given in close temporal proximity given concern for leukoencephalopathy (Aur et al. 1973).
The long-term side effects of prophylactic cranial RT in pediatric patients led to increasing attempt to use intrathecal chemotherapy and/or intermediate or high-dose IV MTX to replace cranial RT. Disease control in all but highest risk subsets have been comparable.
Several trials comparing cranial RT versus high-dose MTX along with IT chemotherapy have shown that patients with low- or standard-risk ALL without cranial radiation can have a CNS relapse rate roughly around 5% or less if IT MTX is used (Conter et al. 1997; Mahoney et al. 1998). A large meta-analysis in 2003 of 65 randomized trials of pediatric ALL patients showed that intrathecal MTX lead to comparable outcomes as cranial radiotherapy (Clarke et al. 2003). Furthermore, this meta-analysis saw that addition of intravenous MTX improved 10-year event-free survival from 62 to 68% and decreased rates of systemic relapse.
Dose reduction studies of cranial irradiation at 18 Gy have generally shown and equivalent outcome compared to 24 Gy (Nesbit et al. 1981). The CCSG trial 101 from the 1970s (when less intensive therapy for ALL unguided by modern prognostic factor tailoring of therapy) helped to further define volume of radiotherapy for CNS treatment. This large randomized trial showed that 24 Gy CSI with or without supplemental 12 Gy to liver, spleen, kidneys, or gonads for 12 Gy was not superior to 24 Gy cranial RT with intrathecal therapy although IT chemo alone was inferior to any CNS irradiation volume (Nesbit et al. 1982). German BFM studies have focused on optimizing cranial irradiation dose with high-dose methotrexate, including doses 0–12 or 18 Gy (Buhrer et al. 1990; Schrappe et al. 2000b). The St. Jude group has been very concerned about late effects of cranial RT in ALL patients such that their latest trials have completely removed all upfront use of PCI even for high-risk patients (Pui et al. 2009).
More modern trials have demonstrated that standard-risk ALL may be treated without prophylactic cranial irradiation. The risk factors that appear to benefit the most from cranial irradiation are T-cell ALL patients, WBC on presentation >100K (Conter et al. 1997). Some Pediatric Oncology Group (POG) trials have treated T-cell ALL patients with poorer risk features on protocols without prophylactic cranial radiation; there was adverse CNS relapse rates, with the 3-year CNS relapse rate of 18% without cranial radiation compared to 7% with cranial radiation (Laver et al. 2000).
In the minority of patients who present with detectable CNS leukemic involvement, these patients are managed as high-risk patients and are treated with cranial radiotherapy. The historical use of cranial-spinal irradiation used a cranial dose of 24 Gy and spinal dose of 6–15 Gy. However, CSI has been generally replaced by cranial radiation with high-dose intravenous chemotherapy thought to have good brain penetration (Cherlow et al. 1996). The survival rates for patients with detectable CNS disease at presentation have improved over the decades to as high as a 5-year survival rate of 70%. Doses of cranial radiation now range typically from 18 to 24 Gy. One interesting approach to dosing was the ALL-BFM 90 trial which used age-based doses for patients with CNS-3 disease: no RT for those <1 year old age, 18 Gy for 1–2 years, and 24 Gy for older than 2 years (Schrappe et al. 2000b). Modern cooperative group trials including within the Children’s Oncology Group (COG) that use a BFM-type systemic therapy generally employ 18 Gy for patients with CNS3 disease.
There have been several trials with small numbers of patients evaluating the deletion of cranial RT in CNS3 disease. The Dutch Childhood Oncology Group (DCOG) and the European Organization for Research and Treatment of Cancer (EORTC) have omitted cranial radiation by also using more high-dose methotrexate during post-induction consolidation and by an increased frequency of intrathecal chemotherapy. The SJCRH study also included higher cumulative doses of anthracycline than on COG trials, and frequent vincristine/dexamethasone pulses and intensified dosing of l-asparaginase, while the EORTC trials included multiple doses of high-dose Ara-C, during post-induction treatment phases for CNS3 patients. On the DCOG-9 trial, CNS3 patients treated without cranial radiation had a 5-year EFS of 67%. On the EORTC trial, the 8-year EFS of CNS3 patients treated without cranial radiation was 68%. The cumulative incidence of isolated CNS relapse for those patients was 9.4%. This CNS3 subgroup is relatively uncommon and further studies will be required to see if CNS irradiation can be safely eliminated contrary to current standard of care (Sirvent et al. 2011; Veerman et al. 2009; Pui et al. 2009). Arguably, CNS3 patients treated without cranial irradiation have inferior results and RT should not be deleted.
Additional considerations are cranial or cranial-spinal boost for patients undergoing TBI typically in preparation for bone marrow transplantation. A recent Stanford retrospective study found that pediatric patients undergoing TBI for stem cell transplant also tolerated sequential CSI to bring the cumulative cranial median dose of 24 Gy without significant long-term cognitive deficits (Hiniker et al. 2014).
Patients with T-cell ALL with few exceptions are considered at high risk, including risk for CNS relapse. As such, most modern ALL treatment schemes for T-cell ALL include cranial irradiation. An analysis of T-cell ALL patients treated within several Pediatric Oncology Group (POG) protocols suggested that omitting cranial radiation had an adverse impact on CNS relapse rates. Specifically, the 3-year CNS relapse rate was 18% for those who did not receive RT compared to 7% who did (Laver et al. 2000). But in a subgroup of favorable T-cell ALL patients defined as those with young age and low WBC at initial presentation, it may be safe to omit cranial radiation (Conter et al. 1997). Most T-cell ALL patients present with high WBC counts, however. A retrospective comparison of the AIEOP-91 trial with the BFM-90 trial in which similar backbone chemotherapy was used for T-cell ALL, differed principally in the use of cranial RT. The AIEOP-91 trial omitted cranial RT albeit with more IT chemotherapy, leading to a significantly higher CNS relapse along with a 3-year event-free survival of 62% compared to 88% for the BFM-90 patients who received cranial RT. Multivariate analysis showed that WBC >100,000 in particular defined a group of T-cell ALL patients who should receive cranial RT (Conter et al. 1997).
17.6.3 Role of RT: Relapsed ALL
In decades past, CNS relapse was thought to have a poor prognosis with disease control in the 25–50% range (Kun et al. 1984). More recent trials that utilize more intensive chemotherapy as well as RT to the CNS have reported ALL 5-year survivals of 50–70% (Kumar et al. 1995; Kun et al. 1984; Ribeiro et al. 1995; Ritchey et al. 1999). There is an ongoing debate between cranial RT alone and CSI. Doses used have generally been approximately 18–24 Gy to the brain and 10–15 Gy to the spine. Most nonrandomized comparisons suggest superior outcomes with CSI. One small phase III trial did show superiority for CSI compared to cranial RT (Land et al. 1985).
Several prognostic factors have been found to be of importance in the setting of CNS relapse (Bleyer et al. 1986). Patients who were originally deemed at diagnosis to be at low risk for CNS relapse by virtue of a low initial leukocyte count (<20,000/μL), who originally did not receive cranial irradiation, or whose CNS relapse occurred at a relatively long period after the original diagnosis have a better prognosis after CNS recurrence. An isolated CNS relapse has generally been more favorable compared to combined CNS and systemic relapse. After completing chemotherapy, those children with CNS relapse have better outcomes with longer disease-free intervals. Within the Pediatric Oncology Group (POG), RT for isolated CNS relapse of ALL used a cranial dose of 24 Gy and a spine dose of 15 Gy. The 4-year event-free survival was 71%. Those patients who presented with greater than an 18-month disease-free interval prior to CNS relapse had a 4-year event-free survival of 83% compared to 46% for those with shorter initial remission durations (Ritchey et al. 1999). This led subsequent POG trials to omit RT to the spine if there is a long disease-free interval. Whether the omission of spinal RT in this favorable subgroup is detrimental is unclear. However, high-dose Ara-C has generally been added to the management of CNS relapse of ALL. One report using high-dose Ara-C reported a favorable 63% complete response rate (Morra et al. 1993). These concepts have been maintained within current COG trials for CNS relapse of ALL; but with intensification of systemic therapy, spinal RT has been proposed to be dropped even for short disease-free intervals, while the brain is treated to 18 Gy.
17.6.4 Role of RT: AML
The role of CNS prophylaxis is not well defined for AML, particularly because CNS relapse rates are relatively infrequent (at roughly 5–10%) for this uncommon pediatric leukemia. The German-based BFM group has studied the role of prophylactic RT in combination of increased intense induction therapy with anthracycline an intrathecal Ara-C. The AML-BFM-87 study found that PCI decreased risk of CNS and bone marrow relapse. Five-year disease-free survival for patients who received cranial RT was 69% compared to 46% those not receiving RT Response to 12 Gy appeared to be similar to 18 Gy (Creutzig et al. 1993).
Other studies, however, show no clear difference in relapse rates with cranial radiation. Nevertheless, many pediatric regimens employ IT drugs such as Ara-C. Patients with a high WBC count at diagnosis or monocytic variants of AML are believed to have a higher risk for CNS relapse, which may justify both IT chemotherapy and cranial radiation in this setting. Moreover, additional follow-up of the AML-BFM-87 along with additional patient numbers showed a reduced risk for both marrow and CNS relapses when cranial RT was followed intensive induction therapy emphasizing anthracycline chemotherapy and intrathecal Ara-C chemotherapy. In a nonrandomized analysis, current data suggest that 12 Gy results in the same relapse rate as 18 Gy (Creutzig et al. 2013).
Chloromas (or myeloid/granulocytic sarcomas) are solid masses of leukemic cells that can appear in a variety of extramedullary sites, including epidural space, meninges, and extracranial site such as skin, soft tissue, and GI tract. Doses in the range of 10–20 Gy are effective although planning should take into account the possibility of future TBI.
17.7 Target Delineation
17.7.1 RT Volumes
Whole brain radiotherapy requires full coverage of the intrathecal subarachnoid space, and particular care should be taken to include the cribriform plates. The posterior globes are generally covered due to risk of relapse in the subarachnoid extension along the optic nerves. Inferior margins are typically the bottom of the first or second cervical vertebra and should include the entire vertebral body, ensuring coverage of the obex of the fourth ventricle. This may also be particularly important to help matching with any future spinal treatments. Anterior eye blocks should not be so generous to prevent irradiation of the cribriform plate and the middle cranial fossa. In all other field borders, blocks may flash over the scalp. A theoretical reason why the spine RT need not be treated in conjunction with intrathecal chemotherapy is the poor circulation of intrathecal drugs within the ventricles and sulci of the brain relative to the subarachnoid space in the spine (Rieselbach et al. 1962; Shapiro et al. 1975). See Fig. 17.2 demonstrating cranial RT fields used for leukemia.
