Keywords
Gene therapy, drug resistance, glioblastoma, chemoprotection, in vivo selection, MGMT, hematopoietic stem cell, temozolomide
Introduction
Gene therapy was initially developed as an ideal treatment for defective single-gene diseases because it addresses the underline etiology. Through gene transfer, we can introduce a functional or therapeutic gene to different types of cells, from defective cells to normal stem cells to cancer cells. Since the beginning of gene therapy, its utilization to treat cancer has been proposed because of the ongoing need to search for effective treatments for cancer. However, early gene therapy studies and trials showed the technical difficulty of achieving a therapeutic goal. Two of the most common challenges for gene therapy are the low transduction efficiency and inadequate number of gene-modified cells in the clinical setting.
It remains extremely difficult to eradicate all cancer cells. Conventional cancer treatments were often depicted as bringing the patients to the brink of death and hoping for them to beat the odds and recover . Even such harsh intervention has not been able to kill all cancer cells or keep them at bay for long. Often in the end, metastasis and treatment resistance are the major culprits for patient death. This is often due to the dose-limiting toxicity, most often to the hematopoietic system. Because the mechanisms of many cancer cell-killing drugs are often designed to target cellular activities also used by normal cells, most chemotherapeutic drugs have certain degrees of cytotoxicity. Cancer drug resistance is increased by intermediate dose that allows resistance to develop. Even cancer agents that target cancer cell-specific mutations and that have less toxicity toward normal cells result in cancer cell resistance, likely due to the continued clonal evolution of cancer due to unstable genomes . In addition, some cancer stem cells or tumor-initiating cells have intrinsic treatment resistance , develop mechanisms to further mitigate or evade toxic effects, and contribute to tumor recurrence.
Gene transfer of drug-resistance gene can overcome the problem of low transduction efficiency and low transduced cell numbers. It provides a basis for in vivo selection. Drug-resistance genes can provide transduced cells a survival advantage over nontransduced cells upon drug treatment. When transduced cells are stem cells or progenitor cells, they will survive the treatment and proliferate, increasing the overall percentage of cells expressing therapeutic genes. The survival advantage introduced by drug-resistance genes to normal cells allows the prospect of increasing drug dosage without further myelosuppression. In this chapter, we focus on the gene therapy of drug-resistance genes and review some of the most popular mechanisms and their preclinical and clinical studies for human gliomas.
Mechanisms of Drug-Resistance Gene Therapy
Many types of cancer have been shown to be extremely difficult to eradicate because they have intrinsic resistance to treatments, and they can also develop new resistance in responding to cancer treatments. Unfortunately, once they develop certain treatment resistance, they often develop cross-resistance to other treatment strategies as well. Several treatment-resistant mechanisms used in drug-resistance gene therapy have been observed in cancer cells. Likewise, Bertino et al . suggested to use mechanisms found in resistant cancer cells to develop strategies for drug-resistance gene therapy . In the past two decades, several popular mechanisms have been studied and explored for drug-resistance gene therapy.
Multidrug-Resistant Gene (MDR)
Multidrug-resistant protein 1 (MDR-1) was encoded by the human ABCB1 gene, also called the MDR1 gene. The protein product is P-glycoprotein and belongs to ATP-binding cassette transporters, subfamily B member 1. It is a 170-kDa transmembrane protein and acts as an ATP-dependent efflux pump to remove cytotoxic drugs from cytoplasm ( Figure 26.1 ). It is multidrug resistance because it has broad substrate specificity, and it can decrease the cytosolic accumulation of vinca alkaloids, taxanes, epipodophyllotoxins, and anthracyclines . In various types of cancer, such as breast cancer, lung cancer, gastric carcinoma, and chronic lymphocytic leukemia, overexpression of MDR1 has been linked to multidrug resistance in vitro and treatment resistance in clinics . Because of its effectiveness in eliminating various types of chemotherapeutic drugs from cell cytoplasm, the ABCB1 gene is one of the earliest genes considered for drug-resistance gene therapy. The strategy is to transfer the ABCB1 gene into normal hematopoietic stem or progenitor cells, allowing those gene-modified cells and their progenies to overexpress MDR1 protein and become more resistant to chemo drugs than cancer cells during treatment ( Figure 26.1 ).
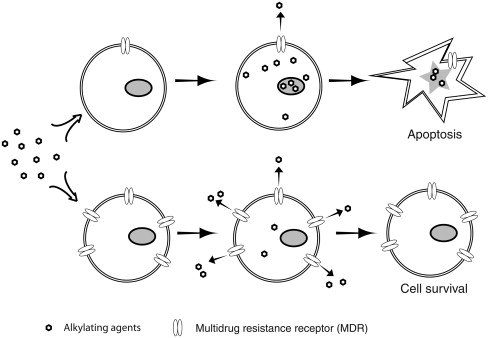
Soon after the link between multidrug resistance and MDR1 was discovered, Guild et al . used retroviral vector to introduce murine mdr cDNA into NIH 3T3 cells to show that transfected cells were highly resistant to colchicine, vinblastine, or doxorubicin even with one copy of the vector after colchicine selection or two copies of the vector without prior selection . Similar studies with human MDR1 cDNA were performed in bone marrow cells of the DBA/2J mice . A high percentage of transduced bone marrow progenitor cells showed resistance to both colchicine and vinblastine treatments by an in vitro colony-forming assay. The first i n vivo selection of drug-resistant bone marrow cells after retroviral gene transfer of human MDR1 was done in 1992 . Human MDR1 cDNA was subsequently transferred into human CD34 + cells from cord blood or bone marrows and showed enrichment after chemoselection and improvement of chemotherapy tolerance , paving the way for human MDR1 clinical trials. Many drug-resistant cancer cells also have overexpressed P-glycoprotein, which can be blocked by trans -(E)-flupentixol, an inhibitor of P-glycoprotein, and become sensitive to cancer drugs again. A mutant form of P-glycoprotein (MDR1-F983A) was developed to protect hematopoietic cells from chemotherapeutic drugs in the presence of trans -(E)-flupentixol . In recent studies, lentiviral vectors have been used to efficiently transfer MDR1 to human CD34 + hematopoietic progenitor cells and protect them against radiotherapy and chemotherapy . MDR1 has also been introduced to keratinocyte stem cells by lentiviral vector for the selection of transduced cells and chemoprotection against topical application of colchicine in the treatment of skin disease .
O 6 -Methylguanine Methyltransferase (MGMT)
One of the most popular and successful drug-resistance genes is O 6 -methylguanine methyltransferase, also known as alkylguanine DNA-alkyltransferase (AGT). It is a 21-kDa DNA repair protein, encoded by human MGMT gene, that is mainly responsible for repairing DNA damage caused by alkylating agents in a suicide reaction . Alkylating agents, such as temozolomide (TMZ) and 1,3-bis(2-chloroethyl)-1-nitrosourea (BCNU), are often used as chemotherapeutic agents in cancer treatment because they add an alkyl group to the O 6 position of guanine, which is a highly genotoxic lesion, resulting in mutation, double strain break, and interstrand cross-link . Endogenous MGMT protein can irreversibly transfer the alkyl group from alkylated guanine to a cysteine residue on itself and undergo the degradation process. Various types of cancer cells have increased the level of MGMT protein to make themselves more resistant to the treatment of alkylating agents . The methylation status of MGMT promoter has been studied in patients as a potential predictive marker for prognosis and response to alkylating treatment in various types of cancer, particularly in high-grade glioblastoma . There is also evidence linking TMZ resistance to cancer stem cells in gliomas . Therefore, treatment that targets endogenous MGMT has been suggested as a valid approach to enhance chemo treatment in treatment-resistant patients . To resensitize resistant cancer cells, an MGMT inhibitor, O 6 -benzylguanine (BG), is often used to permanently inactivate wild-type MGMT protein in cancer cells to impair their cellular repair mechanism against alkylating agents . However, in a recent clinical trial, it was found that different TMZ-resistant high-grade gliomas may respond differently to O 6 -BG treatment. Anaplastic glioma was resensitized to TMZ after treatment with O 6 -BG, whereas glioblastoma multiforme was not, indicating different or additional TMZ-resistant mechanisms .
One of the most severe side effects of cancer chemotherapy is myelosuppression. In the early days of MGMT gene therapy, because of its myeloprotection, whole length MGMT cDNA was introduced to hematopoietic cells of mouse and human . However, in the application of drug-resistance gene therapy for cancer, to provide a unique drug-resistance mechanism in normal cells that is different from that in cancer cells and to reduce resistance in cancer cells, mutant forms of MGMT were screened and developed , and the two most effective mutations are P140K and G156A (proline-to-lysine mutation at amino acid 140 and glycine-to-alanine mutation at amino acid 156). Both mutations are shown to be highly resistant to BG-mediated degradation but are still functional in repairing DNA damage caused by alkylating agents . After introducing mutant forms of MGMT to normal stem and progenitor cells by gene transfer, a combination treatment of BG and alkylating agents will inactivate endogenous MGMT protein inside cancer cells and make them more sensitive to alkylating agents. At the same time, functional mutant MGMT can repair damaged DNA by removing DNA adducts and protect gene-modified cells against alkylating agents, allowing an in vivo selection of transduced cells ( Figure 26.2 )—an approach first characterized by Gerson and coworkers .
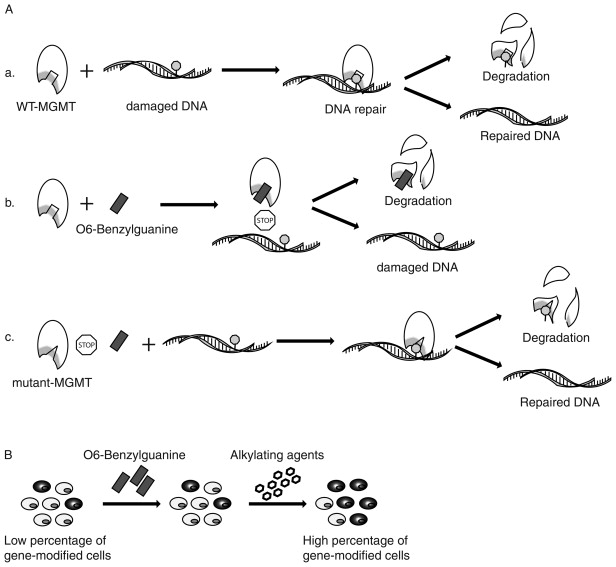
Preclinical studies with mutant MGMT have been performed extensively in the past two decades. Stable gene transfer into both murine and human hematopoietic cells was achieved by using retroviruses and resulted in resistance against O 6 -BG and BCNU treatment . In vivo enrichment of transduced cells under drug selection and chemoprotection against the alkylating agents BCNU and TMZ was first investigated in murine models . It has been shown to protect mice against lethal treatment of BCNU, and it provided significant enrichment of transduced cells after O 6 -BG and BCNU or TMZ treatments in non-myeloablated recipients. In one study, as high as 93% transgene-positive cells were achieved in mice from the initial 12% at the time of transduction and transplantation through in vivo drug selection in primary followed by secondary recipients, indicating reconstitution of hematopoiesis from gene-modified hematopoietic stem cells .
In vivo selection of gene-modified cells has also been studied to enhance treatment of murine β-thalassemia, murine protoporphyria, low-frequency lung engraftment from transduced bone marrow cells, and murine model of hemophilia . In recent small animal studies, a novel imaging technology provided us the tool to monitor bone marrow transplantation and in vivo drug selection with mutant MGMT . From these imaging experiments, there is clear evidence of dynamic engraftment of transduced hematopoietic stem cells after in vivo BG+BCNU selection ( Figure 26.3 ). Not only was focal long-term gene expression identified, and increased after drug treatment, but also individual areas of hematopoietic stem cell engraftment and expansion were observed for up to 9 months at individual sites, suggesting a stochastic rather than systemic process of hematopoiesis under these conditions.
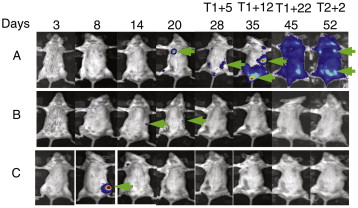
However, there was a huge disparity between successful gene therapy study in mice and its application in humans. It has been difficult to achieve sufficient gene transferring in human trials to observe its clinical benefit. Large animal and nonhuman primate studies were needed to pave the way for successful clinical trials. Successful MGMT gene transfer and myeloprotection have been reported in a canine model and nonhuman primates . In several of these studies, stable and multilineage selections in hematopoietic system have been achieved through several-round BG/TMZ or BG/BCNU treatments with a longest observation time of 2.2 years.
To expand drug-resistance capability against various types of treatments, combinational drug resistance was achieved by gene transferring two different mechanisms of drug resistance into the same targeted cell. MDR1 and mutant MGMT have been integrated into one bicistronic vector to provide modified cells with protection against BG/ACNU or BG/TMZ and vincristine or paclitaxel treatments . Cells transduced with dual drug-resistance genes showed more resistance to combination treatments and better selection outcome.
Cytidine Deaminase (CDA or CDD)
Cytidine deaminase is a key enzyme involved in the pyrimidine salvage pathway and maintains the cellular pyrimidine supply. It is encoded by the human CDA gene. In the 1960s, cytosine nucleoside analogs, such as cytarabine, were reported to contain antitumor effects. Cytidine analogs, such as azacitidine, can also induce cell differentiation and change methylation status of newly synthesized DNA . Nucleoside analogs are mainly used to treat acute myeloid leukemia and non-Hodgkin’s lymphoma . However, an elevated level of cytidine deaminase has been identified as one of the contributing factors in cellular resistance to nucleoside analogs ; thus, CDA was selected as a candidate for drug-resistance gene therapy.
In early studies, human CDA was introduced into murine fibroblast and hematopoietic cells by retroviral gene transfer, and the results conferred enhanced resistance to nucleoside analogs . Transplantation of gene-modified bone marrow cells in lethally irradiated recipients showed long-term expression of CDA; however, ex vivo selection with nucleoside analogs has been challenging due to the release of CDA in the culture media .
In recent studies, in vitro selection of human hematopoietic progenitor cells and protected myelopoiesis by gene transferring of CDA in murine transplantation model have been reported . However, lymphotoxicity with the overexpression of CDA was also reported. To minimize the side effect of overexpressed CDA, Lachmann et al . generated an inducible transgene expression system to regulate CDA expression and showed robust myeloprotection with minimal lymphotoxicity .
Dihydrofolate Reductase (DHFR)
Dihydrofolate reductase is an enzyme that converts dihydrofolate to tetrahydrofolate and is involved in purines and thymidylate synthesis. It is encoded by the human DHFR gene. Antifolate drugs, methotrexate (MTX) and trimetrexate, can tightly bind to DHFR and inhibit DNA synthesis and cell proliferation. On that account, antifolate drugs have been used as potent antitumor drugs. A recent study showed that MTX can also induce oxidative DNA damage, and this kind of treatment is particularly detrimental to MSH2 defective cancers, such as hereditary non-polyposis colon cancer . However, cancer cells can develop resistance to MTX through several mechanisms, such as DHFR overexpression, altered drug transporter, and DHFR promoter polymorphism . Mutant forms of murine and human DHFR has been discovered in methotrexate-resistant cancer cells .
One of the major side effects of methotrexate treatment is also myelosuppression, which makes mutant DHFR another candidate for drug-resistance gene therapy. During the early phase of gene therapy, DHFR was used as a model system for myeloprotection and in vivo selection. It has been successfully introduced into murine, canine, and human hematopoietic cells and showed protection against MTX cytotoxicity . One of the more potent mutations in DHFR that confers most resistance is a Leu-to-Tyr mutation at the codon number 22, particularly when used with trimetrexate treatment . When transplanting DHFR modified murine bone marrow cells to recipients with only mild preconditioning, even low-level engraftment (1%) of transduced murine bone marrow cells can confer methotrexate resistance after MTX treatment . In addition to mutant DHFR gene transfer, efficient DHFR-mediated selection may also require the treatment of MTX or TMTX with a nucleoside transport inhibitor, nitrobenzylmercaptopurine ribose phosphate . In recent studies, mutant DHFR gene transfer effectively protected hematopoietic stem cells and facilitated the combined treatment of trimetrexate and anti-CD137 immunotherapy for large solid tumors, and similar in vivo selection was also observed in human embryonic stem cell-derived cells when transplanted into NSG mice and treated with methotrexate .
Glutathione S -Transferase (GSTP1 and MGST2)
The glutathione S -transferase superfamily proteins can detoxify many endogenous compounds and break down xenobiotic substrates through conjugation of reduced glutathione with various substrates. GST proteins exist throughout the cells, from cytosol to mitochondria to microsomes. GST-pi (GSTP1) has been shown to be resistant to cisplatin, BCNU, cyclophosphamide, busulfan, and melphalan in cancer cell lines . Subsequently, GST-pi overexpression has been linked to treatment resistance in lung cancer, acute myeloid leukemia, head and neck cancer, and breast cancers .
In drug-resistance gene therapy studies, GST-pi transduced human CD34 + hematopoietic cells have been shown to be resistant to alkylating agents, doxorubicin, cisplatin, melphalan, and cyclophosphamide . Recently, overexpression of MGST2 and treatment of busulfan have been shown to increase cell survival in the fibroblast cell line. Because MGST2 was not highly expressed in murine bone marrow CD34 + cells, it can be used as a potential drug-resistance gene in hematopoietic stem cell gene therapy .
Hypoxanthine Phosphoribosyltransferase (HPRT)
Hypoxanthine phosphoribosyltransferase is a human enzyme involved in the purine salvage pathway. It recycles guanine to guanosine monophosphate during DNA degradation. It is encoded by the human HPRT1 gene and has been widely studied since the 1960s. The deficiency of HPRT in humans has been linked to Lesch–Nyhan syndrome and gout due to a high level of uric acid in the blood . Ultraviolet and radiotherapy have been shown to increase the HPRT mutation frequency in cancer cells , which used the mutation to develop resistance to 6-thioguanine, an antimetabolite for treating cancer . Interestingly, a recent study has also shown that 6-thioguanine resistance in melanoma cells is linked to increased levels of MGMT .
As a nucleotide analog, 6-thioguanine is relatively less toxic. Thus, knockdown HPRT gene expression in combination with 6-thioguanine can be a potential in vivo selection strategy. Porter and DeGregori demonstrated the use of a lentiviral vector, containing interfering RNA against hypoxanthine phosphoribosyltransferase, to transduce murine bone marrow cells and transplant them into recipients. After 6-thioguanine treatments in recipients, they observed increased percentages of transduced peripheral blood cells and hematopoietic progenitor cells in the bone marrow compared with control or untransduced cells. Thus, they suggested that interfering RNA-mediated purine analog resistance (iPAR) plus 6-thioguanine can be used for in vivo selection . Hacke et al . showed that 6-thioguanine alone could be used as a non-myeloablative preconditioning and in vivo selection agent in HPRT-deficient murine bone marrow cell transplantation . By using 6-thioguanine alone, they were able to achieve consistently 95% of engraftment from HPRT-deficient donor bone marrow cells, resulting in normal hematopoiesis in both primary and secondary recipients.
Gene Delivery Methods
Corrective genes or therapeutic genes can be delivered to patient cells by in vitro or in vivo methods, and delivery vehicles include naked DNA, artificial chromosome, adenovirus, adeno-associated virus, retrovirus, and lentivirus. Recently, transposon and transposase and zinc finger nucleases have been added as additional delivery methods. In current clinical trials, γ-retroviral transduction is still the dominant method of gene transfer despite the possible adverse effect of insertional mutagenesis. The use of lentivirus for gene transfer in preclinical studies and clinical trials is on the rise because lentiviruses transduce nondividing cells, such as quiescent stem cells, and are less likely to cause insertional gene-activating mutagenesis compared to γ-retroviruses. Zinc finger nucleases are artificial DNA-binding proteins that generate double-strain DNA break at specific sequences, allowing site-directed genome editing and gene correction. Zinc finger nucleases have great promise in the field of gene therapy because of its minimum toxicity and high specificity . They are transiently expressed during gene transfer and allow the safe integration of drug-resistant genes into targeted cells. More detailed descriptions of gene delivery methods are discussed in Section I .
Preclinical Studies for Human Gliomas
Human gliomas are classified as grades II–IV by the World Health Organization based on histopathological degrees. Grade II includes astrocytoma, oligoastrocytoma, and oligodendroglioma; grade III includes anaplastic astrocytoma, anaplastic oligoastrocytoma, and anaplastic oligodendroglioma. Grade IV is glioblastoma, which is the most malignant . Glioblastoma is the most common brain tumor in adults, accounting for half of all brain tumors, and brain tumor is also the most common solid tumor in children. Effective treatment for glioblastoma remains challenging. Median survival is approximately 1 year after diagnosis for glioblastoma patients, and 2-year survival rate remains poor. Upon first diagnosis and if condition allows, initial treatment is usually surgical removal followed by radiotherapy and chemotherapy. In the 2005 European Organization for Research and Treatment of Cancer (EORTC) 26981-22981–National Cancer Institute of Canada (NCIC) CE.3 trial involved 85 oncology centers, radiotherapy combined with chemotherapy was compared with radiotherapy alone in 573 patients with glioblastoma. Radiotherapy with TMZ extended medial survival from 12.1 months to 14.6 months and increased 2-year survival rate two- or threefold . TMZ is an oral alkylating agent that can easily cross the blood–brain barrier. In the early 1990s, TMZ was shown to be effective in treating primary brain tumors, with high tolerance and minimal toxicity . It was not until 2005 that the U.S. Food and Drug Administration approved it for treatment in newly diagnosed glioblastoma, in conjunction with radiation therapy . As one of the drug-resistance mechanisms, MGMT has been shown to be able to repair DNA damage caused by TMZ, thus resulting in TMZ resistance . Subsequently, Estelle et al . demonstrated that MGMT promoter methylation in some glioma patients silenced MGMT activity, rendering their tumors more responsive to alkylating agents and increasing overall survival in patients . By analyzing the methylation status in the same 2005 multicenter trial, Hegi et al . showed that MGMT promoter methylation was useful in predicting the response of glioblastoma patients to treatment with TMZ and radiotherapy, and similar to Estelle et al .’s study, approximately 45% patients had MGMT promoter methylation . These landmark studies have prompted the call for MGMT methylation status testing in all newly diagnosed glioma patients. It has also been suggested to test MGMT protein level or measure mRNA, but there are limitations to both methods .
Despite the fact that TMZ is well tolerated, it still causes myelosuppression. In certain cases, it causes severe thrombocytopenia and pancytopenia when used in combination with radiotherapy . Therefore, gene transfer of mutant MGMT (MGMT-P140K) to hematopoietic stem cells would provide multiple benefits in treating TMZ-resistant glioma. First, by adding O 6 -BG, it can inactivate endogenous MGMT in tumor cells, making them more sensitive to TMZ treatment. Second, infused autologous hematopoietic stem cells can rescue myelosuppression from initial radiotherapy and chemotherapy. Third, gene-modified hematopoietic stem cells can generate protected bone marrow cells and maintain hematopoiesis against further TMZ treatment and thus increase the tolerance dosage of TMZ. Fourth, further TMZ treatment can in vivo select gene-modified bone marrow cells, providing myeloprotection.
An initial preclinical study has been performed using the human glioma xenograft model in NOD/SCID mice. Kreklau et al . transduced murine hematopoietic stem cells with MGMT(P140K) retroviral vectors and challenged them with a high-dose combination of BG and BCNU treatment . After in vivo selection with BG and BCNU, murine bone marrow cells were harvested and transplanted into secondary recipients, and then secondary recipients were injected with human glioma SF767 xenograft tumors. After in vivo selection, there were 80% transgene-positive bone marrow cells compared to 6.5% before selection. Three weeks after injecting tumor cells, mice were treated with one cycle of high-dose BG/BCNU, and tumor sizes were measured for 28 days. In mice that received MGMT(P140K)-positive bone marrow cells, tumor sizes increased 1.7-fold, whereas in mice that received control bone marrow cells, tumor sizes increased 6.8-fold. However, after two cycles of BG/BCNU treatment, in mice that received MGMT(P140K)-positive bone marrow cells, tumor sizes had shrunk 0.2-fold, whereas tumor sizes in control mice increased 7.6-fold. In secondary transplanted animals, there were on average 90% transgene-positive bone marrow cells. From the previous experiments, there was clear evidence that MGMT(P140K) protected gene-modified bone marrow cells, allowed effective in vivo selection, and allowed dose-intensified treatment for glioma cells. Effective in vivo selection and myeloprotection have also been observed in large animals, as mentioned previously. Gori et al . tested a clinically relevant proconditioning regimen in dogs by giving them reduced-intensity BCNU and TMZ treatment before infusing LV-MGMT-P140K transduced canine bone marrow cells . The study showed sustained in vivo selection and stable myeloprotection from MGMT(P140K)-modified canine bone marrow cells. Remarkably, dose escalation of TMZ of more than 10-fold was well tolerated once selection in favor of the transduced hematopoietic cells had occurred. This indicates that the dose-limiting toxicity of TMZ is truly hematopoietic, and other organs tolerate higher levels of DNA damage.
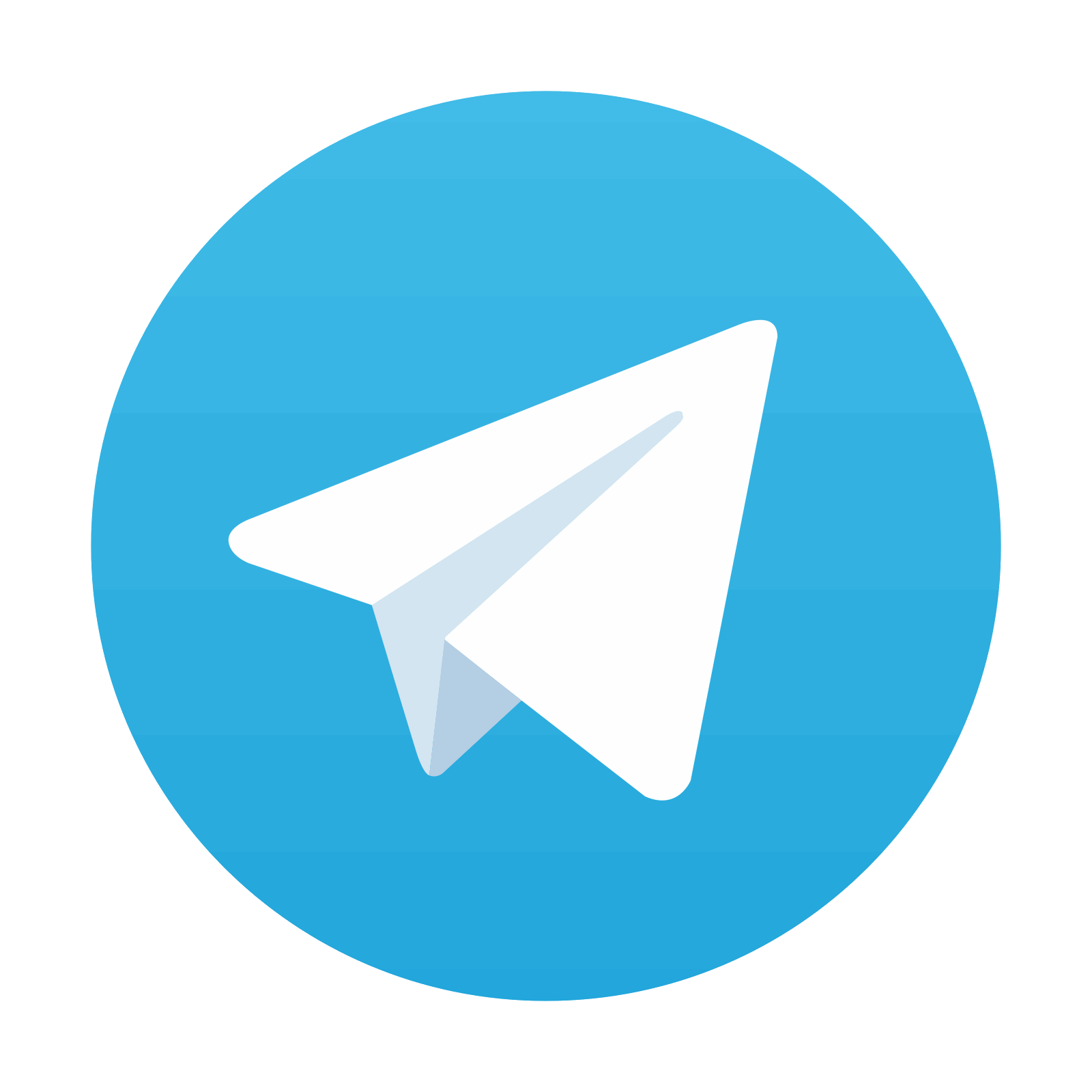
Stay updated, free articles. Join our Telegram channel

Full access? Get Clinical Tree
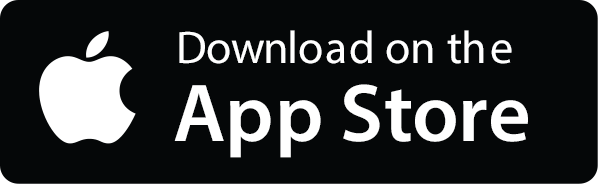
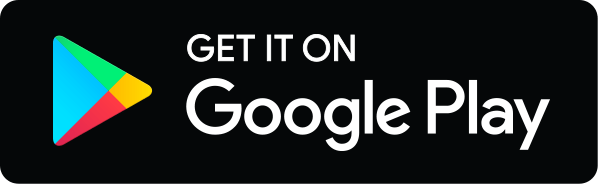