© The Author(s) 2017
Anne T. Mancino and Lawrence T. Kim (eds.)Management of Differentiated Thyroid Cancer10.1007/978-3-319-54493-9_66. Clinical Presentation and Diagnosis of Papillary Thyroid Cancer
(1)
Department of Surgery, Section of Endocrine Surgery, Northwestern University Feinberg School of Medicine, 676 North Saint Clair Street, Suite 650, Chicago, IL 60611, USA
Keywords
Papillary thyroid cancerDiagnosisTreatmentRisk factorsIntroduction
Over the past half century, the primary treatment for biopsy-proven papillary thyroid cancer (PTC) has been surgical, and in the United States (US), it has been almost always treated with a total thyroidectomy [1]. Recent reports on the outcomes of hemithyroidectomy for PTC [2, 3] and observation of small, low-risk cases of PTC [4] have renewed the controversy over extent of surgery and have even suggested that some patients with quiescent disease can be placed into active surveillance protocols. In addition, there have been several recent high-profile publications indicating that there is an epidemic of unnecessary overdiagnosis of thyroid cancer [5, 6]. Due to these factors, the clinical decision-making process for patients with PTC is more complex than ever. There must be a rational approach to the workup of thyroid nodules and the management of thyroid cancers in order to avoid placing undue burden on patients and the healthcare system. Herein, we discuss the clinical presentation of PTC and outline the steps for the evaluation of the patient with thyroid nodules or newly diagnosed PTC.
The Incidence of Thyroid Cancer Is Rising
Thyroid cancer is the fifth most common cancer in women in the United States, and over 62,000 new cases occurred in both men and women in 2015 [7]. Although the most common presenting symptom of thyroid cancer is a neck mass, most thyroid nodules and in turn, thyroid cancers, are diagnosed when asymptomatic nodules are evaluated. Thyroid cancer is steadily, and some would say explosively, increasing in incidence in the United States. The incidence of thyroid cancer in 1973 was 3.6 cases per 100,000 people. The incidence of thyroid cancer as of 2009 has increased almost fourfold to 14.3 cases per 100,000. This dramatic increase is virtually completely attributable to an increase in the incidence of PTC, with the incidence increasing from 2.7 cases per 100,000 people in 1973 to 12.5 per 100,000 in 2009 [8, 9].
One explanation for this phenomenon is that there has been some change in the risk of thyroid cancer in the general population in the United States. The most well-established risk factor for the development of thyroid cancer is a history of exposure to ionizing radiation, particularly during childhood. There is evidence to support that the general population has had increasing exposure to ionizing radiation over time, particularly from medical sources; however, there is insufficient evidence to attribute any significant proportion of the increase in thyroid cancer incidence to increasing radiation exposure [10]. In contrast to other malignancies, cigarette smoking has been shown to have a protective effect on the risk of thyroid cancer [11], and smoking has decreased in the United States along with the increased incidence of thyroid cancer. However it is difficult, and dangerous, to attribute a large part of the rise in incidence in thyroid cancer to a decrease in smoking rates. Other risk factors for thyroid cancer that have been described include obesity [12], taller height [13], and changes in dietary iodine fortification [14].
An alternate explanation for the apparent increase in the incidence of thyroid cancer is the substantial evidence that increased detection, or overdiagnosis, has played an important role over time [8, 9, 14]. A study recently published by the International Agency for Research on Cancer suggests that the rising incidence of thyroid cancer in multiple countries across the globe can be attributed to overdiagnosis [5]. In industrialized countries, the introduction of widely available ultrasound machines and population screening appear to be the key factors associated with overdiagnosis. Increases in the incidence of thyroid cancer have also been attributed to the incidental detection of asymptomatic thyroid nodules on computed tomography (CT), magnetic resonance imaging (MRI), and positron-emission tomography (PET) scans. As a result, more small nodules harboring early stage thyroid cancers are being detected and treated.
It is well known that there are a substantial proportion of patients that have subclinical thyroid cancer. This subpopulation of patients, if never diagnosed with thyroid cancer, will die of other causes, and the untreated thyroid cancer will not contribute in a negative way to their health or longevity. The idea of a “substantial reservoir” [9] of patients with subclinical thyroid cancer is supported by multiple autopsy studies, most notably that of Harach et al. [15], which demonstrated that 36% of people not known to have thyroid cancer during their lifetime had one or more foci of thyroid cancer at the time of autopsy. The mean diameter of incidentally discovered thyroid cancers in one multicenter study was 1.1 cm [16]. Well-differentiated thyroid cancers less than 1 cm in diameter (“microcarcinomas”) have an exceedingly low rate of distant metastatic disease and mortality rates near zero [17]. Those who believe that overdiagnosis is the driver of increasing thyroid cancer incidence point to the evidence that the overall mortality rate for thyroid cancer has remained relatively stable over the same time period (0.5 deaths per 100,000 people) [5, 9]. Critics of the overdiagnosis theory cite the evidence that thyroid cancer mortality has increased in the United States in recent years, with an annual percent change of 0.8% per year from 1992 to 2012 [14]. In the end, it is likely that both a change in the risk profile of the US population and overdiagnosis have contributed to the increase in incidence of thyroid cancer.
What Are the Risk Factors for Developing PTC?
Demographics and Epidemiology of Thyroid Cancer
The probability of malignancy in a thyroid nodule is affected by the patient’s age, sex, race, and ethnicity [18, 19]. New nodules identified in patients under 30 years of age or older than 60 have a higher risk of malignancy compared with those arising in the fourth or fifth decade [20]. Approximately 75% of all patients with PTC are women, but a new thyroid nodule found in a male has a higher likelihood of being malignant [19]. Non-Hispanic whites have the highest risk of thyroid cancer by ethnicity, followed by Asian/Pacific Islanders, Hispanics, and non-Hispanic blacks [14].
Thyroid cancers of follicular cell origin comprise the vast majority of thyroid cancers; 85% of cases are PTC, 12% of cases are follicular or Hurthle cell carcinomas, and <3% of cases are poorly differentiated carcinomas [21]. Variants of PTC exist, including the follicular variant of PTC (FVPTC), and more aggressive variants such as the tall cell variant, columnar variant, insular variant, solid/trabecular variant, and diffuse sclerosing variant. FVPTC and follicular and Hurthle cell carcinomas have been demonstrated to have similar prognoses as classic PTC [22, 23]. Recently, the encapsulated noninvasive variant of FVPTC has been reclassified as noninvasive follicular thyroid neoplasm with papillary-like nuclear features (NIFTP) rather than a carcinoma due to its indolent course [24].
Family History
A family history of thyroid cancer or a syndrome associated with the development of thyroid cancer should be specifically sought. Approximately 3–9% of cases of follicular cell-derived thyroid cancers are familial and are inherited in an autosomal dominant pattern. The majority of these are PTCs that are inherited in a non-syndromic fashion. These non-syndromic familial thyroid cancers are referred to as familial non-medullary thyroid cancer (FNMTC). Recent studies from the NIH have identified a putative susceptibility gene (HABP2) [25]; however, at this time there are no clinical genetic tests available. The definition of FNMTC is satisfied when two or more first-degree relatives are found to be affected with the same follicular cell-derived thyroid cancer [26]. There is controversy regarding this definition because the specificity is low. In families with only two relatives with thyroid cancer, there is still a 60% chance that the cancer is sporadic [27]. A stricter definition of FNMTC requiring three or more affected family members yields a higher probability of genetic linkage. For larger kindreds it should be possible to adequately evaluate this risk factor by taking a history, whereas those smaller kindreds with less than two siblings may be impossible to detect. Follicular cell-derived thyroid cancers are also a recognized component of several familial cancer syndromes including familial adenomatous polyposis, Gardner syndrome, Cowden syndrome (PTEN hamartoma tumor syndrome), Carney complex type 1, Werner syndrome (adult progeria), and the DICER1 syndrome.
Radiation History
Radiation exposure in childhood, either from external sources or when ingested, is a risk factor for the development of thyroid cancer. The experience of using radiation for benign conditions in infants and children led to the discovery that a significant exposure to ionizing radiation in childhood, particularly to the head and neck area, is a risk factor for the development of thyroid pathology [28]. In the first half of the twentieth century, many benign childhood conditions were treated with external beam radiation including acne, tinea capitis, ringworm, tonsillitis, and enlarged thymus. Specifically, it was found that there was a higher risk of both benign and malignant thyroid disease in those who received radiation treatments in childhood [29]. The lag phase for the development of thyroid cancer appears to be approximately three decades following the exposure of low-dose external radiation to the head and neck [30]. In one study of over 12,000 survivors of childhood cancer, the risk of developing thyroid cancer was related to the dose of radiation received up to 20 Gy and peaked at 14.6-fold [31]. The risk of cancer appears to be dose dependent, have a linear dose-response curve, and also is dependent upon age of exposure [32]. The greatest risk appears to be for those who were aged 5 or younger at the time of radiation exposure [32–34]. It was later recognized that external beam radiation in childhood is also a risk factor for the development of hyperparathyroidism, salivary tumors, acoustic neuromas, and other neural tumors [35, 36]. Mantle field radiation used for the treatment of Hodgkin’s lymphoma and total body irradiation for bone marrow transplantation are also risk factors for the development of thyroid cancer [31, 37]. Several studies have also indicated that patients who received radioactive iodine ablation for hyperthyroidism also have an increased risk of thyroid cancer [38–40]. Following the meltdown at the Chernobyl nuclear reactor in Ukraine in April of 1986, radioactive fallout of iodine (I-131) led to contamination of the local environment. The incidence of thyroid cancer rose sharply in the following decades, primarily for those individuals who were children living in Belarus, western Russia, and Ukraine at the time of the meltdown [41–44]. The risk of developing thyroid cancer was increased for individuals exposed to nuclear fallout from the Chernobyl meltdown, atmospheric nuclear tests in Nevada in the 1950s, the Marshall Islands nuclear bomb testing, and Hiroshima and Nagasaki atomic bomb survivors [41, 44–47].
Nodular Goiter
The presence of a nodular thyroid has been associated with increased risk of thyroid cancer [16]. The overall rate of incidental thyroid cancer in surgical specimens of patients treated for benign thyroid conditions is 15.6%. Most notably, 17.5% of patients with multinodular goiter harbor incidental thyroid cancer, mostly papillary thyroid carcinoma. Toxic nodular goiter, historically described as having low rates of malignancy, has been more recently described to harbor thyroid cancer in 18.3% of surgical specimens in which malignancy was not suspected [48]. The lowest rate of incidental thyroid cancer (6.1%) is found in patients with nodular Graves’ disease [16, 48]. In populations with iodine deficiency, there is a higher risk of endemic goiter and a higher risk of thyroid cancer. Specifically, there are more follicular and anaplastic carcinomas in iodine-deficient populations, whereas there appears to be a higher rate of PTC in populations with high iodine intake [49]. Meta-analysis has also shown PTC to be associated with Hashimoto thyroiditis [50].
Evaluation of Patients with a Thyroid Nodule
History and Physical Examination
The evaluation of a patient with a thyroid nodule should begin with a history and physical examination. A history of significant radiation exposure or a family history of thyroid cancer both increases the risk of thyroid cancer as well as influences the decision regarding the extent of surgery because in either case the entire gland is at risk for the development of cancer. A history of symptoms of possible local invasion including voice hoarseness, progressive dysphagia, dyspnea, or hemoptysis should be sought.
Physical examination has notoriously poor sensitivity and specificity for the detection of thyroid cancer [51]. In addition, many patients with thyroid cancer have no signs or symptoms. Consequently, PTC is often incidentally discovered. The physical examination of a patient with a thyroid nodule should pay particular attention to thyroid gland size, mobility, presence of tracheal deviation, substernal extension, and cervical or supraclavicular lymphadenopathy. Physical exam findings that are worrisome for thyroid cancer include fixation of a thyroid nodule, cervical lymphadenopathy (especially ipsilateral to a thyroid nodule), and vocal cord paralysis (also, particularly when ipsilateral to a thyroid nodule). Approximately 10% of patients with differentiated thyroid cancer present with invasion of local structures, and these patients are at a twofold higher risk of recurrence and have increased mortality rates [22, 52]. Although palpation of the neck nodal basins is routine, physical examination is often unreliable for the detection of abnormal neck lymph nodes. In studies evaluating patients with differentiated thyroid cancer with nonpalpable lymph nodes on physical examination, neck ultrasound detected abnormal lymph nodes in 16–34% of patients [53, 54].
The quality of the speaking voice should be documented in the physical exam. A paralyzed or paretic vocal fold will often manifest as dysphonia; however, a normal voice is no guarantee that vocal fold motion is normal [55]. Up to 8% of patients with thyroid cancer present with a vocal fold paralysis. The majority of these patients will be symptomatic with breathy dysphonia and/or aspiration; however, up to one-third of patients may be completely asymptomatic. Preoperative laryngeal exam is indicated for patients with voice abnormalities, a history of neck or chest surgery during which the recurrent laryngeal or vagus nerves are at risk for injury, or thyroid cancers with posterior extrathyroidal extension or extensive paratracheal lymph node metastases [21]. Some experts recommend routine laryngeal exam for all patients [56]. The finding of preoperative laryngeal paralysis influences extent of surgery and has medicolegal implications.
Role of Ultrasonography in the Evaluation of Thyroid Nodules
Due to the poor sensitivity and specificity of physical exam for thyroid cancer, ultrasound has become an extension of the physical exam. High-resolution ultrasound is the optimal imaging study for the characterization of the primary thyroid tumor and the status of the regional nodal basins [21]. Ultrasound can detect lesions as small as 1–2 mm within the thyroid and can be used to distinguish cystic from solid, determine blood flow characteristics through Doppler, accurately measure size of lesions, and give information that increase or decrease suspicions for malignancy in a thyroid nodule or cervical lymph node. Recently, ultrasound of the larynx has been found to be an accurate and reproducible method to screen for vocal fold motion abnormalities [57].
Advantages to ultrasound over other imaging modalities include low cost, portability, speed, lack of exposure to radiation, and accessibility to those outside of a radiology department. It is highly operator dependent, however. Compared to cross-sectional imaging modalities (e.g., CT and MRI), ultrasound has a higher sensitivity and specificity and does not require exposure to radiation or placement within uncomfortable confined spaces.
Several sonographic features of thyroid nodules that have a high specificity for PTC have been recognized. PTC usually manifests as a solid nodule, but also is rarely found within the mural component of a predominantly cystic nodule. PTC is usually sonographically hypoechoic, but can also appear isoechoic or hyperechoic. Microcalcifications, or small bright reflectors without reverberation artifact or posterior acoustic shadowing, are commonly seen in both PTC and medullary thyroid cancer. There is considerable interobserver variability in the differentiation of true microcalcifications from other more benign findings of cystic interface or inspissated colloid. The simultaneous detection of a hypoechoic solid nodule with microcalcifications has a high positive predictive value for thyroid cancer [58]. A meta-analysis of 52 observational studies found that the features with the highest specificity for malignancy were nodules taller than wide in axial imaging (i.e., the anteroposterior measurement is greater than the transverse measurement), microcalcifications, or an irregular margin [59].
Fine Needle Aspiration Biopsy
Fine needle aspiration (FNA) biopsy is the first test of choice for thyroid nodules that meet specific size or imaging criteria [21]. FNA biopsy for cytology is highly accurate for the detection of PTC [60, 61]. In 2007 a State of the Science Conference was held at the National Institutes of Health in Bethesda, Maryland, in order to develop consensus guidelines on how to classify cytologic specimens from thyroid FNA biopsies [62]. Briefly, there is a six-tiered scheme for categorizing thyroid FNA results with defined criteria and an associated specific risk of cancer for each category. Expert guidelines detail recommendations for the interpretation and management of cytology results based on these Bethesda categories [21]. A detailed description of the Bethesda categories can be found elsewhere in this text. Furthermore, gene expression classifiers and gene panel tests designed to detect specific DNA mutations and RNA rearrangements in FNA aspirates are commercially available and are also described elsewhere.
Evaluation of Patients with Biopsy-Proven PTC
Laboratory Tests
Measuring thyrotropin (TSH) is useful in the preoperative evaluation of a patient with biopsy-proven PTC. Several studies have demonstrated that an elevated TSH is an independent risk factor for the development of thyroid cancer [19, 63–66]. Perhaps one of the most interesting findings from these studies is that the risk of thyroid cancer was higher than expected for patients with a TSH at the upper end of the normal range [65, 66]. The lowest risk of thyroid cancer appears to be for patients with a low TSH. Conversely, there is a stepwise linear increase in the risk of thyroid cancer within the population as the TSH rises. TSH levels were found to be significantly higher in patients with stage III and IV thyroid cancer compared to those with stage I and II thyroid cancer in one study [66] and are also associated with the presence of gross extrathyroidal extension and lymph node metastases [67]. It is hypothesized that higher TSH levels affect signaling pathways associated with more aggressive thyroid cancer behavior [67]. Detecting elevated TSH before surgery also impacts the risk of hypothyroidism following lobectomy and also may be an indication for total thyroidectomy. A preoperative diagnosis of hyperthyroidism or hypothyroidism might also influence the timing of surgery because either condition should be managed prior to a general anesthetic.
Expert guidelines clearly state that assessment of serum thyroglobulin (TG) and anti-thyroglobulin (anti-TG) levels are indicated only in the surveillance of patients with differentiated thyroid cancer and are not sensitive or specific for the diagnosis of thyroid cancer, or the prediction of the stage of disease [21]. TG is a protein made by both thyroid follicular cells and differentiated cancers that arise from follicular cells. The amount of TG circulating in the bloodstream is proportional to the number of benign and well-differentiated malignant follicular thyroid cells present and the status of the TSH receptor on the surfaces of those cells. TG is not specific for thyroid cancer; many benign conditions are associated with an elevation in TG including goiter, thyroiditis, and autoimmune thyroid disease. Approximately 10% of the population has detectable anti-TG antibodies, whereas approximately 20% of patients with PTC have anti-TG antibodies [68, 69]. The presence of anti-TG interferes with the immunometric assay for TG and prevents an accurate measurement of TG [69]. In patients in surveillance for thyroid cancer, the anti-TG titer may be used as a surrogate marker of the amount of TG present, but it may not reliably estimate the true volume of disease [69, 70]. TG is not elaborated by medullary thyroid cancers (MTC) or anaplastic thyroid cancers (ATC) and, therefore, is not useful for monitoring those cancers.
Other laboratory tests have been employed in an attempt to influence the surgical or postoperative management of a patient with PTC, but data supporting their use have been mixed. Some authors have proposed testing for BRAF-V600E mutation status and using this information to decide when to perform prophylactic central neck lymph node dissection, as some studies have shown an association between this mutation and the presence of lymph node metastases [71, 72]. Other studies, however, have not found this association [73, 74], and the most recent version of the American Thyroid Association (ATA) guidelines state that BRAF-V600E mutation status should not be used to influence the decision to perform prophylactic central compartment nodal dissection [21].
Screening for hyperparathyroidism is recommended for all patients before thyroidectomy for any indication, since hyperparathyroidism can be addressed at the time of thyroidectomy and may necessitate a bilateral exploration [75].
Imaging Studies in the Patient Diagnosed with PTC: Ultrasound
Because PTC first metastasizes to the cervical lymph nodes, the initial imaging test recommended in the preoperative evaluation of a patient with PTC is cervical ultrasound, which should include the thyroid and both the central and lateral compartments of the neck [21]. Ultrasound can characterize the number, size, location, and appearance of cervical lymph nodes. Under normal circumstances the nodal basins of the lateral neck should appear symmetric. Normal cervical lymph nodes are usually flattened and elongated, are never cystic, and are usually homogenous. The lymphovascular pedicle can be demonstrated sonographically in most benign lymph nodes and appears as a hyperechoic “fatty” hilum. The following sonographic features of lymph nodes are concerning for metastatic disease: enlarged or rounded shape, loss of the fatty hilum, calcifications, cystic changes, or peripheral vascularity [76–78]. Ultrasound has higher sensitivity for the identification of lateral compartment nodal metastases compared to central compartment nodal metastases. The sensitivity of ultrasound in the central neck is limited by the overlying thyroid gland, the tracheal air shadow, the clavicle, and the sternum [79, 80].
Abnormal lymph nodes should undergo ultrasound-guided FNA biopsy. An adjunct to the cytologic evaluation of an abnormal lymph node is the measurement of lymph node aspirate TG, particularly in cases when the FNA yields an inadequate number of cells for cytologic evaluation or when a sonographically suspicious lymph node has benign cytology [81, 82]. Measurement of TG in a lymph node aspirate can increase the accuracy of cytology (sensitivity of 95% and specificity of 94.5%) and is recommended in expert guidelines, but is not mandatory [60]. Limitations in the use of TG “washouts” include that there is no standardization of this procedure, false positives may occur in patients in whom the thyroid gland is still in place, and circulating anti-TG antibodies can theoretically interfere with the measurement of TG [83].
Additional Imaging Studies in the Patient Diagnosed with PTC: Cross-Sectional Imaging
Cross-sectional imaging tests (e.g., CT, MRI) may also be indicated in the preoperative evaluation of a patient with PTC, particularly when there is a fixed thyroid mass, concern for substernal extension, aerodigestive tract invasion, or bulky lymphadenopathy [21]. CT or MRI generates high-resolution images that can be reconstructed in multiple planes [84, 85] and can therefore display the full extent of the primary tumor and its effect on adjacent structures, including the presence of aerodigestive tract invasion or invasion or encasement of the internal jugular vein or carotid artery. Cross-sectional imaging can also detect lymph node metastases in areas that are poorly assessed by ultrasound (i.e., the retropharyngeal or retrotracheal space and the inferior central neck/superior mediastinum) [85]. Furthermore, technical limitations such as kyphosis, obesity, and other neck pathology may limit the ability of ultrasound to characterize the entire thyroid and cervical nodal basins. CT is the primary modality for the evaluation of lung parenchymal metastases and mediastinal lymphadenopathy. However, these events are sufficiently rare enough that it is not necessary to routinely obtain a CT of the chest for staging thyroid cancer, unless indicated by symptoms or physical exam findings. The main limitation of CT is that the iodine load associated with the intravenous contrast may affect the timing of postoperative radioactive iodine treatment [86, 87]. The main limitation of MRI is that respiratory motion artifact can affect the clarity of the structures in the paratracheal and retrosternal spaces [88].
Additional Imaging Studies in the Patient Diagnosed with PTC: Nuclear Medicine Studies
Approximately 35% of nodules with focal 18-fluorodeoxyglucose (18-FDG) uptake on PET scans are malignant [89]. Despite this fact, 18-FDG PET has not been found to be expedient for the detection of thyroid cancer because many well-differentiated PTCs are not FDG avid. 18-FDG PET is useful in the evaluation of poorly differentiated, recurrent, or radioiodine-negative thyroid cancer. However, its utility in the initial detection of PTC or the preoperative staging of PTC is limited by false positives and decreased specificity [90].
Treatment of PTC: Implications of Tumor Size, Multifocality, and Metastatic Disease
Some patients with small-volume, low-risk cancers are being actively observed as part of research protocols [4]. In addition, some patients with low-volume disease have been treated with forms of lesion-directed therapy such as ethanol ablation, radiofrequency ablation [91], or percutaneous laser ablation [92]. A discussion of protocols used at some institutions for lesion-directed therapy or active observation is outside of the scope of this chapter, and the remainder of this chapter will instead focus on the factors that impact decisions about surgery in patients with biopsy-proven PTC.
Hemithyroidectomy is the preferred extent of surgery for well-differentiated papillary cancer less than 1 cm in diameter that is confined to the thyroid parenchyma in one lobe without overt evidence of metastatic disease [21]. The incidental finding of such a lesion in a hemithyroidectomy specimen, therefore, is usually not an indication for completion thyroidectomy.
Hemithyroidectomy and total thyroidectomy are acceptable surgical procedures for patients with PTC between 1 and 4 cm (T1 or T2 primary tumors) limited to one thyroid lobe, without overt evidence of metastatic disease [21]. This is a change from the previous version of the ATA guidelines, which recommended total thyroidectomy for nearly all PTCs > 1 cm without evidence of lymph node or distant metastases [93]. However, one issue that is not specifically addressed by both the most recent ATA guidelines recommendation and the AJCC staging system for PTC is that of multifocality. PTC is frequently multifocal, occurring in 18–87% of cases, and is usually characterized by a dominant tumor measuring >1 cm, with most of the remaining foci measuring <1 cm [94]. Multifocality has been found to be associated with a higher risk of lymph node metastases and regional recurrence compared to patients with unifocal disease [95–97]. Because contralateral foci of PTC in multifocal PTC are usually <1 cm, they are often an incidental pathologic finding in patients who underwent total thyroidectomy and are usually not identified at the time of initial diagnosis. Nevertheless, hemithyroidectomy is proposed to be adequate treatment for multifocal PTC with a dominant T1–T2 primary tumor, as rates of completion thyroidectomy have been reported to be <10% and recurrences do not appear to impact survival [98–100]. More investigation may be required to clarify the implications of multifocality and to guide clinical care when it is discovered.
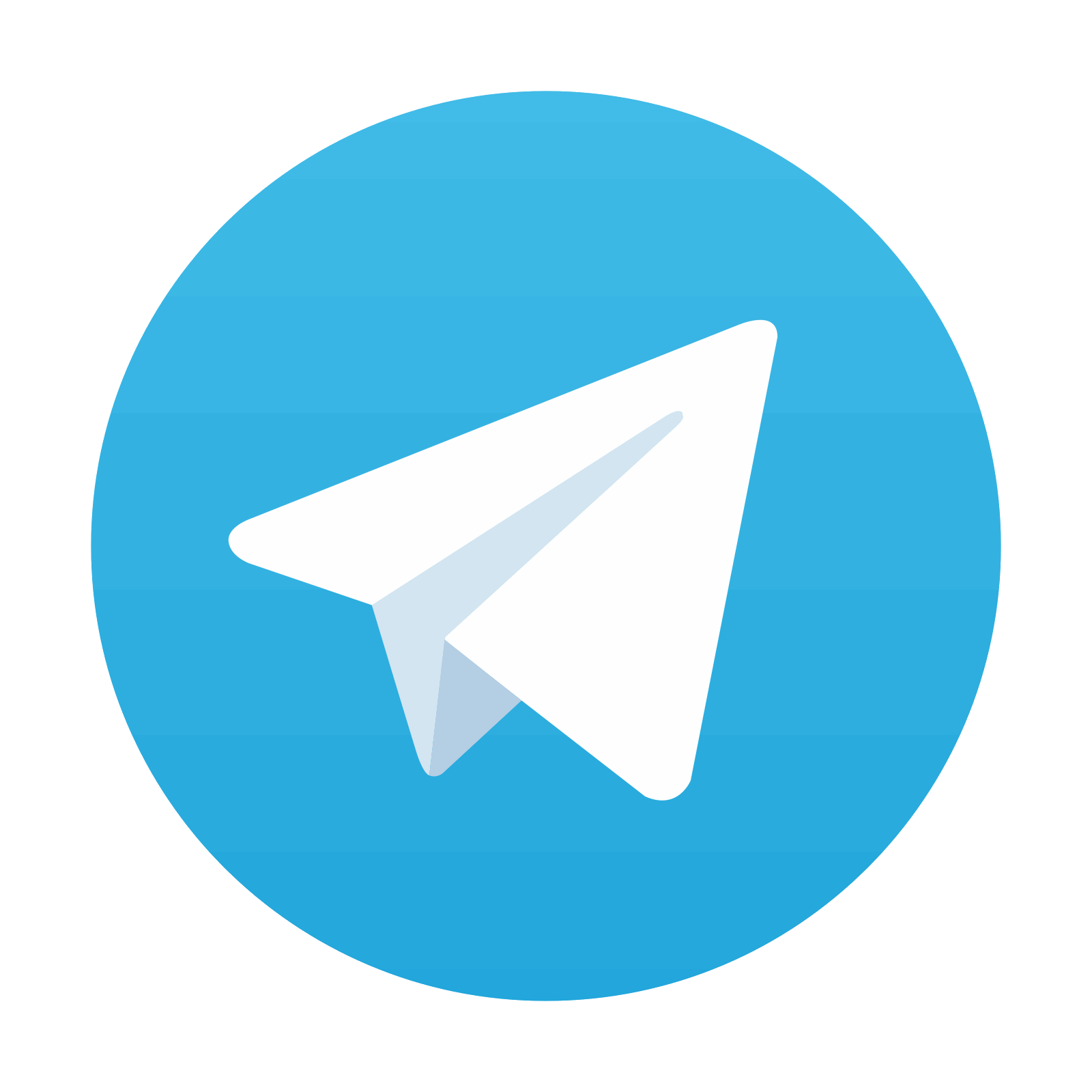
Stay updated, free articles. Join our Telegram channel

Full access? Get Clinical Tree
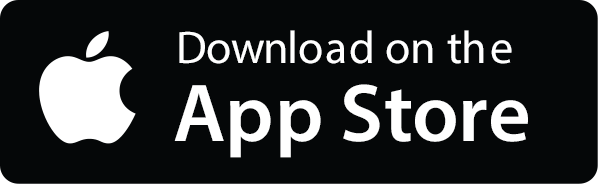
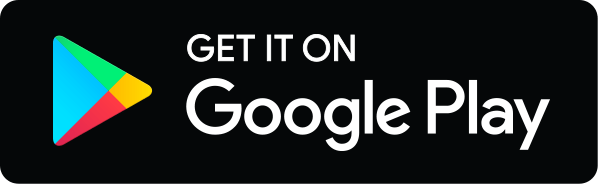