© Springer International Publishing Switzerland 2015
Varsha Gandhi, Kapil Mehta, Rajesh Grover, Sen Pathak and Bharat B. Aggarwal (eds.)Multi-Targeted Approach to Treatment of Cancer10.1007/978-3-319-12253-3_2121. Chemopreventive and Anticancer Efficacy of Silibinin Against Colorectal Cancer
(1)
Department of Pharmaceutical Sciences, Skaggs School of Pharmacy and Pharmaceutical Sciences, University of Colorado Anschutz Medical Campus, Aurora, CO 80045, USA
(2)
University of Colorado Cancer Center, University of Colorado Anschutz Medical Campus, Aurora, CO 80045, USA
(3)
Department of Pharmaceutical Sciences, Skaggs School of Pharmacy and Pharmaceutical Sciences, 12850 E. Montview Blvd, C238, Room V20-2117, Aurora, CO 80045, USA
Abstract
The risk of developing colorectal cancer (CRC) as well as the incidence of CRC-associated mortality is increasing globally. It is thus imperative that we look at alternative nontoxic natural measures which can be used by all, either as a prevention measure or as a safe intervention strategy at any time during the course of the clinical treatment of this deadly malignancy. The focus of the present review is to encompass the efficacy and mechanisms of silibinin (a flavonolignan isolated from the seeds of milk thistle, Silybum marianum) against CRC. Over the years, preclinical studies have shown that silibinin has strong preventive and therapeutic efficacy against various epithelial cancers, including CRC. Given its high bioavailability in colonic tissues of CRC patients, and the fact that silibinin consumption is exceptionally safe, these studies advocate the efficacy evaluation of silibinin in clinical trials, both as a CRC preventive agent and as an “adjunct therapy” for uses in clinical cases where current treatment approaches fail to cause absolute suppression of CRC.
Keywords
Colorectal cancerSilibininCancer chemopreventionGrant Support
Supported by NIH RO1 grant CA112304.
21.1 Introduction
Over the years, colon screening as a colorectal cancer (CRC) prevention strategy, molecular/biomarker analysis, and chemotherapy interventions have had a major impact on CRC management in the USA (National Cancer Institute 2013). However, the risk of developing CRC is also increasing at an alarming rate in the USA as well as globally; medical conditions, such as inflammatory bowel disease, obesity, and associated type 2 diabetes, together with a lack of active lifestyle/exercise and an increase in the intake of high-calorie processed foods, are all well-recognized contributing factors to the increase in CRC risk (Center et al. 2009). Also, the colon screening initiatives are underused in the USA, resulting in some of the CRC cases in their initial preneoplastic stages to be left undiagnosed, such that it results in their uninhibited progression to more advanced forms of the disease; the eventual distant metastasis results in an increase in CRC mortality (National Cancer Institute 2013). Given that statistical estimates for 2014 indicate ~136,830 new CRC cases and 50,310 associated deaths (American Cancer Society 2014), clearly we are still behind in our efforts to overcome the risk to develop CRC or to control the increased mortality associated with this malignancy. It is thus imperative that we look at alternative nontoxic natural measures which can be used by all, either as a prevention measure or as a safe intervention strategy at any time during the course of the clinical treatment of this deadly malignancy (Raina and Agarwal 2013; Rajamanickam and Agarwal 2008).
In this regard, several research groups worldwide have focused their efforts to develop interventions which can reduce the incidence of preneoplastic adenomatous polyps and/or prevent their progression to more advanced forms of CRC (Rajamanickam and Agarwal 2008). While the chemopreventive potential of a wide range of agents against CRC is under investigation (Rajamanickam and Agarwal 2008; Derry et al. 2013), the focus of the present review is to encompass the efficacy and mechanisms of one such chemopreventive agent, i.e., silibinin, against CRC. Silibinin (a flavonolignan isolated from the seeds of milk thistle, Silybum marianum) is a very promising cancer chemopreventive agent and has shown strong preventive and therapeutic efficacy against various epithelial cancers, including CRC, in different preclinical models (Rajamanickam and Agarwal 2008; Raina and Agarwal 2007; Ramasamy and Agarwal 2008; Ting et al. 2013); notably it is a widely consumed nutraceutical supplement for liver ailments (considered safe for human consumption and has a long history of human use) as well as used clinically for hepatotoxicity in various parts of the world including the USA (Polyak et al. 2010). Regarding the efficacy of silibinin against CRC, as elaborated in detail in the following pages, several preclinical studies have demonstrated that silibinin has strong preventive and therapeutic efficacy against this malignancy.
21.2 Silibinin Efficacy in CRC Cell Lines
Treatment of CRC cells, viz., HT29, LoVo, and SW480 with different concentrations of silibinin ranging from 25 to 200 μM, for 24–72 h has been reported to cause a concentration- and time-dependent decrease in growth and proliferation of these cells (Agarwal et al. 2003; Kaur et al. 2009, 2010; Raina et al. 2013a). While silibinin significantly decreased the total cell numbers by inducing cell cycle arrest at either G1 or G2M phases depending upon concentration and CRC cell type, a considerable cell death was observed only at the highest concentration (200 μM) of silibinin or after longer incubation with lower concentrations (Agarwal et al. 2003; Kaur et al. 2009, 2010; Raina et al. 2013a). Mechanistic studies in HT29 cells have shown that silibinin-induced G0/G1 arrest was associated with an increase in both mRNA and protein levels of cyclin-dependent kinase (CDK) inhibitors Kip1/p27 and Cip1/p21, and a strong inhibition in CDK-2 and CDK-4 kinase activity, together with a decrease in CDK-2 and CDK-4 and cyclin-D1 and E protein levels (Agarwal et al. 2003). In G2M arrested cells, silibinin decreased the protein expression of cell cycle regulators cdc25C, cdc2/p34, and cyclin B1 and increased the kinase activity of cdc2/p34 molecule (Agarwal et al. 2003). Furthermore, silibinin-induced death in HT29 cells through apoptosis was independent of caspase pathway (Agarwal et al. 2003). These effects of silibinin on cellular proliferation and cell cycle regulatory molecules were also corroborated by similar findings in other CRC cell lines (Fet, Geo and HCT116) by Hogan et al. (2007) and in HT29 cells by Akhtar et al. (2014) as well as Karim et al. (2013). Another investigation reported that apoptosis induction by silibinin in HT29 CRC cells was through early growth response-1 (EGR-1)-mediated nonsteroidal anti-inflammatory drug-activated gene-1 (NAG-1) upregulation (Woo et al. 2014). In contrast, the cell death induced by silibinin in LoVo cells has been shown to be caspase dependent and associated with increased levels of cleaved caspase-3 and caspase-9 and cleaved PARP (Kaur et al. 2009). Similar to the effect in HT29 cells, silibinin increased the levels of Kip1/p27 and Cip1/p21 in LoVo cells and decreased the level of cyclin-D1, cyclin-D3, cyclin-A, and cyclin-B1 and CDK-1, CDK-2, CDK-4, and CDK-6 (Kaur et al. 2009). Phosphorylation of retinoblastoma (Rb) protein was also decreased by silibinin without significantly affecting the total Rb levels in LoVo cells (Kaur et al. 2009).
21.3 Silibinin Efficacy Against the Growth of CRC Xenografts
Preventive and/or therapeutic efficacy of silibinin against CRC xenografts in athymic nude (nu/nu) balb/c mice has also been studied (Kaur et al. 2009, 2010). In these studies, oral feeding of silibinin for 6 weeks (at doses: 100 and 200 mg/kg/day) significantly inhibited the growth of human CRC LoVo and SW480 xenografts (P < 0.001) (Kaur et al. 2009, 2010). These xenograft studies were followed by another investigative mice study with an objective to compare the preventive versus the therapeutic efficacy of silibinin against established human CRC xenografts (Velmurugan et al. 2010). In one group of nude mice, silibinin (200 mg/kg body weight) was orally fed for 28 days immediately after xenograft implantation; thereafter, silibinin was discontinued and tumor growth was monitored for another 3 weeks (Velmurugan et al. 2010). In another group of mice, silibinin treatment was started after the tumors were well established and tumors were harvested as a function of time, after silibinin treatment (Velmurugan et al. 2010). Results from both treatment protocols showed that silibinin, used either as a preventive or as a therapeutic measure, has an inhibitory effect on tumor growth and that even after its withdrawal, a strong and sustained inhibitory effect on the growth of xenografts persists (Velmurugan et al. 2010). Furthermore, analyses of xenograft tissues from various efficacy studies have shown that inhibition of tumor growth by silibinin is unequivocally associated with a decrease in cellular proliferation and an increase in apoptosis in tumors (Kaur et al. 2009, 2010; Velmurugan et al. 2010; Singh et al. 2008). In yet another human CRC xenograft study, pure silibinin and its phosphatidylcholine form [silibinin-phytosome (Siliphos™—2:1 ratio of phosphatidylcholine and silibinin, w/w) formulation from Indena (Seattle, WA, USA) that has shown better bioavailability as compared to pure silibinin (Kidd and Head 2005)] were compared for their efficacy against human CRC xenografts in nude mice (Singh et al. 2008). While oral feeding with both decreased tumor volume and tumor weight after 32 days, the inhibitory efficacy displayed by silibinin-phytosome was better than that of silibinin (Singh et al. 2008).
21.4 Efficacy of Silibinin in Carcinogen-Induced Sporadic CRC in Rodent Models
Velmurugan et al. have reported that silibinin-supplemented (0.033–1 %, w/w) diets prevent azoxymethane (AOM)-induced formation of preneoplastic lesions, aberrant crypt foci (ACF), in Fisher 344 rats (Velmurugan et al. 2008). Both pre- and post-initiation treatment protocols with silibinin feeding were followed in this animal study (Velmurugan et al. 2008). Briefly, in pre- and post-initiation protocol, Fisher 344 rats were fed control or silibinin in diet for 2 weeks before AOM injection, and the supplemented diets continued even after AOM injections (two injections one week apart) till study ends (8 weeks post last AOM injection). In post-initiation protocol, silibinin treatments were started 2 weeks after AOM injection. In both protocols, a dose-dependent effect of silibinin was observed; silibinin feeding effectively reduced the mean number of AOM-induced ACF and crypt multiplicity [especially large crypts (≥4 crypts)], though the percentage of inhibition was stronger in animals on the pre- and post-initiation protocol (Velmurugan et al. 2008). These results are consistent with earlier studies, wherein Kohno et al. (2002) have reported the efficacy of dietary feeding of silymarin against incidence and the multiplicity of colonic adenocarcinoma during the initiation/post-initiation phase of AOM-induced colon tumorigenesis. In yet another long-term study by Ravichandran et al., oral silibinin feeding (250 and 750 mg/kg body wt) showed more effective prevention of AOM-induced colon tumorigenesis in A/J mice when used in pre- in comparison to posttreatment regime (Ravichandran et al. 2010). Efficacy of silibinin (300 mg/kg body wt) against AOM-induced hyperproliferative crypts and ACF has been also demonstrated in Wistar rats after 7 weeks of post-initiation treatment (Kauntz et al. 2012a). On similar lines, silibinin has shown strong anticancer efficacy against 1,2-dimethylhydrazine (DMH)-induced colon tumorigenesis in Wistar rats (Sangeetha et al. 2009, 2010a, b, 2012); these anti-CRC effects were also associated with a reduction in fecal-transforming microbial enzymes, a reduction in lipid peroxidation in colonic tissues, and a decrease in phase I xenobiotic metabolizing enzymes (Sangeetha et al. 2009, 2010a, b, 2012).
21.5 Efficacy of Silibinin in Apcmin/+ Mice CRC Model
Efficacy of silibinin against CRC has also been studied against spontaneous intestinal tumorigenesis in Apc min/+ mice model, in both short- and long-term intervention studies (Karim et al. 2013; Rajamanickam et al. 2009, 2010; Verschoyle et al. 2008). In short-term study, different doses of silibinin (250–750 mg/kg body weight) were used by Rajamanickam et al. to orally gavage Apc min/+ mice for 6 weeks, after which total intestinal polyps were counted (Rajamanickam et al. 2009). However, in long-term study, only the highest dose of silibinin was used under similar treatment protocol, except that mice were sacrificed after 13 weeks of silibinin treatment (Rajamanickam et al. 2010). Silibinin feeding strongly reduced the number and size of polyps formed in proximal, middle, and distal portions of small intestine in both protocols (Rajamanickam et al. 2009, 2010). Colon polyps were also observed in the long-term study, which were also significantly reduced by silibinin treatment (Rajamanickam et al. 2010). Most importantly, no polyps with size >3 mm were observed in silibinin-fed mice; there was also a ~92 % decrease in polyp numbers with size range >2–3 mm by silibinin (Rajamanickam et al. 2010). These inhibitory effects of silibinin against CRC were associated with a decrease in proliferation and an increase in apoptosis in polyps only; the normal crypt-villus regions of the intestine were unaffected (Rajamanickam et al. 2010).
21.6 Cellular and Biological Mechanisms Associated with Inhibitory Effects of Silibinin on Growth and Progression of CRC
Using tissues from all in vivo studies and through detailed in vitro investigations, various studies have examined and defined that silibinin targets expression of various molecules regulating cell cycle, cell survival, autophagy, apoptosis, angiogenesis, and inflammation in its efficacy against colon tumorigenesis. The results from these studies are summarized below:
21.6.1 Mechanism of Silibinin Efficacy Against Growth and Progression of CRC Tumors
In all CRC xenografts silibinin decreased cell proliferation, induced apoptosis, and reduced angiogenic effects (Kaur et al. 2009, 2010; Velmurugan et al. 2010; Singh et al. 2008). Analyses of LoVo xenograft tissue showed that the inhibitory effect of silibinin on tumor growth was associated with a strong increase in p27 levels together with a decrease in Rb phosphorylation (Kaur et al. 2009). Detailed mechanistic studies showed that, in HT29 xenografts, antiproliferative and proapoptotic effects of silibinin were associated with downregulation of ERK1/2 and Akt phosphorylation as well as cyclin D1 expression (Singh et al. 2008). Furthermore, in SW480 cells, silibinin decreased the protein expression levels of β-catenin (both nuclear and cytoplasmic levels of ß-catenin were decreased, though the effect in the nucleus was more prominent) and its upstream molecule phospho-GSK3β (Kaur et al. 2010). Silibinin also decreased the expression of CDK-8, a CRC oncogene that positively regulates ß-catenin activity. Furthermore, ß-catenin-dependent TCF-4 transcriptional activity was significantly inhibited (Kaur et al. 2010); the expression of various molecules involved in CRC growth, survival, and angiogenesis, such as c-Myc, cyclin D1, survivin, VEGF, and iNOS, was also decreased by silibinin (Kaur et al. 2010; Velmurugan et al. 2010). A strong anti-angiogenic effect was also observed in CRC xenografts as evidenced by decreased tumor microvessel density in silibinin-treated tumors (Singh et al. 2008). Mechanistic studies in colonic polyps/tumors tissue samples also showed similar effects (Ravichandran et al. 2010; Rajamanickam et al. 2010); silibinin caused a strong decrease in β-catenin levels which was accompanied with a decrease in PCNA and cyclin D1 levels. Furthermore, a strong modulation of PI3K-Akt pathway was observed in these tissues as evidenced by elevated levels of IGFBP-3 protein and a decrease in phosphorylated levels of GSK-3β and Akt proteins together with a decrease in the expression of IGF1R-β receptor (Ravichandran et al. 2010). Furthermore, anti-angiogenic effect of silibinin in AOM-induced CRC models and Apcmin/+ mice was also associated with decrease in the expression levels of iNOS and NOS3 molecules, COX-1 and COX-2, and HIF-1α, and VEGF protein expression was also decreased in the tumors of mice treated with silibinin (Ravichandran et al. 2010; Rajamanickam et al. 2010). In vitro studies by Yang et al. using LoVo cells to delineate the mechanisms involved in the anti-angiogenic efficacy have shown that silibinin inhibits the chemotaxis migration of endothelial cells EA.hy926 toward CRC cells and decreases the vascular density index in the chorioallontoic membrane assay and that these effects are mediated by upregulation of VEGFR-1 (Flt-1) gene expression (Yang et al. 2003, 2005). Regarding the potential of silibinin to affect the invasiveness of CRC cells, studies by Lin et al. have shown that silibinin significantly decreases the cytokine IL-6-induced proliferation and invasion of LoVo cells and that these silibinin effects are mediated by a reduction in the MMP-2 promoter activity via attenuation of AP-1 binding activity (Lin et al. 2012).
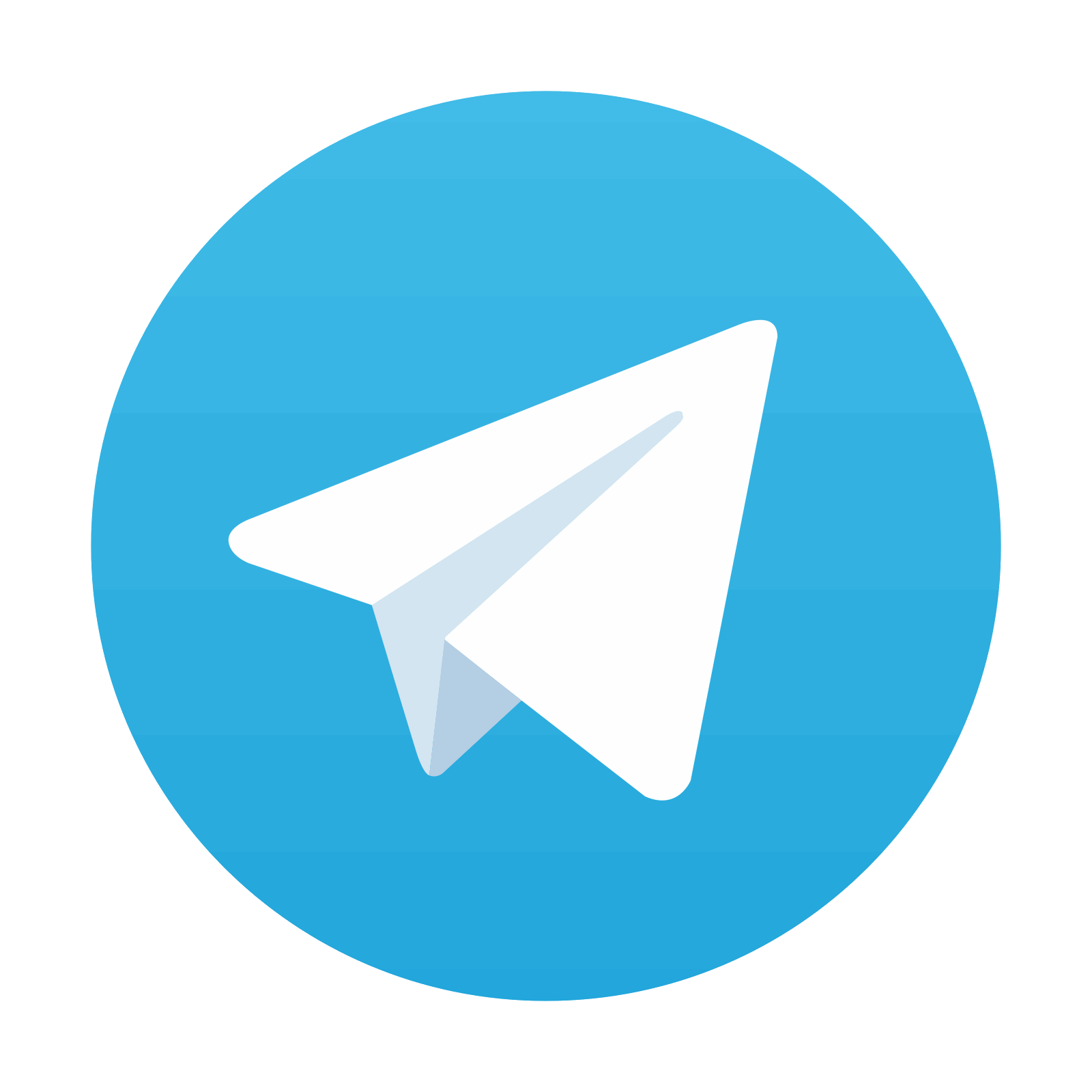
Stay updated, free articles. Join our Telegram channel

Full access? Get Clinical Tree
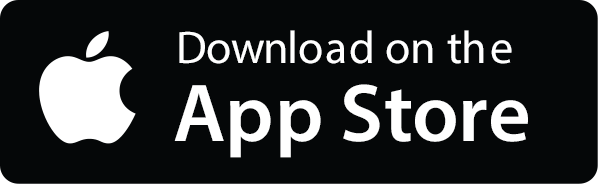
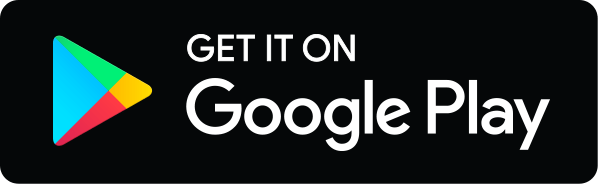