Keywords
Chemokines, antitumor immunity, immunotherapy, dendritic cells, tumor-infiltrating T cells
Introduction
Dendritic cells (DCs) are highly specialized, professional antigen-presenting cells (APCs) that activate naive T cells and are being used in cell-based vaccines for cancer. Chemokines or chemoattractant cytokines are a large family of low-molecular-weight molecules that are classified into four groups based on the first two cysteines adjacent to the amino-terminus: C, CC, CXC, and CX 3 C. Because DCs are the most potent activators of T cells of all APCs and chemokines direct the migration of cells with strong antitumor properties, combining them is an attractive strategy for potentially improving immune-based therapies for cancer. In this chapter, we review the potential role of chemokines introduced into the tumor microenvironment combined with the antigen-presenting qualities of DCs for both generating and enhancing specific T cell-mediated antitumor immunity. We first discuss the biology of particular chemokines that may be useful to recruit immune cell subsets to the tumor microenvironment and on DCs as a vehicle to mount a robust immune response to tumors. We then discuss preclinical studies that describe and support a novel gene therapy approach combining chemokines and DCs to eliminate tumors in vivo . Lastly, we introduce early clinical trials based on this promising gene therapy approach.
Background
During an immune response, DCs uptake and process antigens at the site of infection, migrate to the nearest lymph node, and present the processed antigens in the context of major histocompatibility complex (MHC) class I and class II molecules to resident naive T cells. This interaction results in the activation and subsequent expansion of the T cells followed by their trafficking to, and accumulation in, the site of infection (in some cases through chemokine gradients), which ultimately lead to eradication of the pathogen. These basic processes are critical components of an adaptive immune response. In addition to infection, the immune system can also recognize tumor as foreign through the process of immunosurveillance , and this identification can lead to an adaptive immune response against it.
However, tumors have developed ways to suppress the immune response. For example, as the tumor grows, immunosuppressive host-derived cell types such as tumor-associated macrophages, myeloid-derived suppressor cells, and CD4 + CD25 high Foxp3 + regulatory T cells (Tregs) increase in number. This corresponds to an increase in anti-inflammatory cytokines such as transforming growth factor-β (TGF-β), interleukin-4 (IL-4), IL-10, and prostaglandins. There is also a reduction in the proinflammatory cytokines such as IL-2 . This immunosuppressive environment has a profound inhibitory effect on T cell activity within it. However, it has been shown that T cells removed from the tumor are not defective per se because they can be rescued to mount a specific antitumor immune response after in vitro culturing with proinflammatory cytokines. The existence and therapeutic potential of tumor-infiltrating lymphocytes (TILs), including CD4 + and CD8 + T cells specific for tumor-associated antigens (TAAs) , has been well documented in this regard. The fact that there are preexisting tumor-specific T cells present within the tumor argues that at one point the immune system recognized the mutated cells as foreign. Altering the microenvironment is one of the key attributes that the tumor must possess in order to effectively elude the immune system and use it to its advantage. However, even in the presence of tumor-induced suppressive factors, specific immune responses are observed in patients treated using immunotherapeutic strategies . In fact, the use of genetically modified cells to express IL-7 , CCL21 , and interferon-γ (IFN-γ) inducible protein 10 (IP-10) molecules shown to aid in the development of an effective antitumor adaptive immune response has, in some instances, effectively overcome the barrier of immunosuppression. To this end, novel strategies have been used (described later) to recruit immune cells to the tumor microenvironment in order to alter the cellular and biochemical balance toward one more favorable to an ongoing, effective antitumor immune response .
The following is a critical question: What are the most important immune components that would drive a cell-based adaptive immune response to effectively eradicate tumors? As mentioned previously, effector T cells can actively eliminate a tumor, and APCs such as DCs residing in the tumor process and present tumor antigens, leading to effective T cell activation. Indeed, tumor-specific T cells are often found within and at the peripheral edge of tumors as well as in tertiary lymphoid structures within the tumor that have been shown to actively mount an antitumor immune response . As previously mentioned, the priming and activation of T cells generally occurs within the peripheral lymph node(s), with their subsequent trafficking to the tumor. However, we and others have shown that T cells can be primed extranodally . Successful attempts have been made to actively recruit immune cells to the tumor by harnessing the biologic properties of chemokines to potently and selectively recruit leukocytes, alter the tumor vasculature, and stimulate an antitumor immune response . Although we acknowledge that the existing literature showing that, in some cases, chemokine expression at the site of a tumor may also have detrimental effects in cancer patients (e.g., in metastasis spread) , in this chapter we mainly focus on the beneficial effects that chemokines have in the development of an effective immune response to cancer as well as their use in gene therapy approaches.
Chemokines in Cancer
Chemokines are secreted by a variety of cells, including tissue leukocytes, fibroblasts, endothelial cells, and epithelial cells, and they selectively direct the migration of leukocytes via locally high concentration gradients . Chemokine expression is often induced by inflammatory cytokines, cellular growth factors, and factors produced and secreted by pathogens during an immune response in infection . Chemokines interact with their corresponding G protein-coupled receptors on a variety of responding cell types, including macrophages, DCs, T cells, natural killer (NK) cells, B cells, monocytes, as well as tumor cells . Chemokines are functionally classified into inflammatory and homeostatic chemokines. Inflammatory chemokines recruit leukocytes to the sites of inflammation and tissue damage, whereas homeostatic chemokines are responsible for leukocyte homing to secondary lymphoid organs, the bone marrow, and the thymus during hematopoiesis . The interaction between chemokines, their receptors, and the migration of cells is vitally important for the maintenance of homeostasis and the induction of immune responses to pathogens and tumors. A list of inflammatory chemokines, their matching receptors, the receptor cellular expression, and their respective roles in leukocyte trafficking is shown in Table 21.1 .
Chemokine | Chemokine Receptor | Receptor Cellular Expression | Recruitment Site |
---|---|---|---|
CC Chemokines | |||
CCL2 | CCR2 | Monocytes, basophils, PMN, NK, DC | Inflammation |
CCL3 | CCR1 | Memory T cells | Inflammation |
CCL4 | CCR1 | Memory T cells | Inflammation |
CCR5 | Progenitors, Th1 cells, macrophages, DC, monocytes | Th1-type inflammatory sites | |
CCL5 | CCR5 | Progenitors, Th1 cells, macrophages, DC, monocytes | Th1-type inflammatory sites |
CCL6 | CCR1 | Memory T cells | Inflammation |
CCL7 | CCR2 | Monocytes, basophils, PMN, NK, DC | Inflammation |
CCL8 | CCR1 | Memory T cells | Inflammation |
CCR2 | Monocytes, basophils, PMN, NK, DC | Inflammation | |
CCR5 | Progenitors, Th1 cells, macrophages, DC, monocytes | Th1-type inflammatory sites | |
CCL9/10 | CCR1 | Memory T cells | Inflammation |
CCL11 | CCR2 | Monocytes, basophils, PMN, NK, DC | Inflammation |
CCR5 | Progenitors, Th1 cells, macrophages, DC, monocytes | Th1-type inflammatory sites | |
CCL12 | CCR2 | Monocytes, basophils, PMN, NK, DC | Inflammation |
CCL13 | CCR2 | Monocytes, basophils, PMN, NK, DC | Inflammation |
CCR5 | Progenitors, Th1 cells, macrophages, DC, monocytes | Th1-type inflammatory sites | |
CCL14 | CCR1 | Memory T cells | Inflammation |
CCL15 | CCR1 | Memory T cells | Inflammation |
CCL16 | CCR1 | Memory T cells | Inflammation |
CCR2 | Monocytes, basophils, PMN, NK, DC | Inflammation | |
CCR5 | Progenitors, Th1 cells, macrophages, DC, monocytes | Th1-type inflammatory sites | |
CCL19 | CCR7 | T cells, B cells, DC | Secondary lymphoid organs |
CCL20 | CCR6 | Memory T cells, DC | Skin |
CCL21 | CCR7 | T cells, B cells, DC | Secondary lymphoid organs |
CCL23 | CCR1 | Memory T cells | Inflammation |
CCL25 | CCR9 | α 4 β 7 + T cells, DC, thymocytes, macrophages | Small intestine; thymocyte selection |
CXC Chemokines | |||
CXCL1 | CXCR2 | PMN, mast cells, monocytes, macrophages | Inflammation |
CXCL2 | CXCR2 | PMN, mast cells, monocytes, macrophages | Inflammation |
CXCL3 | CXCR2 | PMN, mast cells, monocytes, macrophages | Inflammation |
CXCL5 | CXCR2 | PMN, mast cells, monocytes, macrophages | Inflammation |
CXCL6 | CXCR1 | PMN, mast cells, monocytes, macrophages | Inflammation |
CXCR2 | PMN, mast cells, monocytes, macrophages | Inflammation | |
CXCL8 | CXCR1 | PMN, mast cells, monocytes, macrophages | Inflammation |
CXCR2 | PMN, mast cells, monocytes, macrophages | Inflammation | |
CXCL9 | CXCR3 | T cells (Th1>Th2) | Inflammation |
CXCL10 | CXCR3 | T cells (Th1>Th2) | Inflammation |
CXCL11 | CXCR3 | T cells (Th1>Th2) | Inflammation |
CXCL12 | CXCR4 | Most progenitor cells, T cells, B cells, PMN, monocytes, macrophages, DCs | Bone marrow and follicular B emigration; thymocyte homing; early recruitment of T cells to inflammation of the lung |
CXCL13 | CXCR5 | B cells, memory T cells | B cell follicles |
CXCL16 | CXCR6 | Memory T cells | Inflammation |
CXCL17 | DCs and monocytes | ||
C Chemokines | |||
XCL1 | XCR1 | T cells | N.A. |
XCL2 | XCR1 | T cells | N.A. |
CX 3 C Chemokines | |||
CX 3 CL1 | CX 3 CR1 | PMN, monocytes, NK, T cells | Inflammation |
Chemokine expression has been shown to have positive effects on antitumor immune responses. For example, tumor cells expressing HSP-70 secreted CCL2, CCL3, CCL4, CCL5, CCL19, CCL20, CCL25, CXCL10, and CX3CL1, resulting in the recruitment of DCs and T cells initiating antitumor immunity . Production of CCL1 and CCL17 has been shown to augment CD8 + T cell activation . Cell lines derived from glioma, medulloblastoma, and renal cell carcinomas expressing CCL2 attracted ex vivo expanded human T cells . CXCL9 produced by tumor cells is important for T cell-mediated suppression of cutaneous tumors . Genetically altering J558L (plasmacytoma) and K485 (adenocarcinoma) tumor cell lines to express CXCL10 (IP-10) mediated the recruitment of lymphocytes, neutrophils, and monocytes, which were associated with tumor eradication in subcutaneous murine models . Additional examples of positive effects of chemokines on antitumor responses are provided in a review of preclinical chemokine studies by Dell’Agnola and Biragyn . Early work investigating the possible efficacious role of chemokines to induce antitumor immunity involved the introduction of chemokines into the tumor microenvironment by stably transfecting tumor cell lines. For example, when CXCL12 (SDF-1) was transfected into myeloma cells and examined in BALB/c mice, CD4 + and CD8 + T cells infiltrated the tumor and the tumor regressed . Intratumoral injection or application of recombinant CCL2 on the surface of the tumor resulted in effective interaction of monocytes and endothelial cells within the peritumoral area of a pancreatic tumor, which was accompanied by an upregulation of intercellular adhesion molecule 1 (ICAM-1) . Furthermore, immunization of mice with tumor cells expressing CXCL12 elicited protective immunity against subsequent tumor challenge . Interestingly, colorectal cancer cells expressing high levels of CXCL16 correlated with good prognosis and increased TILs in humans . This result is not surprising because CXCR6, the receptor for CXCL16, is expressed on memory T cells and is involved in the recruitment of Th1 cells to the sites of inflammation, as illustrated in Table 21.1 . The positive predictive effect of chemokines is not limited to CCL2 and CCL5. Forced expression of the tumor necrosis factor (TNF) superfamily member LIGHT in the tumor microenvironment induces a substantial infiltration of naive T cells. LIGHT has been shown to be an important costimulatory molecule for T cell activation via interaction with its two main receptors, herpesvirus entry mediator (HVEM) and lymphtoxin β receptor (LTβR) expressed on B cells and T cells. LIGHT expression in the tumor correlated with an upregulation of CCL21 production and the expression of adhesion molecules . These infiltrating naive T cells become primed inside the LIGHT-expressing tumors, leading to the eradication of established local and distant tumors . Precedent for the positive effect of chemokines in the tumor microenvironment was established when tumors transduced to produce RANTES (CCL5) showed antitumor immunity, which was partially attributable to the recruitment of monocytes and T cells to the tumor site . Together, these studies have demonstrated the importance of chemokines for the generation of antitumor immune responses and immune cell infiltration into the tumor microenvironment.
In primary tumors and metastases, chemokines may also play a negative role, aiding the migration of tumor cells by acting as growth or survival factors, regulating angiogenesis, determining metastatic spread, recruiting immunosuppressive cells hindering the antitumor immune response, and leading to escape from immunosurveillance . A striking example is CXCR4, which is expressed in at least 23 different types of cancer originating from mesenchymal, epithelial, and hematopoietic tissues and is implicated in aiding metastatic spread. It has been shown that tumor cells from breast, pancreatic, prostate, ovarian, and lung carcinomas, glioblastoma, and neuroblastoma all express CXCR4 . Also, CCL2 and CCL5 expression in breast cancer cells had a positive correlation with macrophages, lymph node metastasis, clinical aggressiveness, and tumor progression . In mouse and human tumors, post-translational modification of CCL2 by intratumoral reactive nitrogen species led to the failure of tumor-specific T cells to infiltrate the tumor .
Notwithstanding, controversy remains regarding the negative role played by CCL2 and CCL5 within the tumor microenvironment. For example, higher serum levels of CCL2 in patients with pancreatic cancer correlate with macrophage tumor infiltration and are associated with improved survival . Immune cells expressing CCR5, the receptor for CCL5—such as Th1 cells, DCs, macrophages, and monocytes—are recruited to Th1-type inflammatory sites. CCL5 expression in patients with non-small-cell lung cancer (NSCLC) is associated with an active lymphocyte response and is a positive predictor of survival . These observations demonstrate that chemokines can have a significant dual role—that is, enhancing metastasis and coordinating tumor immunity by recruiting immune cells to the tumor. Hence, understanding the dynamic biology and therapeutic implications of chemokines by modifying the tumor microenvironment chemokine milieu and monitoring the subsequent cellular composition as a result is currently an area of active research.
Ectopic Lymph Nodes within the Tumor Microenvironment
T lymphocytes and DCs infiltrate solid human tumors and are distributed diffusely within the tumor parenchyma or localized at the peripheral rim of the tumor mass . Their in situ presence has been perceived as evidence of an ongoing immune response to tumors. It has recently been observed that infiltrating immune cells may coalesce to form organized tertiary lymphoid structures or ectopic lymph nodes, localized at the invasive edge of the tumor .
Ectopic lymph nodes structures were first observed in patients with autoimmune diseases at the sites of chronic inflammation, such as joints and lungs of rheumatoid arthritis patients, salivary glands in Sjögren’s syndrome, the pancreas in diabetics, and the central nervous system in multiple sclerosis . These ectoptic lymph node structures are composed of separate B and T cell areas and have specialized populations of DCs, well-differentiated stromal cells, and high endothelial venules . Notably, patients with the more organized ectopic follicles had the more severe autoimmune disease symptoms.
The importance of ectopic lymph nodes in the cancer setting is under active investigation. In NSCLC, ectopic lymph nodes, termed tumor-induced bronchus-associated lymphoid tissue, have follicle-like structures as well as germinal centers . Analysis of these structures showed mature DCs/T cell clusters including T-bet + Th1 T cells, which were associated with a favorable clinical outcome . In a study of 326 colorectal carcinoma patients, the presence of ectopic lymph node structures correlated with improved overall patient survival independent of tumor staging, the location of the tumor, microsatellite instability or stability, and patient treatment .
The role that chemokines may play in the formation and function of ectopic lymph nodes is an area of active research as well. Certain chemokines expressed in situ have been linked to tertiary intratumoral lymphoid structures correlating with better prognosis . In NSCLC patients, the presence of T cells was associated with CCL19, CCL21, CXCL13, CCL17, CCL22, and IL-16 chemokines and ICAM-2, ICAM-3, VCAM-1, and MAdCAM-1 adhesion molecules . In colorectal cancer patients, the presence of ectopic lymph nodes could be predicted by a unique gene-related signature comprising a 12-chemokine gene subset, including CCL4, CCL5, CCL8, CXCL9, and CXCL10 . These chemokines are partially responsible for the recruitment of immune cells such as memory T cells, Th1 T cells, macrophages, DCs, basophils, and NK cells, as delineated in Table 21.1 . Notably, CXCL13 and CCL21 have been shown to attract CD3 – CD4 + CD45 + IL-7 R + +c-Kit + lymphoid tissue inducer (LTi) cells , cells of hematopoietic origin thought to be critical for inducing Peyer’s patches , and CXCL13 is essential for lymph node development by clustering LTi cells . CXCL13 has also been reported within B cell follicles and is known to selectively recruit B cells ( Table 21.1 ). Due to the positive effects that chemokines may play in the formation of ectopic lymph nodes and immune cell recruitment, strategies to introduce chemokines into the tumor microenvironment are being explored.
Chemokines as a Component of Dendritic Cell-Based Vaccine Strategies
Dendritic Cell Vaccine Strategies
Several DC-based cancer vaccine strategies highlighted in Table 21.2 were designed to attempt to take advantage of the crucial importance of DCs in mounting effective antitumor immunity. Phase I clinical studies have found that pulsing DCs with TAA peptides such as the HLA-A2-binding MUC1 peptide in renal cell carcinoma and the oncofetal antigen α-fetoprotein (AFP) in hepatocellular carcinoma results in tumor-specific CD8 + cytotoxic T cell responses, an increase in NK cell activation, and a decrease in Tregs . A major disadvantage of peptide-pulsed DC-based vaccination is that many tumors are heterogeneous and some tumor cells may not express the vaccine-associated peptide, leading to decreased immune cell recognition and efficacy of the treatment. As the number of known human TAAs increases , pulsing DCs with multiple peptides to increase the repertoire of activated tumor-specific T cells has been explored. For example, in patients with prostate carcinoma, vaccination with DCs pulsed with peptides derived from prostate stem cell antigen, prostatic acid phosphatase, prostate-specific membrane antigen, and prostate-specific antigen (PSA) resulted in the activation of cytotoxic CD8 + T cell responses against all four antigens .
Cancer | Mode of Action and Results | Reference |
---|---|---|
Renal cell carcinoma |
| Wierecky et al . |
Prostate carcinoma |
| Waeckerle-Men et al . |
Hepatocellular carcinoma |
| Bray et al . |
Melanoma |
| Ridolfi et al . |
Colorectal |
| Barth et al . |
Pancreatic | Autologous tumor lysate-pulsed plus TNF-α and PGE 2 . Twelve patients received the vaccination, 1 partial remission, 2 remained in stable disease with an overall median survival of 10.5 months. Vaccination increased the frequency of tumor-reactive cells in all patients tested. Five patients survived more than 1 year. Patient with the highest number of reactive T cells survived the longest (56 months). | Bauer et al . |
Colorectal | Allogeneic tumor cell lysate-pulsed. Phase I trial showed vaccination was well tolerated and nontoxic. Phase II trial of 20 patients (17 with intervention) showed 4 SD (24%), 2 remaining SD. Vaccine induced increasing amounts of serum Th1 cytokines GM-CSF, TNF-α, IFN-γ, and IL-2. | Burgdorf |
Multiple myeloma | 4× intranodal weekly injections of idiotype and KLH-pulsed, CD40L-matured DCs in 9 patients. All patients mounted a Id-specific IFN-γ T cell response. IL-4 response elicited in 2, and skin DTH observed in 7. Id-specific cytotoxic T cell response in 5. Six SD at 1-year follow-up; 4 of the 6 SD still SD at 5-year follow-up. | Yi et al . |
Melanoma | Autologous tumor lysate pulsed PBMC-derived DCs cryopreserved, thawed, and administered intradermally for 6 biweekly injections in 46 stage IV patients, 34 of whom completed the priming. Vaccine well tolerated with 3 CR and 3 PR. | O’Rourke et al . |
SCLC | PBMC transduced with adenovirus encoding p53 given intradermally to 54 patients at an average dose of 3.8×10 6 DC-Ad-p53/patient. Overall, an immune response was available in 43 patients, with 18 (41.8%) showing a p53-specific response to ALVAC and 7 of 12 (58.3%) using a p53-derived peptide by ELISPOT. Two PR (3.7%) and 13 SD reported. | Chiappori et al . |
Melanoma | Formation of tertiary lymphoid structures. | |
Pancreatic | Recruitment of immune cells. | Mule |
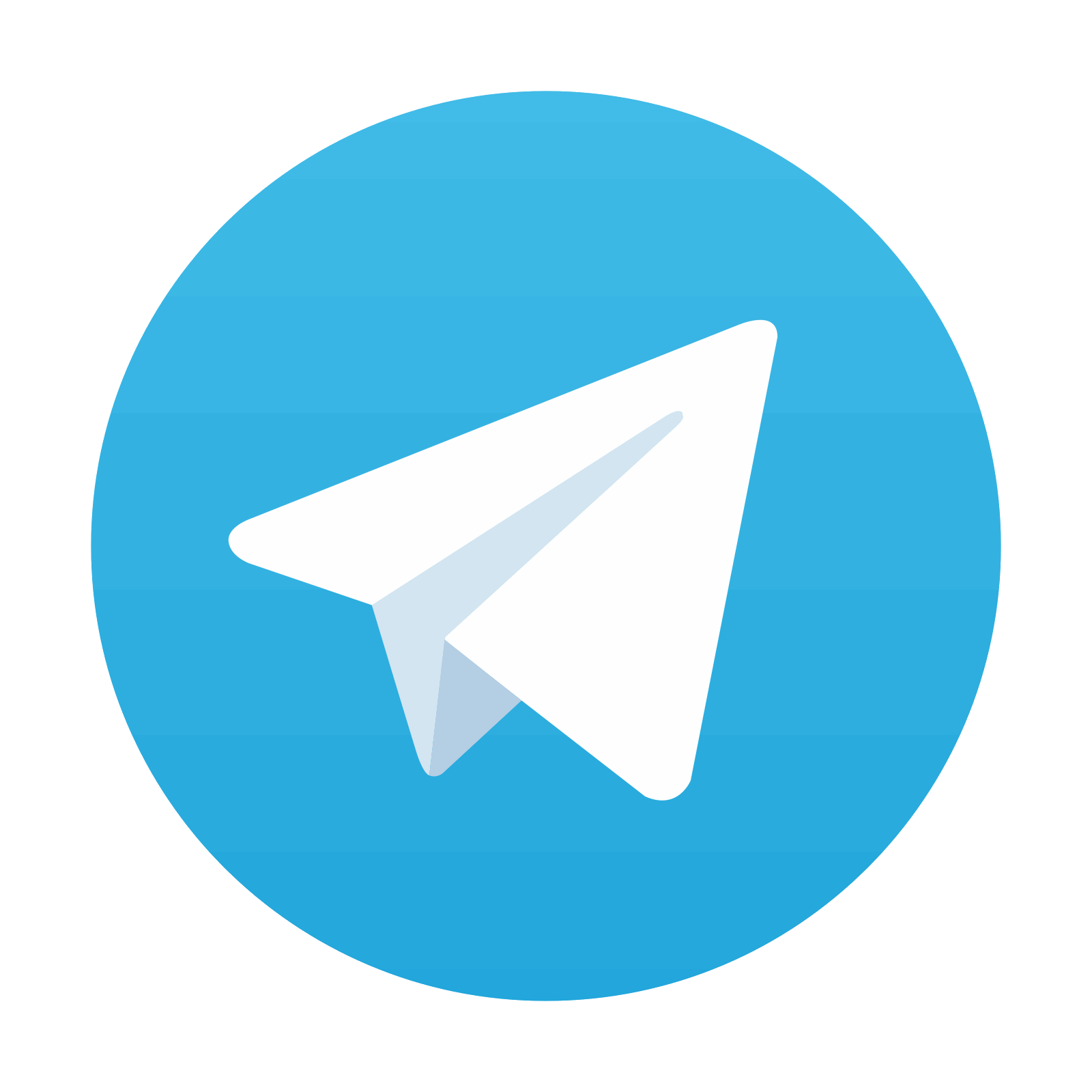
Stay updated, free articles. Join our Telegram channel

Full access? Get Clinical Tree
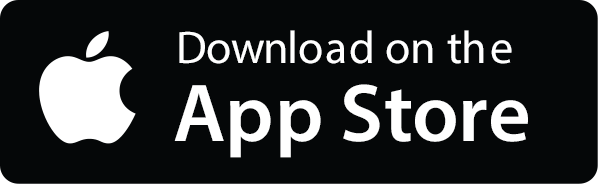
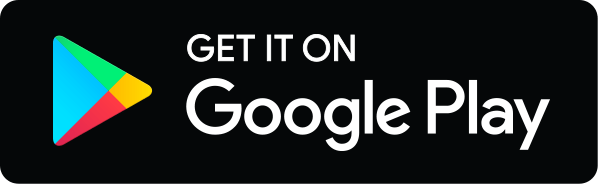
