Fig. 11.1
Increasing research interest in cognitive function and cancer
The incidence and duration of CRCI varies considerably from study to study and across diseases, with estimates that range from 0 % to nearly 70 % cancer patients being affected [10]. The actual incidence rate is difficult to identify due to the lack of sensitivity of many tests, the variable study designs used (e.g., cross-sectional or longitudinal, with or without a control group), a range of instruments used to measure cognitive functions, and the variety of definitions of what is determined to be an impairment on those scales [1]. In general, studies investigating CRCI have struggled with design and methodology issues that have limited progress. In addition to the known issues of confounding factors that can impact cognitive function, research in cancer is challenged with issues of sample size, defining an appropriate control group for comparison and defining an appropriate measurement tool or instrument [11].
11.2 Evidence
11.2.1 Tools and Instruments in CRCI
A number of meta-analyses and reviews [3, 12] have identified more than 30 tools and instruments assessing a variety of domains of cognitive function, demonstrating the disparate aspects of measurement of the overall concept of “cognitive function” and partially explaining why so many studies have found inconsistent results (Table 11.1).
Domain | Instrument measuring cognitive function | Patient-reported instrument |
General cognitive function | CLOX test High Sensitivity Cognitive Screen (HSCS) Exit 25 test HeadMinder Cognitive Stability Index Mini Mental State Exam (MMSE) Hopkins Verbal Learning Test-Revised (HVLT-R) Trails A and B test Finger tapping test Cognitive Drug Research Computerized Cognitive Assessment Digit Span Test Revised Rey-Osterrieth Complex Figure Test (ROCFT) Neurosensory Center Comprehensive Examination for Aphasia F-A-S subtest California Verbal Learning Test-II (CVLT) Cog State Weschler Memory Scale (WMS) Weschler Adult Intelligence Scale (WAIS) Cantab Cognition CogHealth | Patient assessment of Own Functioning (FAP) Cognitive Failures Questionnaire Questionnaire of Experienced Deficits of Attention Perceived Cognition Questionnaire Functional Assessment of Cancer Therapy – Cognition (FACT-Cog) Perceived Health Scale – Cognitive subscale |
Executive function | Fepsy Finger Tapping Grooved Pegboard Thumb Finger Sequencing Booklet Category Test HSCS self-regulation subtest Stroop Color and Word Test Trail Making Test (TMT)-Part B WAIS similarities subtest Controlled Word Association Test (COWA) WAIS-Revised/Similarities | EORTC-Cognitive item |
Memory | Rey Auditory Verbal Learning Test (RAVLT) TMT Rey Complex Figure Copy test (RCFT) RCFT recall; RCFT delayed recall WMS recall Visual reproduction Verbal memory subtest of the Barcelona test Benton Visual Retention Test California Visual Learning Test (CVLT) Verbal Selective Reminding Test (VSRT)-Delayed recall Nonverbal Selective Reminding Test (NVSRT)-Delayed recall Groeninger Intelligence Scale MMSE WAIS Weschler Memory Scale (WMS) visual reproduction subtest | |
Verbal function and language | WAIS-III C WMS logical memory subtest Boston Naming Test Word fluency test Luria Memory Words Test Controlled Oral Word Association Test (COWAT) HSCS language subtest CVLT HSCS memory subtest RAVLT | |
Construction | Block Design RCFT Copy | |
Concept and reasoning | Wisconsin Card Sorting Test Arithmetic test | |
Motor function | Grooved Pegboard Halstead-Reitan Neuropsychological Battery finger tapping subtest HSCS psychomotor subtest Fepsy Finger tapping Grip strength | |
Perception/recognition | Benton Faces Letter Cancelation CVLT RAVLT Hopkins Verbal Learning Test (HVLT) Rey 15-Item Test WMS verbal memory Repeatable Battery for the Assessment of Neuropsychological Status (RBANS) immediate memory VRST short term RBANS delayed memory WMS delayed recall Verbal Selective Reminding Test – delayed recall WMS visual memory Visual reproduction II Family pictures II ROCFT recognition Visual association | |
Processing speed | Fepsy Binary choice subtest Fepsy Visual reaction subtest Fepsy Visual searching subtest Paced Auditory Serial Addition Test TMT-Part A WAIS digit symbol subtest | |
Visuospatial skills | HSCS spatial subset RCFT copy WAIS-R block design subtest | |
Orientation/attention | HSCS attention subset WAIS digit span subset WAIS special span subtest WAIS-revised/arithmetic Intra-Extra Dimensional Set Shift Digit span test TMT Letter Number Sequencing Useful field of view test Reading Span Test Timed Instrumental Activities of Daily Living WAIS III – symbol search Stroop Digit Symbol Cognitive Performance Test D2 Test Fepsy Binary Choice Fepsy Visual Searching Fepsy Visual Reaction Paced Auditory Serial Addition Test Symbol search Spatial Span Attention CR/Attention RT TMT – B Wisconsin Card Sorting Test Tower of London Consonant Trigrams Boston Naming Test WAIS Wide Range Achievement Test-Reading RBANS language Block design RBANS visuospatial |
There is a lack of standardization across research trials, and there is no instrument designed specifically to measure CRCI. Instead, CRCI is measured by tools that have been developed for issues such as head trauma [13, 14], psychiatric issues [15], dementia [16], and other health concerns that are likely quite different from those experienced by cancer patients. Additionally, cognitive function may be measured by memory and attention in one study but measured as response time and verbal abilities in another, so the research reports quite different domains of the same overall construct, which may not all be affected in the same manner. The domains are also not measured uniformly across trials. Memory may be evaluated with the California Verbal Learning Test (CVLT) in one study but then may be evaluated using various subtests of the Weschler Memory Scale in another, making direct comparisons between trials difficult [12, 13]. In 2006, Vardy and colleagues demonstrated the variability in assessment of cognitive function using several instruments, including the CogHealth, HeadMinder, and the High Sensitivity Cognitive Screen (HSCS) tests, which found that 26 %, 55 %, and 30 % of patients in the same sample, respectively, experienced cognitive impairment simply depending on which instrument was used [17]. Other studies have demonstrated the lack of sensitivity of a variety of tests for the assessment of cancer-related cognitive function, despite those tests being sensitive, validated, and reproducible in the diseases or conditions for which they were developed [18, 19].
The International Cognition and Cancer Task Force was established in 2006 by several leading scientists in the field to address issues of assessment, study design and methodology, and the prevention and management of CRCI [20]. In 2011, this task force recommended for Hopkins Verbal Learning Test-Revised (HVLT-R), Trail Making Test (TMT), and the Benton Controlled Oral Word Association (COWA) of the Multilingual Aphasia Examination to be used to study CRCI. These instruments measure the cognitive domains of learning and memory, executive function, and processing speed and were selected as recommended instruments because they met the task force criteria of (1) being an objective measure; (2) measuring the domains of learning and memory, processing speed, or executive function; (3) having adequate sensitivity to measure the domain; (4) having adequate psychometric properties; and (5) being frequently used in the study of CRCI [20]. While harmonization is certainly needed, there remains a need for appropriate instrument development and instrument selection in research of CRCI, as none of the recommended instruments were developed for this purpose.
The HVLT-R was developed to measure abnormal forgetfulness in the domain of verbal memory [21]. The HVLT-R takes approximately 30 min to complete (5–10 min assessment + 25 min delay). It has been shown to be sensitive to dementia. The test is available in the public domain. In studies of lung cancer, the HVLT-R detected a decline in cognitive function among approximately 20 % of patients treated with prophylactic cranial irradiation [22]. Differences in cognitive function were found in a study of breast cancer patients receiving erythropoietin using the HSCS, but no significant differences were detected from the HVLT-R [23]. In a study of newly diagnosed glioblastoma, the HVLT-R detected a 60 % rate of cognitive impairment [24]. A recent meta-analysis of CRCI did not identify any studies that included the HVLT or HVLT-R [3]. The HVLT was also not identified in studies included in a meta-analysis evaluating the effect size of instruments to study cognitive function in cancer patients [18].
The TMT was developed in the mid-twentieth century to assess brain damage [25]. The respondent draws a line to connect a series of 25 circles as quickly as possible. In Part A of the test, the circles are numbered 1–25 and they must be connected in numeric order. In Part B, the circles contain letters (A–L) or numbers (1–13), which must be connected alternating from letter to number and both in ascending order. The score is based on the time needed to complete. It has shown to be sensitive to both dementia, cerebrovascular disease, and Alzheimer’s disease [26]. Despite its ability to detect subtle cognitive changes, the TMT was found to have a small effect size in a meta-analysis of tools evaluating cognitive function in breast cancer patients [18]. In a study of colon cancer patients, the TMT detected no significant change over time (pre-chemotherapy through the post-chemotherapy or 6-month follow-up assessments) [27]. A longitudinal study of breast cancer patients found no significant differences using the TMT, but they did find significant differences using the HSCS when comparing cancer patients to controls [28]. A second study of breast cancer patients found no differences with the TMT-Part A, but that participants performed significantly worse before chemotherapy than during treatment on the TMT-Part B [29]. Other breast cancer studies have found no differences over time pre- to post-chemotherapy [30]. The TMT was also used to evaluate cognitive change over time in an ovarian cancer patient population; stable or improved scores (not significantly different) were found from baseline to the treatment period or following chemotherapy [31]. In a study evaluating cytokine levels of patients with leukemia, the TMT-Part B performance was found to be lower among those with higher levels of circulating IL-6 [32].
The COWA (also termed the FAS test in some studies, although the Benton set is most commonly used) is a verbal fluency test within the Multilingual Aphasia Examination that can be administered within 5–10 min [33]. The administrator provides a letter of the alphabet, and the respondent is to provide as many words as possible that begin with that letter within a one-minute time period [34]. It is scored by counting the number of words identified by the respondent. It was originally developed for patients with focal brain lesions and has been found to discriminate in studies of attention deficit and hyperactivity disorder, attention deficit disorder, amnesia, Huntington’s disease, children with learning disabilities, and adults and children with head injuries [33]. In cancer research, one study found no differences in COWA test results with glioblastoma patients versus non-glioblastoma controls [35]. A study comparing pre-chemotherapy to short- and long-term follow-up time points among women receiving adjuvant treatment for breast cancer found no significant differences over time using the COWA, but significant differences were found using the Block Design, Booklet Category Test, Digit Symbol Test, Verbal Selective Reminding Test Long-Term Storage, and Nonverbal Selective Reminding Test Long-Term Storage at the short- but not long-term follow-up [30]. In a study comparing breast cancer patients to controls, COWA scores were significantly different, but the effect sizes were small [36]. Another longitudinal study in breast cancer demonstrated 5 % of patients experience cognitive decline over time using the COWA [37].
While each of the tests recommended by the Task Force met their stated criteria, none were developed specifically for the concerns of cancer patients. The selection of an appropriate measurement tool in any study must further be based on factors such as its ability to distinguish between those who are affected by CRCI and those who are not, the sensitivity of the test to detect CRCI, and the availability of parallel forms for prospective or longitudinal studies [18]. While the Task Force guidelines are an important first step to standardize the field and to produce criteria that may reduce the use of tests with little sensitivity that has troubled prior work, scientists must still thoughtfully select the instrument most appropriate to the study question and population of interest.
Patient self-report measures are useful for the impact of function on patient perspectives but cannot directly measure the cognitive function of individuals. This does not negate their importance in any way, as the most important aspect of toxicity to understand is the impact on individual patients, their quality of life, and functional abilities. Unlike the objective instruments discussed above, there are self-reported measures for cognitive function that were developed specifically for the cancer patient (e.g., EORTC-QLC C30 cognitive subscale and the FACT-Cog). The EORTC cognitive subscale contains only two items for cognitive function (memory and concentration, respectively) in the cognitive domain [38], limiting its use for studies focused on cognitive function. It has also been shown to perform poorly as a stand-alone subscale [39]. The development and psychometric properties of the FACT-Cog are available [40], and work has been published on the translation of the FACT-Cog for use in non-American populations [17, 41]. A new version of the scale is in development but has yet to be published.
Thus, despite the increased interest and recognition of this issue, there currently is no standard approach to measure cognitive function in the cancer patient receiving chemotherapy. The underlying problem is still poorly understood, and no validated tools have been developed to measure the cognitive changes that occur specifically in cancer populations.
Despite the lack of validated instruments to measure CRCI and the variability in outcomes across studies, many studies are able to identify a subset of patients who experience cognitive decline in various domains. Individual studies report incidence rates of CRCI ranging from 0 % to nearly 70 % [10]. Meta-analyses of cognitive function in the cancer patient have tried to better understand this concern by pooling results across cognitive domains.
A meta-analysis of 13 studies that were published through 2010 found that executive function, memory, verbal function, and language skills were significantly impacted but that all had very small effect sizes [3]. Neither patient age nor time since treatment discontinuation was a contributing factor to these declines. This study was limited to studies comparing patients receiving chemotherapy compared with a control group, patients over the age of 21, and studies that provided calculated effect sizes and that had a primary aim of studying cognitive function. The eligible studies were primarily breast cancer trials, but leukemia, testicular cancer, and lymphoma studies were also included in the analysis.
A larger meta-analysis was conducted of 44 studies that were published through January 2011 [10]. This meta-analysis included more studies due to broader eligibility criteria. All studies of chemotherapy and cognitive function that had a control group and reported a mean value of the cognitive assessment with either a cross-sectional or longitudinal design were included. This study computed a Hedge’s g for each of the studies; nearly three quarters of the eligible studies were from breast cancer populations. This meta-analysis found few statistically significant effects (visual memory and visual recall significantly improved and only selective attention declined versus controls), and the effects were of small size. This could be largely due to the heterogeneity of studies washing out any potential effect seen in a more selective subgroup of studies. In a subset analysis of cross-sectional trials, there were more impairments identified versus controls (significant differences found for the domains of memory, immediate free recall, delayed memory, delayed recognition, verbal memory, verbal immediate free recall, verbal delayed free recall, verbal delayed recognition, selective attention, and capacity of attention) [10]. When limited to longitudinal studies, improvements in cognitive function after chemotherapy were identified for many of the domains studied (i.e., immediate free recall, verbal immediate free recall, visual immediate free recall, visual delayed memory, focused attention, capacity of attention, and verbal abilities all significantly improved over time).
An additional meta-analysis was conducted of 17 studies in breast cancer published through June 2011 that found significant declines in verbal ability and visuospatial ability for breast cancer patients receiving chemotherapy compared to controls, but the effect sizes were small [42]. These declines were also not moderated by a number of factors that had been suspected of influencing cognitive function, such as age, time since treatment, education, or endocrine therapy. Thus, even across recent meta-analyses for similar time periods, the results remain inconsistent and additional research is needed.
11.2.2 Neuroimaging in CRCI
In recent years, researchers have shown greater interest to utilize novel neuroimaging technology to define structural, functional, and molecular changes to understand patterns of brain abnormalities associated with CRCI in breast cancer survivors. These studies use neuroimaging techniques combine with detailed cognitive assessment to evaluate the changes associated with chemotherapy. Quite a few of studies showed evidence of alterations on neuroimaging that may explain the cognitive impairment in patients exposed to chemotherapy [43–65].
Several structural magnetic resonance imaging (MRI) studies have utilized voxel-based morphometry (VBM) to assess regional abnormalities in the brain associated with chemotherapy [43, 44, 46–51]. Vertebral Motion Analysis (VMA) is a fully automated structural neuroimaging technique used for quantitatively evaluation of the brain to detect the differences in regional volume and density. A prospective, longitudinal study of 44 patients included 17 breast cancer patients treated with systemic chemotherapy, 12 breast cancer patients without chemotherapy and 18 healthy controls. At baseline, no between-group structural brain differences were observed prior to initiation of systemic chemotherapy. One month following chemotherapy, patients had diffuse loss of gray matter volume and density. At 1-year follow-up, a partial gray matter recovery was observed with alterations persisting predominantly in frontal and temporal regions. This trend was not observed in patients who received antiestrogen treatment without systemic chemotherapy and healthy control.
Other prospective VBM analyses [43, 46] also showed diffuse loss of gray matter volume and density shortly after systemic chemotherapy. These gray matter alterations partially recover overtime after chemotherapy. At 1 year, close to half of the regions with gray matter volume loss had resolved [43]. This phenomenon correlates with the severity of cognitive impairment in cancer patients, worst during or soon after chemotherapy and gradual improvement in cognitive function with partial recovery overtime [43, 44]. A study found that gray matter density was directly related to cognitive performance in the domains of processing speed, working memory, and visual memory [48]. The recovery from chemotherapy overtime was partial and not a complete return to baseline. Long-term irreversible abnormalities related to chemotherapy persistent in the gray matter distributed in frontotemporal regions [43, 44, 49]. Moreover, studies have demonstrated residual gray matter deficits in the chemotherapy-treated breast cancer patients, even beyond a decade after adjuvant therapy completion [48–50]. The total reduction of gray matter volume associated with chemotherapy was comparable to almost 4 years of age-related decline in gray matter volume [48]. These findings may provide evidence to explain the subtle but clinically relevant residual cognitive deficit in a subset of breast cancer survivors.
Diffusion tensor MRI (DTI) is capable of detecting subtle changes in the microstructure of white matter fiber tracts. DTI has the sensitivity required to quantify microstructural changes in white matter integrity related to CRCI in the breast cancer population. DTI measures the diffusion of water molecules along fiber tracts, reflecting white matter local microstructure and anatomy. The main parameters are fractional anisotropy (FA) reflecting the directionality of diffusion and mean diffusivity (MD) describing the amount of diffusion within a voxel. Intact white matter tissue is thought to be characterized by high FA and low MD [66].
A cross-sectional, pilot study [55] investigated the effect of chemotherapy on white matter in the genu of the corpus callosum in breast cancer patients compared to healthy controls. Compared to controls, patients treated with chemotherapy had both slower processing speed and lower FA in the corpus callosum. In this region-of-interest analysis, only corpus callosum was selected for DTI parameters of white matter integrity. Subsequently, whole-brain DTI studies showed similar evidence that chemotherapy was associated with abnormal microstructure in white matter indicating loss of integrity. Furthermore, a relationship was identified between white matter alteration and cognitive decline in chemotherapy-treated breast cancer patients [49, 50, 55–57]. The quantitative FA changes in frontal, temporal, and parietal white matter tracts, including the superior and inferior longitudinal fasciculus, directly correlated with neuropsychological test scores in the cognitive domains of memory, attention, and processing speed. The subjective self-reported cognitive complaints also correlated with FA changes in frontal and parietal white matter regions. Moreover, breast cancer patients with CRCI showed a greater degree of white matter injury [56, 57]. Long-term changes in white matter microstructure were also observed in breast cancer patients 10 years after high-dose chemotherapy. In the chemotherapy group, observed DTI abnormalities were localized close to regions with reduced gray matter volume and density [49, 50].
A longitudinal whole-brain study [56] compared DTI measures in white matter taken before and 3–4 months after chemotherapy with detailed cognitive assessment. This study also included breast cancer patients not exposed to chemotherapy and healthy controls which underwent the same assessment at matched intervals. No baseline differences between the three groups were observed in FA values or cognitive testing score. After chemotherapy, both FA values and cognitive test scores decreased compared to baseline. No changes were observed in either control groups at same time interval. These findings suggest that the longitudinal changes in white matter microstructure are specific to chemotherapy exposure and not related to cancer or antiestrogen therapy. A larger cross-sectional study [54] in breast cancer survivors (average >20 years) found a decline in white matter microstructural integrity with longer time since chemotherapy.
In CRCI research, very little evidence has been reported on differences in specific chemotherapy, chemotherapy regimen, or the relationship between dose and cognitive outcomes. A study compared high-dose versus conventional-dose chemotherapy regimen in addition to radiation only and healthy control [50]. A lower global white matter integrity and lower cognitive test score was found in the high-dose chemotherapy group compared to the conventional-dose group. In the conventional-dose chemotherapy group, a focal loss of white matter integrity was found predominantly in the frontal brain region [50, 57]. These findings suggest an association between high-dose chemotherapy and worse cognitive functioning, long-term lower local gray matter volume, lower white matter integrity, and lower axonal function, whereas the effects of conventional-dose chemotherapy were less pronounced and less widespread. This suggests that even ≥10-year post-high dose treatment, patients may experience worse cognitive and structural brain outcomes than those treated with conventional-dose chemotherapy [50].
Functional MRI (fMRI) and positron emission tomography (PET) studies are functional neuroimaging techniques that may contribute to detect differences in brain function even without a clear structural damage. PET records the distribution of radioactive tracers to assess brain metabolism, whereas fMRI relies on differences in the magnetic properties of oxygenated and deoxygenated hemoglobin varying with the metabolic demands of the brain tissue. Functional studies measure areas of brain activation during selected tasks to target cognitive domains previously identified in neuropsychological assessment [67, 68]. In functional brain research, fMRI has become the preferred method in recent years in view of its higher resolution and the absence of radioactive exposure [68].
In a functional imaging study [60], PET showed increased activation of left inferior frontal cortex, during verbal memory task, in the chemotherapy-treated group compared to the not-treated breast cancer survivors. This increased activation during the verbal memory task was interpreted by the study team as compensatory response to overcome the underlying chemotherapy-induced deficit [60]. A case study [61] utilized fMRI to investigate a monozygotic twin (chemotherapy-treated twin compared to the healthy, untreated twin). The chemotherapy-treated twin had substantially more self-reported cognitive complaints. Although the task performance scores were similar, the chemotherapy treated had broader task activation of bilateral frontal and parietal regions compared to the untreated. Again, the authors interpret this pattern of increased activation as evidence of compensatory strategy (increase in effort to recruit more circuitry) to maintain performance.
More recently, fMRI studies reported significant decrease in brain activation during multitasking in the chemotherapy-treated breast cancer patients [47, 49, 52, 58, 59]. Specific fMRI findings include task-specific decreased activation in the prefrontal and parietal regions during tasks of executive function [47, 49, 52, 58, 59], and decreased activation in the parahippocampal gyrus was observed with a memory-encoding paradigm and generalized decreased activation of lateral posterior parietal cortex consistent with decreased attention processing [49, 59]. Interestingly, chemotherapy-induced loss in gray matter density [47, 49, 53, 59] and/or white matter microstructural [49] overlapped with some of the brain regions with altered pattern of activation on fMRI. Therefore, the significant decrease in brain activation during multitasking may be associated with chemotherapy-induced decline in quality of brain tissue. In neurocognitive assessments, chemotherapy-treated patients performed significantly worse than the control group(s) [47, 49, 53, 58, 59]. The subjective self-reported cognitive complaints also correlated significantly to decreases in multitasking-related brain activation [52, 58]. Conversely, an increased activation of the dorsolateral region of the prefrontal cortex was associated with improved task performance in chemotherapy-treated patients [59]. These findings were interpreted as specific regional impairment in brain function related to CRCI.
Several fMRI studies reported increase in bilateral frontoparietal brain activation in breast cancer patients not (yet) treated with chemotherapy compared to healthy control [53, 62, 64], which raised the concern about possible early deficiency in brain function in breast cancer associated with other variables (e.g., fatigue, other treatment(s), response to illness). These functional alterations in frontoparietal region resemble the findings associated with normal aging that is evident in older adults [64]. The authors interpreted the increase brain activation as recruitment of additional circuitry to compensate the functional insufficiency [53, 62, 64] possibly due to fatigue [64]. A prospective study in resting-state fMRI of 65 patients reported greater fatigue in pre-chemotherapy group compared to radiation therapy-only and health control group. The pre-chemotherapy group showed greater frontoparietal executive network variance on fMRI than the radiation therapy-only group. The authors interpreted this greater frontoparietal variance in the executive network as a predictor of neural inefficiency that is more likely to lead to future CRCI [65]. Another study [63] on resting-state fMRI displayed altered network organization in frontal, striatal, and temporal areas in chemotherapy-exposed breast cancer patients compared to healthy controls. The breast cancer group had greater self-reported complaints of decline in executive function and memory. These alterations in resting-state network properties suggest a decrease in processing efficiency. The functional inefficiency may lead to greater effort and perceived challenge for everyday cognitive demands. The authors note that several of the specific alterations observed in the study resemble findings reported in association with normal aging [63].
More complex findings were reported in a prospective fMRI evaluation of breast cancer patients at matched intervals (at baseline/pre-chemotherapy, 1 month and 1 year post-chemotherapy) [53]. At baseline, breast cancer patients (not treated and not yet treated with chemotherapy) had increased activation in frontal region bilaterally compared to healthy controls. At 1 month after chemotherapy, a decrease activation was observed in the previously mentioned area of activation. At 1 year after chemotherapy, increased activation to baseline level was observed in the frontal brain regions, accompanied by overlapping of a persistent gray matter volume decrease [53]. These findings were interpreted by some authors as compensatory activation, but early cognitive compromise specifically related to cancer could not be excluded. In contrast, a similar longitudinal fMRI study [52] did not find a pre-chemotherapy difference in task-related brain activation among the three groups. Moreover, no structural differences in gray matter volume [46] or white matter microstructure [56] at baseline were reported in the prior VMA or DTI studies; therefore, the observed pre-chemotherapy increase in brain activation is more likely related to cognitive strategies (compensatory activation) rather than a reflection of brain pathology [52].
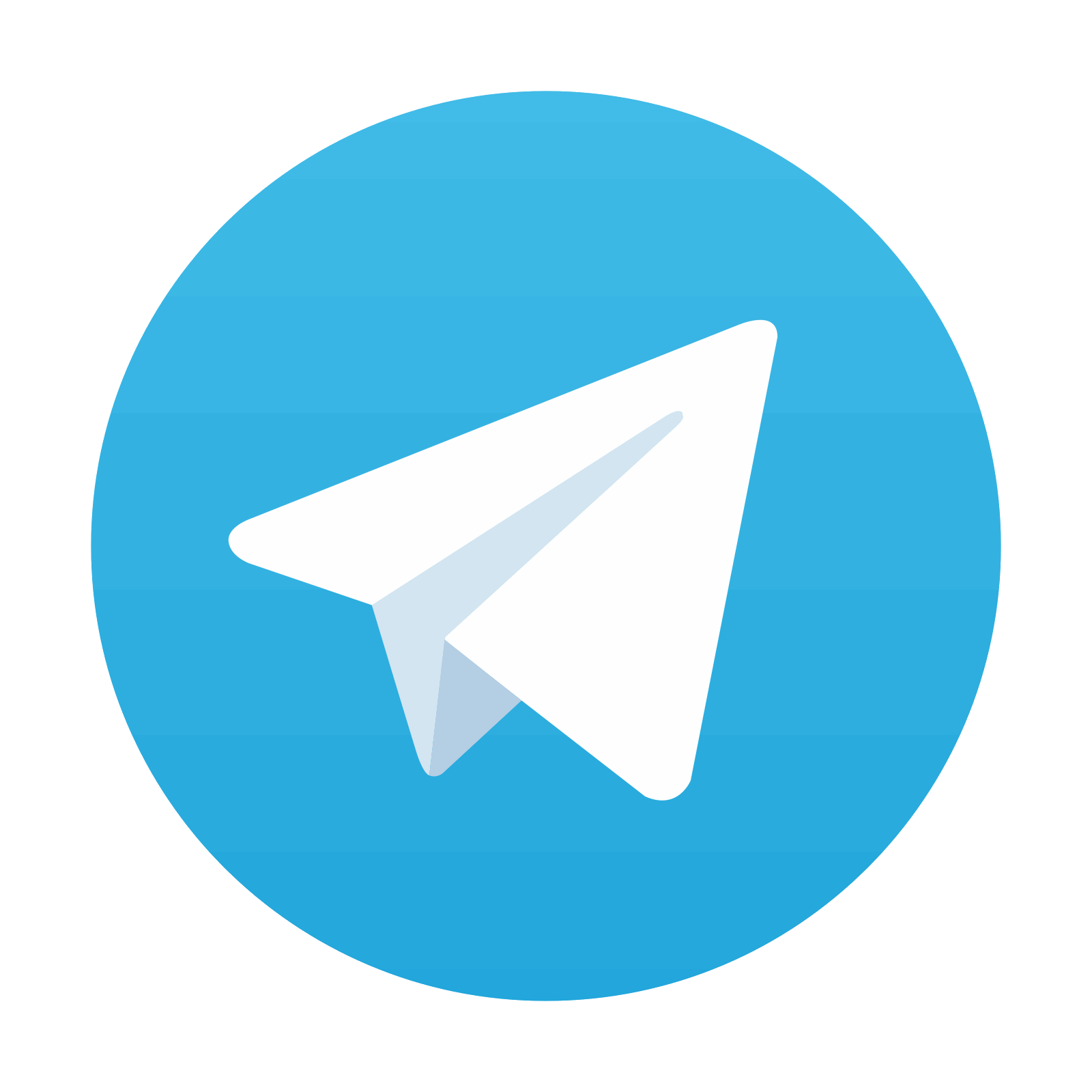
Stay updated, free articles. Join our Telegram channel

Full access? Get Clinical Tree
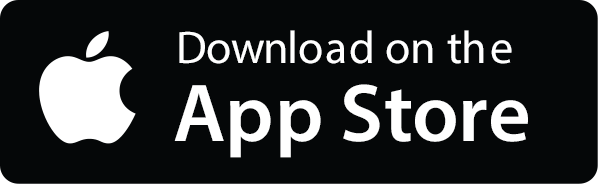
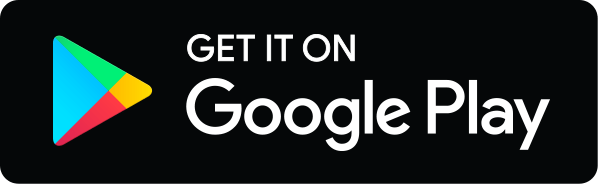