Fig. 15.1
Immature teratoma arising from the suprasellar area in a 13-year-old boy. (a) CT scan shows a mass in the third ventricle with low attenuation. Minute dots of high attenuation are observed in the mass, indicating the presence of calcification. (b) Areas of high signal intensity are found in the tumor in a T1-weighted image which represent fatty tissues in the tumor. (c) The tumor is well enhanced in gadolinium-enhanced T1-weighted image
15.5 Workup
Accurate diagnosis and staging is required to guide therapy. Diagnosis is based on the combination of clinical symptoms, tumor markers, neuroimaging characteristics as well as cytological and histological confirmation. CT scan and/or magnetic resonance imaging (MRI) scan of the brain and spine with Gd-DTPA should be performed. Leptomeningeal spread is not uncommon at diagnosis (Aoyama 2009; Calaminus et al. 2005). Many studies with large patient numbers include both germinoma and NGGCT (Matsutani et al. 1997; Sawamura et al. 1998; Aoyama et al. 1998; Sano et al. 1989), and the exact rate of leptomeningeal spread specifically for NGGCT is not readily available in these studies. In the studies with relatively small number of patients, screening for detection of leptomeningeal disease is inconsistently performed (Kim et al. 2012; Ogawa et al. 2003; Robertson et al. 2014). According to a recent report which used modern MRI imaging for spinal leptomeningeal spread, it is reported to be as high as 22% (Korean Society of Pediatric Neuro-Oncology group study, unpublished data). Lumbar puncture for examination of human chorionic gonadotropin-beta (β-hCG) and α-fetoprotein (AFP) should be performed along with cerebrospinal fluid (CSF) cytology. Examination of serum AFP and β-hCG are also required. CSF level of tumor markers are more sensitive and reliable for diagnosis than serum level. Due to the common presentation of endocrinopathies, a thorough endocrine evaluation at the time of diagnosis is highly recommended (Thakkar et al. 2013). Imaging is not reliable to distinguish germinomas from NGGCTs, and diagnosis is made either by tissue confirmation or tumor markers. Tumor markers can be more sensitive than changes in images (Jorsal and Rorth 2012) and can be used during treatment to assess tumor response. It is highly controversial whether a tissue biopsy is mandatory for ICGCT because many patients with ICGCT can be correctly diagnosed without a tissue biopsy if demographic and radiological data were combined with serum/CSF tumor markers. In the recent COG study, patients with elevation of serum or CSF β-hCG >100 IU/L or any elevation of serum and CSF AFP >10 ng/mL are considered as NGGCT. The SIOP CNS germ cell tumor study uses >50 IU/L and >25 ng/mL as cut-off values for β-hCG and AFP, respectively, however, a biopsy is regarded as mandatory if marker levels are ≤50 IU/L (total HCG) or ≤25 ng/mL (AFP) in order to exclude the possibility of any malignant component in tumors (https://www.skion.nl/workspace/uploads/2_siop_cns_gct_ii_final_version_2_15062011_unterschrift_hoppenheit.pdf). There are pitfalls in diagnosing NGGCT on the basis of elevated tumor markers without a histologic diagnosis. Using an ultrasensitive enzyme immunoassay (EIA) method or a quantitative measurement of β-hCG mRNA in tumor tissues (Takami et al. 2015), Japanese investigators showed that some pure germinomas and at least some NGGCTs produce β-hCG. In that study, germinomas with syncytiotrophoblastic giant cells and those located other than at midline structures of the brain were associated with higher β-hCG levels. There are occasional patients with biopsy-proven germinoma who initially presented with mild elevation of AFP below reference level and recur with a mixed malignant component. Patients with mildly elevated AFP need to be carefully watched particularly if biopsy was not performed at the time of diagnosis. More emphasis is now being given in taking tissue biopsy before treatment starts. More standardized cut-off value needs to be adopted for diagnosis of NGGCT especially with serum and CSF β-hCG. When treatment is completed, routine physical and neurologic examinations are carried out every 3–6 months after treatment. Follow-up MRI is generally carried out every 6 months for the initial 5 years after treatment or more frequently for tumors which do not show complete response to treatment, and then once a year.
15.6 Acute Management
Obstructive hydrocephalus is frequently observed in the patient with ICGCT. Diagnosis of accompanying hydrocephalus and its management is the initial step in the treatment of patients with ICGCT. Intravenous mannitol can alleviate symptoms of increased intracranial pressure (ICP). Although corticosteroids are commonly used for the management of brain edema associated with tumors, they are less helpful for ICGCT because brain edema is less prominent for intraventricular tumors than for parenchymal tumors. If obstruction of the CSF pathway is evident in MRI and/or CT, surgical diversion of the CSF is required after initiation of mannitol and corticosteroid administration. For tumors located in the pineal area, endoscopic third ventriculostomy (ETV) is the treatment of choice to relieve the increased ICP. ETV can be performed simultaneously with an endoscopic tumor biopsy. For suprasellar tumors, ETV is rarely attempted because the tumor mass blocks the third ventricular floor. If a large suprasellar GCT occludes both foramina of Monro and causes severe hydrocephalus, an endoscopic septostomy is recommended with tumor biopsy. The procedure should be followed by a temporary extraventricular drainage (EVD) if surgical resection is planned (as is common for NGGCTs), or by a permanent ventriculoperitoneal shunt if chemotherapy or radiation therapy is scheduled (as is common for germinomas) for definite treatment of the tumor. If only one foramen of Monro is occluded, an endoscopic septostomy with tumor biopsy may be sufficient.
15.7 Treatment
15.7.1 Chemotherapy
Systemic chemotherapy is an essential part of the multimodal treatment of NGGCT. The use of neoadjuvant chemotherapy with radiation therapy results in a very high rate of response with acceptable toxicity and excellent outcomes. Survival rate of patients with NGGCT increased to 60–70% by use of combined chemoradiotherapy (Kim et al. 2012; Robertson et al. 1997, 2014; Goldman et al. 2015) compared with 20–40% when only RT (Dearnaley et al. 1990; Hoffman et al. 1991) or only chemotherapy (Balmaceda et al. 1996; Baranzelli et al. 1998; Chang et al. 1995) was given . Most of the patients are treated with cisplatin or carboplatin in combination with other agents. Cisplatin and etoposide (PE), carboplatin and etoposide (CARB-VP) combination treatment are commonly used. Ifosfamide has been added to PE or CARB-VP for patients at higher risk for recurrence (Calaminus et al. 2005; Robertson et al. 2014; Goldman et al. 2015). Vincristine and cyclophosphamide also have been used (Thakkar et al. 2013; Ogawa et al. 2004). For patients with an immature teratoma component (Japanese intermediate prognosis group, Table 15.1), an approach which includes surgical resection, radiotherapy, and chemotherapy leads to the best treatment outcome (Matsutani et al. 1997; Sawamura et al. 1998). Patients with a serum AFP level >1000 ng/mL and age <6 years are categorized as high risk group in the phase II Society of International Pediatric Oncology (SIOP) CNS germ cell tumor trial, and high dose chemotherapy with peripheral stem cell transplant is attempted for this subset of patients and who show residual unresectable tumors after three cycles of chemotherapy. The current COG study (ACNS1123) also tests whether dose escalation of chemotherapy would increase event-free survival in patients with higher risk for recurrences based on serum/CSF AFP using high dose cisplatin, etoposide, ifosfamide (PEI), and peripheral stem cell transplant (Calaminus et al. 1997, 2012). The current study of the Japanese CNS germ cell tumor study group adopts concomitant radiochemotherapy for NGGCT patients with histologic diagnosis of choriocarcinoma, embryonal carcinoma, yolk sac tumors, or tumors with mixed histology. Adjuvant chemotherapy is also given after completion of radiotherapy (personal communication, Masao Matsutani, Saitama International Medical Center, Hidaka, Japan).
Table 15.1
Classification of intracranial germ cell tumors by prognosis (Matsutani classification) (Matsutani et al. 1997)
Good prognosis |
Pure germinoma |
Mature teratoma |
Intermediate prognosis |
Germinoma with syncytiotrophoblastic giant cells |
Immature teratoma |
Teratoma with malignant transformation |
Mixed tumors mainly composed of germinoma or teratoma |
Poor prognosis |
Choriocarcinoma |
Yolk sac tumor |
Embryonal carcinoma |
Mixed tumors mainly composed of choriocarcinoma, yolk sac tumor, or embryonal carcinoma |
15.7.2 Radiotherapy
15.7.2.1 Radiotherapy Volume and Dose
NGGCT is less radiosensitive than germinoma. Radiation doses higher than 50 Gy given to the primary tumor site is associated with superior survival rates (Matsutani et al. 1997; Kim et al. 2012; Sawamura et al. 1998; Aoyama et al. 1998; Schild et al. 1996) than those with less than 50 Gy. Regarding the volume of radiation therapy, the studies using craniospinal irradiation (CSI) tend to give the best survival outcome; however, it has not yet been determined whether CSI should be used in all patients with NGGCT. In the SIOP phase II trial, focal radiotherapy was adopted on the basis of the results of their preceding trials (Calaminus et al. 1997, 2012), which showed that focal irradiation was sufficient for local tumor control in localized disease if >50 Gy was delivered. All SIOP patients with localized NGGCT receive focal radiotherapy, and 30 Gy CSI is given only to the patients >6 years old with disseminated disease (multiple masses and/or positive cytology) with 24 Gy boost to the gross tumor. In the SIOP study, patients in the high risk group (serum AFP level >1000 ng/mL and age <6 years) are treated with focal radiotherapy, whereas the Japanese CNS germ cell tumor study group uses 30.6 Gy CSI with a 30.6 Gy tumor bed boost for high risk group (AFP level >2000 ng/mL and/ or a serum β-HCG level >2000 IU/L). In the Japanese protocol, the rest of the patients with localized tumors receive whole ventricular irradiation (WVI). The COG ACNS1123 study is currently underway to test whether the dose and volume of radiotherapy can be adapted according to the tumor response to six cycles of carboplatin/etoposide alternating with ifosfamide/etoposide chemotherapy. In this protocol, CSI is avoided when the response to chemotherapy is good. The patients are given 30.6 Gy of WVI with a boost dose of 23.4 Gy to the primary site if the patients showed complete response (CR) to chemotherapy or showed partial response (PR) with normalization of tumor markers. The patients who showed less than PR with negative tumor markers undergo second-look surgery. If second-look surgery converts the patient to CR or PR, then the patients get 30.6 Gy WVI followed by a 23.4 Gy primary site boost.
There are studies which support the use of CSI in intracranial NGGCT. The results of a phase II multi-institutional study which used WVI for patients with localized disease and for patients who showed CR to four cycles of neoadjuvant chemotherapy showed that there was a relatively high frequency (25%) of relapse outside the initial RT volume despite the use of high dose chemotherapy and second-look surgical resection (Robertson et al. 2014). In a multi-institutional study by Aoyama et al. (1998), spinal canal recurrence was observed in 37.8% (3/8) of patients in the poor prognosis group who did not have CSI. None of the 5 patients in the same group who received CSI experienced spinal recurrence. Matsutani et al. (1997) also reported a 21.7% (5/23) rate of spinal recurrence in the poor prognosis group patients treated primarily by whole brain radiotherapy without spinal irradiation.
15.7.2.2 Radiotherapy Technique
Adoption of radiotherapy techniques which can minimize radiation doses to the normal tissue is important in treatment of patients with NGGCT. Comparison of the dose–volume histograms (DVH) of intensity-modulated radiotherapy (IMRT) and three dimensional-conformal radiotherapy (3D-CRT) for WVI showed that IMRT reduced the irradiated volume receiving radiation doses of 20, 30, and 40 Gy by 7.5%, 12.2%, and 9.0%, respectively, compared with 3D-CRT (Chen et al. 2010). Proton beam therapy (PBT) can further reduce radiation dose to the normal tissue because of its physical characteristics with the absence of dose beyond target tissue. The use of PBT for CSI also significantly reduces hematological toxicity (Barney et al. 2014; Brown et al. 2013; Song et al. 2014) as well as decreases the risk of late effects such as secondary malignancy (Miralbell et al. 2002; Yoon et al. 2011). In addition, using PBT for WVI brought the volume of the cerebral cortex receiving ≤10 and ≤15 Gy down to two-third to a half of the dose achieved with 3D-CRT and IMRT, respectively (Fig. 15.2) (Kim and Park 2015). In tumors arising in the pineal gland, suprasellar area, or basal ganglia, PBT was shown to deliver lower mean radiation doses to the cochlea, pituitary gland, and temporal lobes (Fig. 15.3) (Park et al. 2015). PBT significantly differs from photon therapy in the volume of brain parenchyma that receives doses of 5–20 Gy, and the volume of temporal lobes that receives doses of 5–30 Gy when a total of 30.6 Gy was delivered to the primary tumor (Park et al. 2015). Whether this extent of dose reduction would translate into better neurocognitive function remains to be seen.
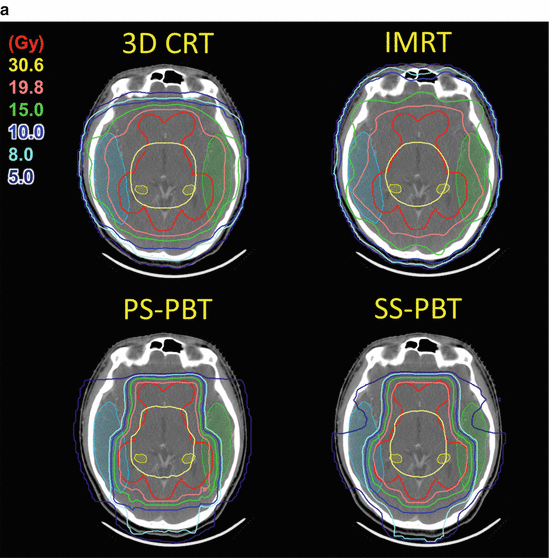
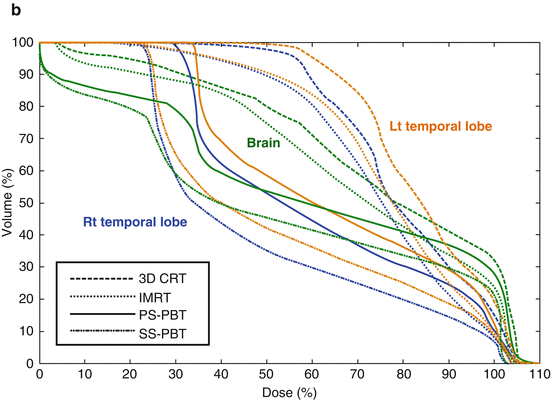
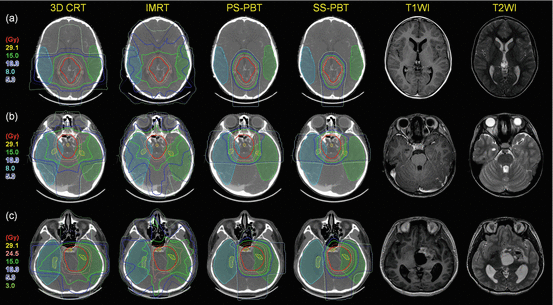
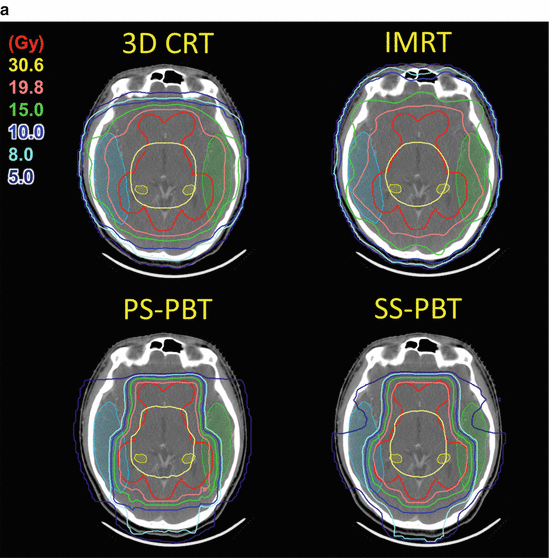
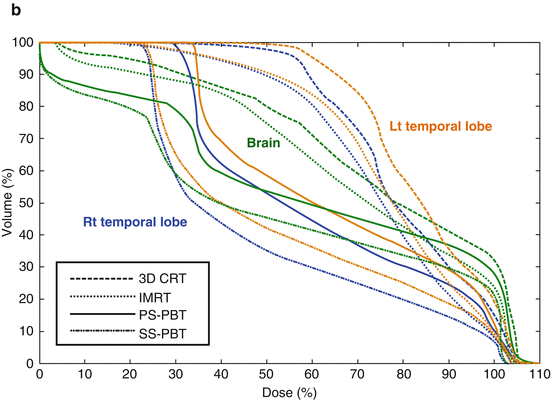
Fig. 15.2
(a) Dose distribution for whole ventricle irradiation. Comparison of 3D-conformal radiotherapy (3D-CRT), intensity-modulated radiotherapy (IMRT), passive scattering proton beam therapy (PS-PBT), and spot scanning proton beam therapy (SS-PBT). Thick red lines and thick yellow lines are combined planning target volume (PTV) for the whole ventricle and tumor bed boost for pineal tumor. Blue, green, pink, and yellow lines represent 10, 15, 19.8, and 30.6 Gy isodose lines, respectively. Both temporal lobes and hippocampi are contoured. (b) Dose volume histogram (DVH) of normal brain and both temporal lobes in the whole ventricular irradiation including the pineal gland tumor bed. Prescribed dose are 19.8 Gy at 95% volume of whole ventricular PTV and 10.8 Gy boost to the pineal gland boost PTV (Calaminus et al. 2012)
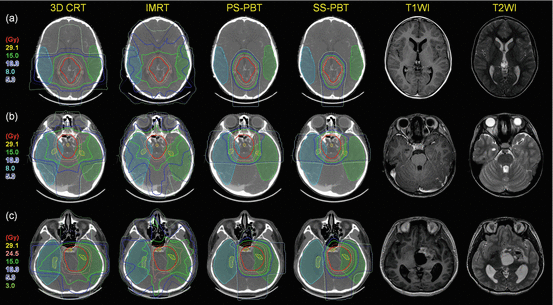
Fig. 15.3
Dose distributions of primary site boost irradiation to pineal gland, suprasellar, and basal ganglia tumors with their pre-chemotherapy MRI images. Thick red lines are primary site PTV. Thick blue lines represent 10 Gy, and green lines represent 15 Gy isodose line. Prescribed dose is 30.6 Gy at 95% volume of PTV. Tumors arising in the (a) pineal gland (b) suprasellar area (c) left basal ganglia (Calaminus et al. 2012)
15.7.3 Surgery
The roles of surgery in the management of NGGCTs are as follows: (1) management of hydrocephalus, (2) tissue biopsy, (3) primary resection, (4) delayed (second-look) resection. Endoscopic tumor biopsy is the most commonly applied procedure because of the versatility and safety. Bleeding from the tumor during the procedure is a potential complication. Tumor bleeding can be managed successfully with water tamponade, direct coagulation, and EVD. Open craniotomy for bleeding control is rarely required. Stereotactic biopsy is reserved for deep-seated NGGCTs in the basal ganglia or brainstem. The management of hydrocephalus is discussed above. Even when a radical surgical resection is planned for NGGCTs, management of hydrocephalus should be considered first.
Primary surgical resection is the only treatment option for mature teratoma. After complete resection, the prognosis of mature teratoma is excellent. However, for NGGCTs other than mature teratoma, the situation is more complex because these malignant tumors can rarely be cured by surgery alone. Currently, the majority of treatment protocols adopt neoadjuvant chemotherapy, radiation therapy, followed by a delayed, “second-look” surgery. Delayed surgery is commonly recommended for the patients who show residual tumors after chemotherapy and radiotherapy are completed. However, some authors advocate surgery after a few cycle of chemotherapy before the patients undergo radiotherapy for the tumors which show minimal response. The tumors thus resected were mostly mature teratomas (Goldman et al. 2015; Ogiwara et al. 2015). Delayed surgical resection of NGGCTs confers several advantages to patients. First, neoadjuvant therapies can reduce the tumor burden and make the surgery easier to accomplish. Second, neoadjuvant therapies also reduce tumor vascularity and delayed surgical resection enforces less blood loss of the patient. Third, generally, malignant components of NGGCTs are more susceptible to neoadjuvant therapies than teratoma components. After neoadjuvant therapies, tumor is devoid of highly vascular malignant tissues and the potential risk of tumor dissemination during surgery is also diminished. Finally, many patients with NGGCTs may not need delayed surgical resection if tumors disappear after chemotherapy and/or radiation therapy. In a recent clinical trial assessing the outcome of neoadjuvant chemotherapy for NGGCTs, six cycles of chemotherapy yielded CR or PR in 69% of patients (Goldman et al. 2015). Therefore, at present, neoadjuvant chemotherapy is considered a reasonable initial treatment for NGGCTs except for mature teratomas. However, surgical resection of NGGCTs should be undertaken in some situations. Immature teratomas and mixed GCTs composed predominantly of teratoma components often grow rapidly during neoadjuvant therapies, causing a mass effect and increased ICP (Vaughn et al. 2009). This phenomenon is known as growing teratoma syndrome (GTS) in which the mature teratoma component, especially multiple cystic structures, expand rapidly (Kim et al. 2011). GTS is a definite surgical indication and delaying surgery can be fatal in some patients.
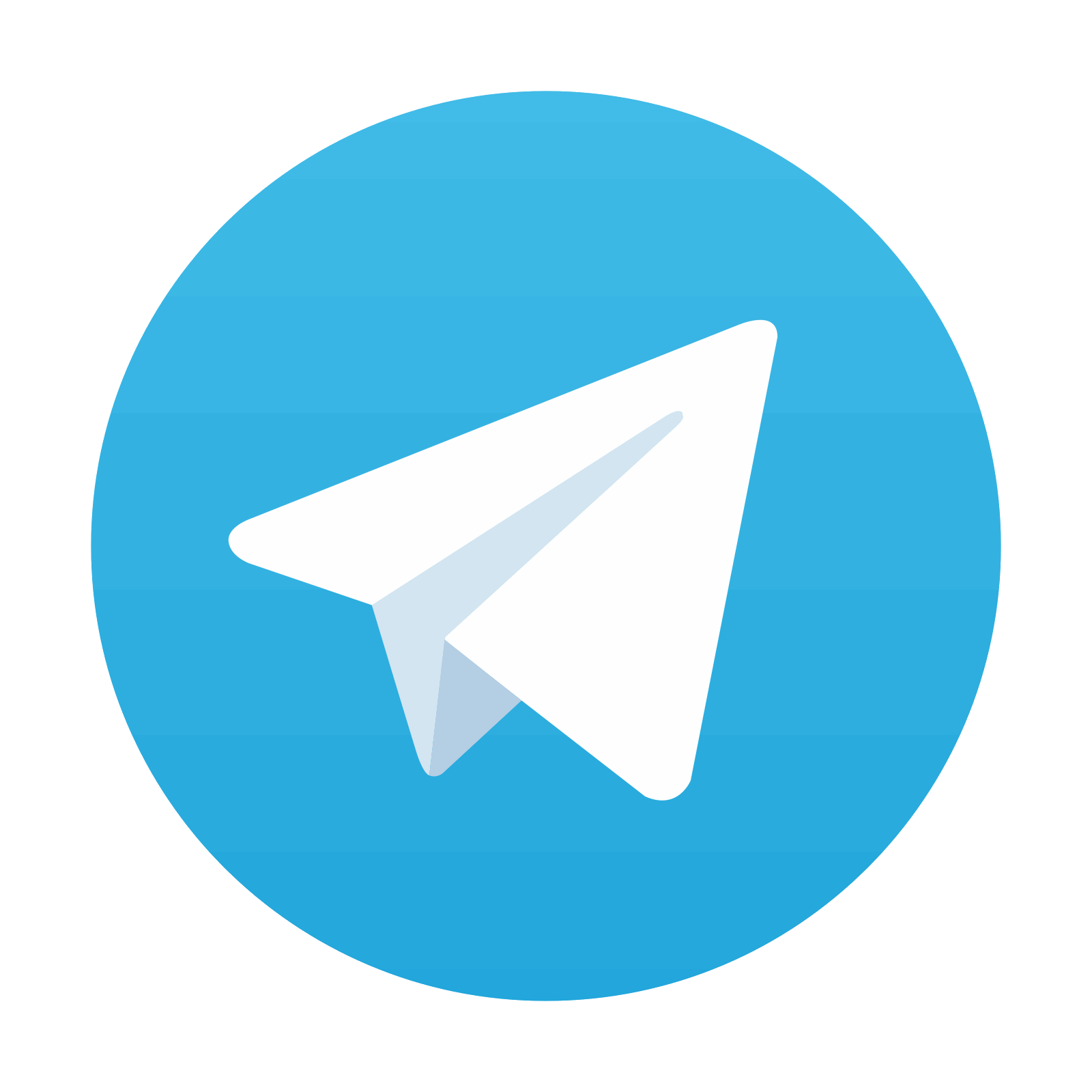
Stay updated, free articles. Join our Telegram channel

Full access? Get Clinical Tree
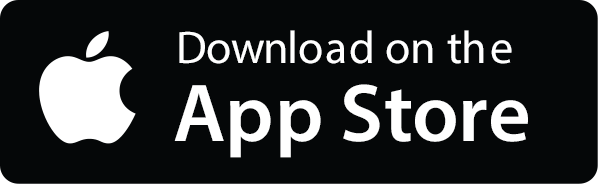
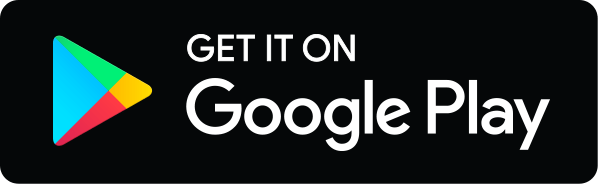