(1)
Institut Bergonié, Bordeaux, France
Abstract
Cell cycle consists of the sequential events occurring between the emergence of a new cell and that of the two daughter cells generated by the first one. Cell cycle integrates a continuous growth cycle (increase in cell mass) and a discontinuous division chromosomal cycle (DNA replication and mitosis, i.e. distribution of the genome between the two daughter cells). Cell cycle entry represents, therefore, the execution of the cell proliferation programme, in response to the messages brought by growth factors, as studied in the previous chapters. The transduction of these messages leads, among other events, to the transcription of the genes required for cell cycle entry, the first one being cyclin D1. Cell cycle control is of crucial importance in oncogenesis, as the loss of some of its essential checkpoints characterises malignant transformation.
We present here essentially the pathways used for triggering and controlling cell cycle progression, and not the events themselves (DNA replication, mitosis). After a brief description of the main events that occur during the different phases of the cycle, we will present the effectors of these events, then the control processes themselves, before the description of the alterations of cell cycle regulation in cancer. In the early days, cell cycle was only defined by two phases, mitosis and interphase; DNA replication was afterwards recognised as an active phase, separated from mitosis by two gaps; these gaps are in no way resting phases, but important periods during which are actively prepared the events leading to DNA replication and mitosis.
17.1 Cell Cycle Phases
17.1.1 G1 Phase
G1 phase, between mitosis and DNA synthesis, has the most variable length among cell cycle phases. If nutriments are lacking or if the cells receive an antiproliferative signal, or a signal inducing terminal differentiation, the cells can delay their progression in the cycle or leave the cycle and enter a quiescent phase, called G0. G1 progression is controlled by a checkpoint on DNA integrity; once this checkpoint has been cleared, the cycle is engaged without any possibility of stopping. DNA replication is prepared in G1 through derepression of transcription factors, which enable the transcription of the genes required for replication. This derepression involves their release from proteins that retain them and are called pocket proteins. The synthesis of the purine and pyrimidine nucleotides aimed at DNA incorporation requires numerous enzymes such as dihydrofolate reductase, thymidylate synthase and many others; DNA replication itself also requires numerous enzymes such as diverse polymerases, primase, helicases, topoisomerases, etc. The promoters of many of the genes encoding these proteins are activated during the G1 phase.
17.1.2 S Phase
DNA replication is simultaneously initiated on numerous different sites, called origins of replication. Each DNA region that is replicated from a given origin is called a replicon. Each group of replicons is replicated at a characteristic time of the S phase, generally at its beginning for the actively transcribed regions, whereas the regions containing transcriptionally inactive heterochromatin are transcribed later.
During the G1 phase, the chromosomes are modified in order to obtain the ‘authorisation’ to replicate by binding special proteins at the origins of replication, so that they form a pre-replication complex. During replication, these complexes are inactivated, preventing thus the chromosomal regions to replicate two times during the cycle. Phase S steps (see Annex A) comprise the opening of the double helix (requiring, for instance, helicases, topoisomerase 2, etc.) and the synthesis of DNA (requiring, for instance, primase, DNA polymerases, etc.). In addition to these enzymes, several proteins are required, associated to the initiation, elongation and termination processes, as well as histones for DNA packaging in nucleosomes.
17.1.3 G2 Phase
During the G2 phase, between DNA synthesis and mitosis, cells can make corrections to DNA structure, thanks to DNA repair processes, and prepare to mitosis. If damaged or non-replicated DNA is detected at the G2 checkpoint, a cascade of protein kinase activities is triggered, leading to the inactivation of cell cycle progression. Furthermore, protection mechanisms prevent the cell from initiating a new DNA replication process as long as mitosis has not occurred.
17.1.4 M Phase
During M phase and the subsequent cytodieresis (often improperly called cytokinesis), chromosomes and cytoplasm are divided to form two daughter cells. Chromosome segregation is controlled by the metaphase checkpoint, which delays chromatid separation until chromosomes are correctly in alignment on the equatorial plate of the mitotic spindle. Mitosis is divided in five phases (Fig. 17.1):
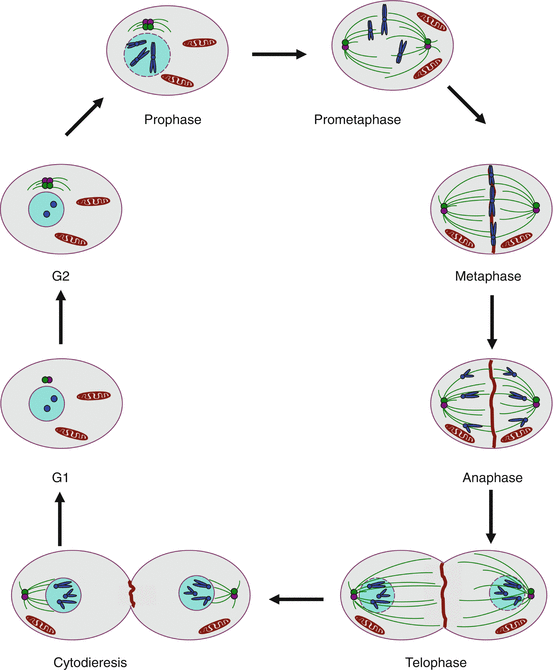
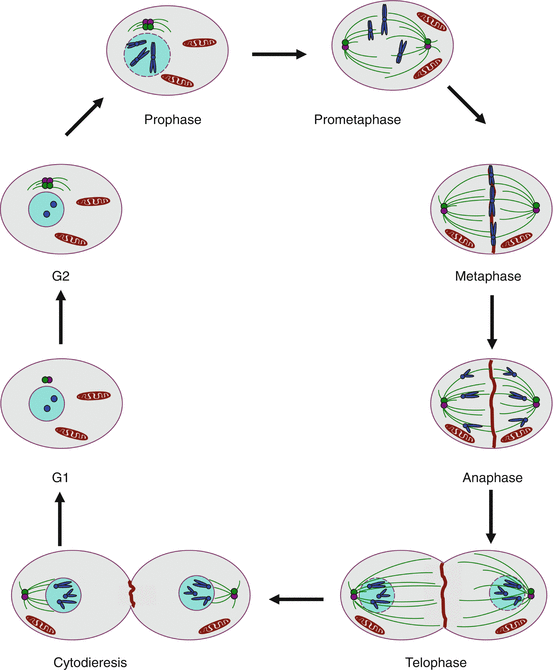
Fig. 17.1
Schematic representation of a mitosis. Prophase: chromatin is condensed to form chromosomes that gather the two sister chromatids; the centrosomes, duplicated in G2, get organised and serve as nucleation starters for microtubules. Prometaphase: the nuclear envelope is disrupted, the centrosomes migrate at two opposite poles of the cell and the microtubules get organised as a spindle on which chromosomes are attached at the level of their kinetochore. Metaphase: the chromosomes get aligned along the equatorial plate after their ‘capture’ by microtubules. Anaphase: the two sister chromatids are separated by APC/C (anaphase-promoting complex); the chromatids are directed to opposite poles and the microtubular spindle is spread in the entire cell. Telophase: the two copies of the genome are distributed at each pole of the cell, the nuclear envelope is reconstituted and a pinch is formed between the two opposite poles. Cytodieresis: a contractile ring is formed between the two nuclei and the daughter cells can separate
Prophase: chromatin is condensed into chromosomes; centrosomes, duplicated during the G2 phase, are organised and serve as nucleation origins for microtubules.
Prometaphase: the nuclear envelope is disrupted, the centrosomes migrate at the two poles of the cell and the microtubules are organised as a spindle where chromosomes are attached, at the level of their kinetochore.
Metaphase: chromosomes align at half-distance of the two poles, on an ‘equatorial plate’, after they have been ‘captured’ by the microtubules.
Anaphase: the homologous chromosomes leave the equatorial plate after deactivation of cohesins and activation of the separase complex enabled by the degradation of securin (gene PTTG1) in the proteasome, where it is driven by the APC/C–CDC20 (anaphase-promoting complex/cyclosome–cell division cycle 20) complex; homologous chromosomes migrate in opposite directions to the poles.
Telophase: the two copies of the genome are distributed at each pole of the cell, the nuclear envelope is reconstituted and a tightening occurs between the two nuclei.
Cytodieresis: a contractile actin ring is assembled at half-distance of the two poles and tightens the equatorial zone. This process enables the separation of the daughter cells.
17.2 Effector Proteins of Cell Cycle Control
17.2.1 Cyclins and Cyclin-Dependent Kinases
Cyclins (CCNs) are proteins of 35–90 kDa with no catalytic activity, but indispensable to the activity of serine/threonine kinases which are thus called cyclin-dependent kinases (CDKs). They are constituted of two central symmetric domains comprising five α helices. One of these domains (cyclin box) is highly conserved and represents the structural characteristic of these proteins. Cyclins undergo cyclic production and are present in the cell at definite periods of the cell cycle: cyclins D during G1 phase, cyclin E at the end of the G1 phase, cyclin A during S and G2 phases and cyclins B during M phase. Synthesis and degradation of cyclins follow thus a precise regulation, which requires definite transcription factors for synthesis and E3 ubiquitin ligases for their proteasomal destruction.
Cyclin-dependent kinases (CDKs) are generally smaller than cyclins (30–40 kDa) and display serine/threonine kinase activity only after binding with a cyclin. Cyclin binding to CDKs induces a conformational change enabling the ATP present in the active centre to react with protein substrates. CDKs are present in constant amounts all along the cell cycle: this is indeed the binding to a stimulatory cyclin that constitutes the decisive event for them to play their catalytic role. CDK4 or CDK6 binds cyclin D during the G1 phase, CDK2 binds cyclin E at the end of the G1 phase and cyclin A during the S phase; CDK1 binds cyclin A during the G2 phase and cyclin B during the M phase. Figure 17.2 presents the intervention of the cyclin–CDK complexes during cell cycle progression.
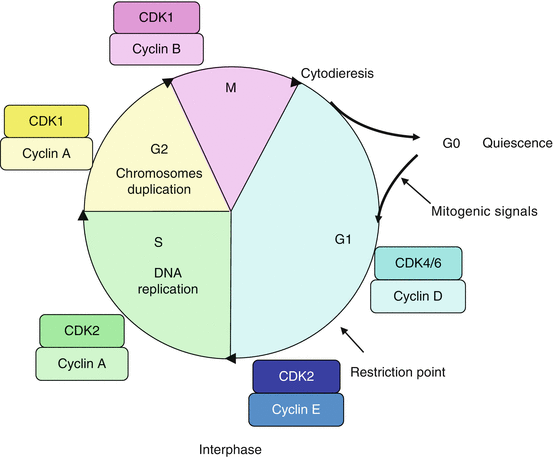
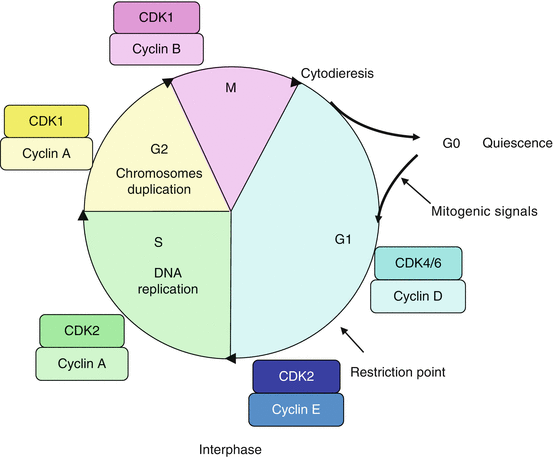
Fig. 17.2
Cyclin–CDK complexes and their intervention during the cell cycle. Cyclins are produced during precise periods of the cell cycle; by associating to cyclin-dependent kinases (CDK), they enable these kinases to phosphorylate the proteins required for cell cycle progression. The cyclin D–CDK4/6 complexes are involved in cell cycle progression in G1; the cyclin E–CDK2 complex allows cell entry into S phase; the cyclin A–CDK2 and cyclin A–CDK1 complexes are active during the S and G2 phases, respectively; the cyclin B–CDK1 complex appears at the end of the G2 phase and constitutes the triggering factor of mitosis
Each cyclin–CDK couple presents phase specificity and substrate specificity for the proteins they can phosphorylate. Some of these substrates are well identified, for instance the RB1 (retinoblastoma 1) protein for the complex cyclin D–CDK4/6 (see below), but all substrates have not yet been identified. As a general feature, the cyclin–CDK substrates are either enzymes that intervene in a specific way to catalyse a step required for cell cycle progression, or transcription factors enabling the synthesis of the proteins required for these processes. A special cyclin–CDK couple operate as activators of other cyclin–CDK couples: this is the cyclin H–CDK7 couple which, when associated to a third protein, MAT1 (Ménage à trois 1), phosphorylates the cyclin D–CDK4/6 and the cyclin B–CDK1 complexes on a threonine residue (Thr161 for CDK1), rendering them up to 300-fold more active. This is why the cyclin H–CDK7 is also known as CAK (CDK–activating kinase). This complex also operates in DNA repair (Annex A) and cannot activate a new mitosis as long as this repair has not been carried out.
17.2.2 Inhibitory Kinases WEE1 and MYT1
In addition to the cell cycle-activating kinase complexes, several inhibitory kinases can delay cell cycle progression. WEE1 (from the name of the ‘petite’ yeast mutation in Scottish) can phosphorylate CDKs on a tyrosine residue (Tyr15 for CDK1) while MYT1 (myelin transcription factor 1) phosphorylates CDKs on a threonine residue (Thr14 for CDK1), at the level of the ATP-binding site. They inhibit thus the kinase activity of the target CDKs, which will require reactivation thanks to phosphatases of the CDC25 subfamily (see below). WEE1 and MYT1 are deactivated by phosphorylation catalysed by AKT, a key element of the PI3 kinase pathway (Chap. 3), as well as by ubiquitinylation that drives them to the proteasome (Annex C).
17.2.3 Phosphatases
In addition to the two kinase types, stimulatory and inhibitory, two types of phosphatases, conversely inhibitory and stimulatory, hydrolyse the phosphate moieties brought by the kinases. Inhibitory phosphatases operate thus against CDK function and remove the phosphate brought by CAK (Thr161 for CDK1); it has been called KAP or KAC (gene CDKN3) by inverting the acronym CAK. Stimulatory phosphatases remove the phosphate groups brought by WEE1 and MYT1; these are called CDC25s, a small family of three different enzymes (CDC25A, B and C) with overlapping specificities.
CDC25A seems rather involved in the regulation of the G1 → S transition and CDC25B and CDC25C in the G2 → M transition. CDC25 are major targets for the control checkpoint of DNA integrity in G2. The activation of cyclin–CDK complexes by CDC25 phosphatases constitutes the triggering event for these complexes. CDC25 phosphatases can be activated by phosphorylation catalysed by PLK1 (Polo–like kinase 1) and by cyclin–CDK complexes, in a positive retroactive loop, and deactivated by phosphorylations on other residues by the checkpoint kinases CHK1 and CHK2 (genes CHEK1 and CHEK2), two serine/threonine kinases activated by the kinases in charge of DNA damage detection, ATM and ATR (see below).
17.2.4 Protein Inhibitors of CDKs
In addition to the regulation system involving phosphorylation–dephosphorylation processes, a system of regulation through protein–protein interactions has been identified. It involves two families of small protein inhibitors of CDKs that are called CKIs. The first family is INK4 (inhibitors of kinases) and comprises four proteins with molecular weights of 16, 15, 18 and 19 kDa, respectively encoded by genes INK4a, INK4b, INK4c and INK4d (now CDKN2A, CDKN2B, CDKN2C and CDKN2D), and usually called p16INK4a, p15INK4b, p18INK4c and p19INK4d. These proteins inhibit the binding of cyclins D to CDK4 or 6 and modify the conformation of the CDKs and their ATP-binding site. The second family is called CIP/KIP and comprises three proteins: p21CIP1, p27KIP1 and p57KIP2, which indicates both their molecular weights and the (older) names of the genes that encodes them, which now are CDKN1A, CDKN1B and CDKN1C, respectively. These proteins bind the cyclin E–CDK2 complex and strongly inhibit its function, firstly by modifying the conformation of the CDK, secondly by binding the ATP site. The cyclin E–CDK2 complex phosphorylates the KIP proteins, enabling their transfer to the proteasome by the E3 ubiquitin ligase complex SKP1–SKP2 (S phase kinase–associated protein 1/2). The AKT protein of the PI3 kinase pathway phosphorylates p21 and p27, ensuring thus their inhibition (Chap. 3).
CKI plays a fundamental role in cell growth regulation during the G1 and G0 phases, causing cell cycle arrest in response to antiproliferative signals, while this inhibition is alleviated in the presence of proliferation signals.
17.2.5 Mitotic Kinases
Two other serine/threonine kinase families are involved in cell cycle, the aurora kinase family (genes AURKA, AURKB and AURKC) and the polo-like kinase family (genes PLK1 to PLK5); they are mainly involved in mitosis control. The aurora kinases can autophosphorylate when activated by appropriate protein interactions. AURKA controls mitosis entry by activating CDC25B and PLK1 by phosphorylation; it then ensures centrosomes separation and maturation, recruiting the proteins required to the assembly of the mitotic spindle. AURKB is localised at the level of the chromosomal kinetochores and ensures the attachment of the kinetochores on the spindle. AURKC principally intervenes during meiosis.
The polo-like kinases are present at the level of the spindle poles and of chromosome kinetochores. PLK1 is phosphorylated by AURKA, then by AURKB, and phosphorylates a series of substrates whose activation or inactivation is required to ensure their functions during mitosis, such as cohesin and separase during anaphase. PLK1 ensures thus the coordination of the successive steps of the mitosis. The other PLKs have been less studied.
17.2.6 Checkpoint Kinases and DNA Integrity Control Kinases
As mentioned earlier, there are two checkpoint kinases, CHK1 and CHK2 (genes CHEK1 and CHEK2), which are involved in the control of cell cycle progress at the level of the G1 → S and of the G2 → M transitions, respectively. They inhibit the corresponding CDC25 phosphatases by phosphorylation and can therefore delay cell cycle progression. They are under the control of other kinases that check DNA integrity, ATM (ataxia telangiectasia mutated), ATR (ataxia telangiectasia and Rad–3 related) and DNA-dependent protein kinase, DNAPK (gene PRKDC).
17.2.7 Biochemical Mechanisms of Cell Cycle Regulation
Several mechanisms of regulation of the controlling elements of the cell cycle have been described here: (1) protein–protein interactions (between cyclins and CDKs, between CKIs and cyclin–CDK complexes); (2) phosphorylation–dephosphorylation processes (kinase and phosphatase activities, either on tyrosine or on serine/threonine residues); (3) proteasomal destruction of proteins such as cyclins, WEE1 and p27KIP1 after ubiquitinylation. Other regulatory mechanisms can operate to control the activity of the cyclin–CDK complexes, such as their translocation from the cytoplasm where they are synthesised to the nucleus where they must operate. Cyclin phosphorylations appear to be responsible for these translocations. Figure 17.3 presents the main positive and negative regulatory processes that ensure cell cycle progression.
