Chapter 9 Cell Cooperation in the Antibody Response
• The primary development of B cells is antigen independent. Pre-B cells recombine genes for immunoglobulin heavy and light chains to generate their surface receptor for antigen.
• T-independent antigens activate B cells without requiring T-cell help. They can be divided into two groups. TI-1 antigens can act as polyclonal stimulators, while TI-2 antigens are polymers which activate by cross-linking the B-cell receptor.
• T-dependent antigens are taken up by B cells, processed and presented to TH cells. T cells and B cells usually recognize different parts of an antigen.
• B-cell activation requires signals from the B-cell receptor and costimulation. CD40 is the most important costimulatory molecule on B cells. Ligation of the B-cell–coreceptor complex can lower the threshold of antigen needed to trigger the B cell. Intracellular signaling pathways are analogous in B cells and T cells.
• Activated B cells proliferate and differentiate into antibody-forming cells. Cytokines from TH cells control the process of division, differentiation and class switching.
• Somatic hypermutation of immunoglobulin genes, followed by selection of high-affinity clones is the basis of affinity maturation. These processes occur in germinal centers.
• Class switching is effected by somatic recombination occurring within the heavy chain genes. Somatic hypermutation and class-switching by recombination are linked processes, which require selective targeting of DNA-modification and DNA-repair enzymes to the heavy chain gene locus.
Development of B cells
Primary B-cell development is antigen independent
Within the bone marrow, a sequence of immunoglobulin rearrangements and phenotypic changes takes place during B-cell ontogeny, analogous to that described for T cells in the thymus which leads to the production of the B cell’s antigen receptor (Fig. 9.w1). The molecular processes involved in immunoglobulin gene rearrangement have been described in Chapter 3, and this section relates these events to B-cell development.
• terminal deoxynucleotidyltransferase (TdT), an intranuclear enzyme uniquely expressed during Vh-gene rearrangement; and
• B220, which identifies a particular splice-variant of leukocyte common antigen (CD45R in humans), a tyrosine phosphatase that appears to be important in regulating B-cell receptor signaling.
Genes for immunoglobulin H and L chains are recombined to generate the surface receptor
Pre-B cells can be divided into large mitotically active pre-B cells and small non-dividing pre-B cells. Both large and small pre-B cells express Igμ heavy chains in the cytoplasm (cμ) and the pre-B cell–receptor complex on their surface (Fig. 9.w2). Large pre-B cells have successfully rearranged their Ig heavy chain genes. As these cells pass from the large pre-B cell group into the small pre-B cell group, they begin to rearrange their Ig light chain genes and upregulate RAG-1 and RAG-2. The final stage of B-cell development is the immature B-cell stage. Immature B cells have successfully rearranged their light chain genes and express IgM. Once again, RAG-1 and RAG-2 expression has been downregulated. As immature B cells develop further into mature B cells, they begin to express both IgM and IgD on their surface. These mature B cells are then free to exit the bone marrow and migrate into the periphery.
Other phenotypic markers such as CD25 (IL-2Rα chain), CD79a (Igα), CD79b (Igβ), and c-Kit can help to identify particular populations of pro-B, pre-B or immature B cells (see Fig. 9.w1).
Transcriptional controls in B-cell development
B-lymphopoiesis is regulated by multiple transcription factors including:
• E2A and EBF, which coordinately activate early B-cell genes;
• Pax5, which ensures development to B-cell lineages by restricting transcription of lineage inappropriate genes and activating expression of B-lineage signaling molecules;
• Sox4 and LEF1, which promote the survival and proliferation of pro-B cells;
• IRF4 and IRF8, which terminate pre-BCR signaling by IRF4 and promote differentiation to small pre-B cells; and
• Bcl-6 expression, which is required for germinal B-cell differentiation and generation of memory B-cells.
B cell activation
T-independent antigens do not require T cell help to stimulate B cells
A small number of antigens, however, can activate B cells without MHC class II-restricted T cell help and are referred to as T-independent (TI) antigens (Fig. 9.1).
• TI-1 antigens are predominantly bacterial cell wall components – the prototypical TI-1 antigen is lipopolysaccharide (LPS), a component of the cell wall of Gram-negative bacteria;
• TI-2 antigens are predominantly large polysaccharide molecules with repeating antigenic determinants (e.g. Ficoll, dextran, polymeric bacterial flagellin, and poliomyelitis virus).
T-independent antigens induce poor memory
Primary antibody responses to TI antigens in vitro are generally slightly weaker than those to TD antigens. They peak fractionally earlier and both generate mainly IgM. However, the secondary responses to TD and TI antigens differ greatly. The secondary response to TI antigens resembles the primary response, whereas the secondary response to TD antigens is far stronger and has a large IgG component (Fig. 9.2). It seems, therefore, that TI antigens do not usually induce the maturation of a response leading to class switching or to an increase in antibody affinity, as seen with TD antigens. This is most likely due to the lack of CD40 activation (see below). Memory induction to TI antigens is also relatively poor.
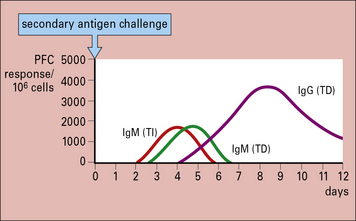
Fig. 9.2 Comparison of the secondary immune response to T-dependent and T-independent antigens in vitro
The secondary response to T-dependent antigens is stronger and induces a greater number of IgG-producing cells, as measured by plaque-forming cells (see Method box 9.1).
It is possible to convert TI antigens into T-dependent antigens by altering their structure. For example, pneumococcal polysaccharides are TI antigens and do not induce immunological memory or antibodies in infants. However, conjugation of pneumococcal polysaccharides to a carrier protein induces polysaccharide-specific antibody in infants, and memory similar to T-dependent antigens (see Method boxes 9.1 and 2.1).
Method box 9.1 Measuring antibody production – the PFC assay and ELISPOT
Antibody-forming cells (AFCs) are measured by means of a quantitative PFC assay (Fig. MB9.1.5), originally developed by Niels Jerne in the 1960s. B cells (e.g. spleen cells) are plated in agar with sheep red blood cells sensitized by binding the specific antigen to their surface. Antibody produced by any B cell will coat the red blood cells. Following the addition of complement, these coated cells may be lyzed, causing the appearance of a zone of lyzed cells (plaque) in the agar. Figure MB9.1.5 shows the appearance of such a plaque, with a B cell in the center, under the microscope. The plaques are then counted to give a quantitative measure of the number of PFCs.
Another way of detecting antibody-producing cells is by means of an enzyme-linked immunospot assay (ELISPOT). An ELISPOT assay starts out by coating a plastic well with antigen and adding a known quantity of B cells. The antigen coated onto the plastic will then capture any antibody in the vicinity of the activated B cell that is producing the antibody. After a period of time, the B cells are removed, and the specific antibody can be detected by adding an enzyme-labeled anti-immunoglobulin plus chromogen. Development of the label in this assay results in a spot surrounding the active B cell. Counting each spot and knowing the quantity of B cells originally added to the well allows one to enumerate the frequency of B cells producing the specific antibody. Method Box 2.1, Fig. MB2.1.3 shows the method of detecting antibodies and the appearance of the spots on the developed plates. In addition to analyzing specific antibody-secreting B cells, the ELISPOT assay has been adapted to measure the frequency of cytokine-secreting T cells and various other cell types (right panel). With the improvement in ELISPOT assay plate design and in ELISPOT detection equipment, antibody- or cytokine-secreting cells can now be detected at the single cell level.
Activation of B cells by T-dependent antigens
T cells and B cells recognize different parts of antigens
By manipulating the cell populations in these experiments, it was shown that:
These experiments were later reinforced by details of how:
This has led to the basic scheme for cell interactions in the antibody response set out in Figure 9.3. It is proposed that antigen entering the body is processed by cells that present the antigen in a highly immunogenic form to the TH and B cells. The T cells recognize determinants on the antigen that are distinct from those recognized by the B cells, which differentiate and divide into antibody-forming cells (AFCs). Therefore two processes are required to activate a B cell:
• antigen interacting with B cell immunoglobulin receptors – this involves ‘native’ antigen;
• stimulating signal(s) from TH cells that respond to processed antigen bound to MHC class II molecules.
B-cell activation and T-cell activation follow similar patterns
Cross-linking of surface Ig leads to activation of the src family kinases, which in B cells are Fyn, Lyn, and Blk. Syk is analogous to ZAP-70 in T cells, and binds to the phosphorylated ITAMs of Igα and Igβ (Fig. 9.4). This leads to activation of a kinase cascade and translocation of nuclear transcription factors analogous to the process that occurs in T cells.
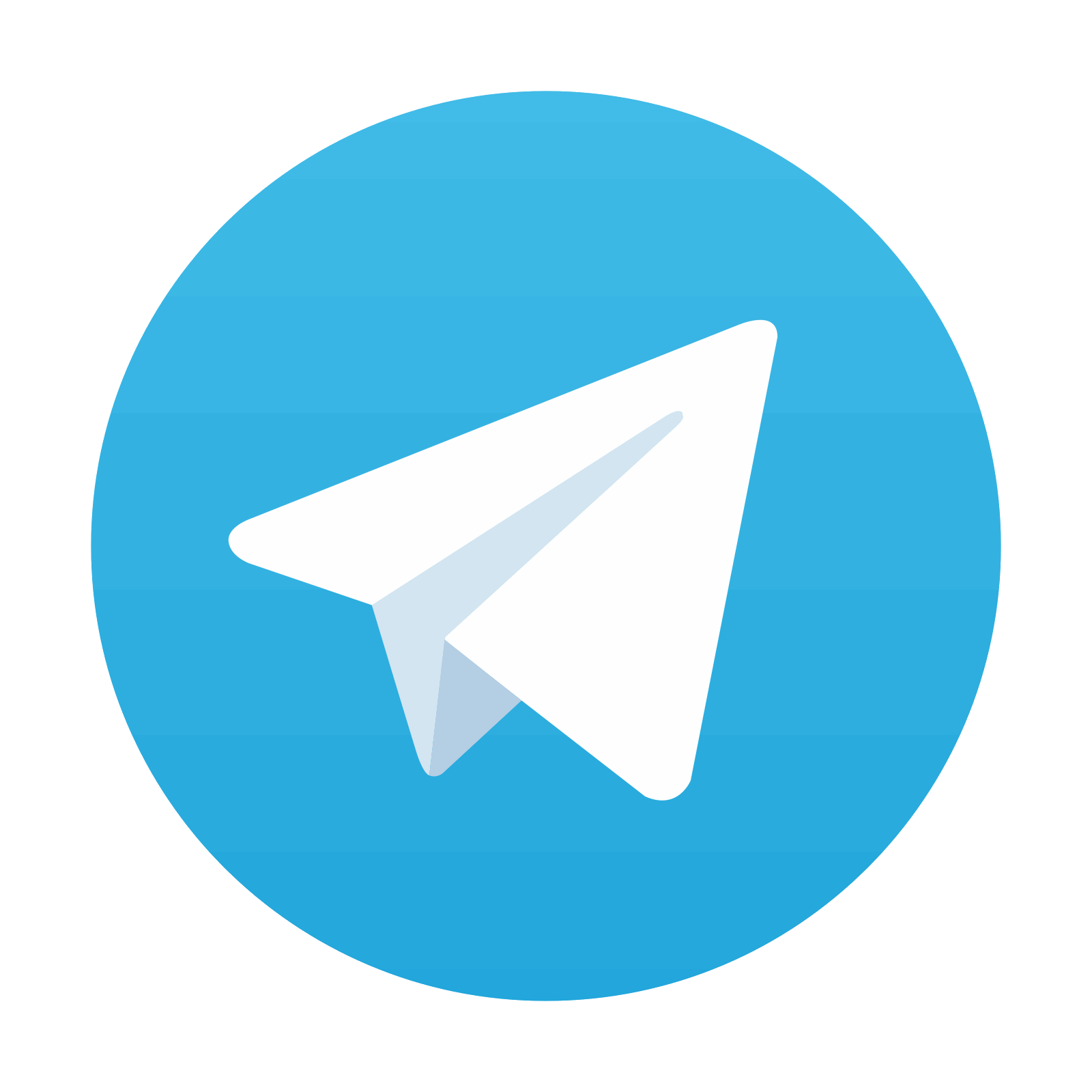
Stay updated, free articles. Join our Telegram channel

Full access? Get Clinical Tree
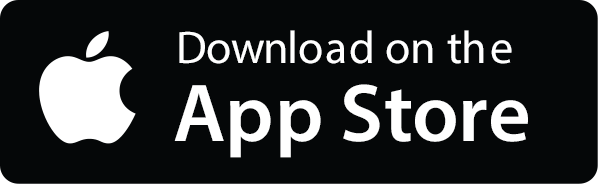
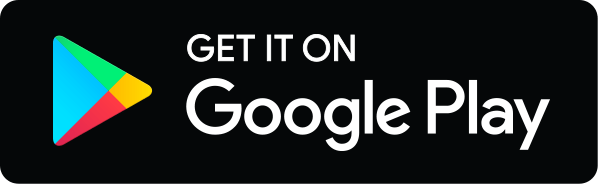