Risk Factor
Comment
References
Cumulative anthracycline dose
Cumulative doses >500 mg/m2 are associated with significantly elevated long-term risk
Time after therapy
Incidence of clinically important cardiotoxicity increases progressively after therapy
Rate of anthracycline administration
Prolonged administration to minimize circulating dose may decrease toxicity; results are mixed
Individual anthracycline dose
Higher individual doses are associated with increased late cardiotoxicity, even when cumulative doses are limited; no dose is risk-free
Type of anthracycline
Liposomal encapsulated preparations may reduce cardiotoxicity. Data on anthracycline analogues and differences in cardiotoxicity are conflicting.
Radiation therapy
Cumulative radiation dose >30 Gy; as little as 5 Gy increases the risk; before or concomitant with anthracycline treatment
Concomitant therapy
Trastuzumab, cyclophosphamide, bleomycin, vincristine, amsacrine, and mitoxantrone, among others, may increase susceptibility or toxicity.
Pre-existing cardiac risk factors
Hypertension; ischemic, myocardial, and valvular heart disease; prior cardiotoxic treatment
Barry et al., 2007 [19]
Personal health habits
Smoking, alcohol consumption, energy drinks, stimulants, prescription and illicit drugs
Lipshultz et al., 2010 [3]
Comorbidities
Diabetes, obesity, renal dysfunction, pulmonary disease, endocrinopathies, electrolyte and metabolic abnormalities, sepsis, infection, pregnancy, viruses, elite athletic participation, low vitamin D levels
Age
Both young and advanced age at treatment are associated with elevated risk
Sex
Females are at greater risk than males
Complementary therapies
38 % of Americans spend $34 billion on nonscientific, alternative, or complementary self-care therapies and medicines; more information needs to be collected to assess risk
Lipshultz et al., 2010 [3]
Additional factors
Trisomy 21; African American ancestry
Krischer et al., 1997 [13]
7.2 Pathophysiology: Antineoplastic Agents Associated with Cardiotoxicity
7.2.1 Chemotherapeutics
Many pediatric patients with cancer receive chemotherapeutic agents that have potentially cardiotoxic effects. Anthracyclines are well known for their cardiotoxicity, and alkylating agents, such as cyclophosphamide, ifosfamide, cisplatin, busulfan, and mitomycin, have also been associated with cardiotoxicity. Other agents reported to have cardiac effects include vinca alkaloids, fluorouracil, cytarabine, amsacrine, and asparaginase and the newer agents—paclitaxel, trastuzumab, etoposide, and teniposide (Table 7.2) [29].
Table 7.2
Cardiotoxic effects of chemotherapeutic agents
Drug class/agent | Adverse cardiac events |
---|---|
Anthracycline/anthraquinolones | |
Doxorubicin, daunorubicin, epirubicin, idarubicin, mitoxantrone | Arrhythmias, pericarditis, myocarditis, HF, LV dysfunction |
Alkylating Agents | |
Busulfan, cisplatin, cyclophosphamide, ifosfamide, mitomycin | Endomyocardial fibrosis, pericarditis, cardiac tamponade, ischemia, MI, hypertension, myocarditis, HF, and arrhythmias |
Antimetabolites | |
Capecitabine, cytarabine, 5-fluorouracil, clofarabine, carmustine | Ischemia, chest pain, MI, HF, arrhythmias, pericarditis, pericardial effusions, and hemodynamic abnormalities |
Antimicrotubules | |
Etoposide, teniposide, paclitaxel, vinca alkaloids | Sinus bradycardia, angina, hypotension or hypertension, HF, ischemia, MI, arrhythmias, conduction abnormalities |
Biological agents | |
Alemtuzumab, bevacizumab, cetuximab, rituximab, trastuzumab | Hemodynamic abnormalities, LV dysfunction, HF, thromboembolism, angioedema, arrhythmias |
Interleukins | |
Interleukin-2, interferon-α, denileukin | Hypotension, arrhythmias, capillary leak syndrome, ischemia, LV dysfunction, coronary artery thrombosis |
Tyrosine kinase inhibitors | |
Imatinib mesylate, sorafenib, sunitinib, dasatinib, relotinib, gefitinib, lapatinib | HF, edema, pericardial effusion, pericarditis, hypertension, arrhythmias, ischemia, prolonged QT interval, chest pain |
Miscellaneous | |
Asparaginase, pentostatin, arsenic trioxide, all-trans-retinoic acid, thalidomide, lenalidomide | HF, hypotension, MI, electrocardiographic changes, pleural-pericardial effusion, QT prolongation, peripheral edema, bradycardia, ischemia, edema, thromboembolism and retinoic acid syndrome that includes fever, respiratory distress, weight gain, angina, Torsades de Pointes |
7.2.1.1 Anthracyclines
Anthracycline-induced cardiotoxicity has been recognized for over 40 years. Most long-term pediatric cancer survivors received anthracyclines, which are widely used to treat several solid tumors and hematologic malignancies [13]. Despite their wide use, the mechanism by which cardiotoxicity occurs is not well understood, though the cause is likely due to more than one variable.
Anthracyclines belong to the antineoplastic antibiotic class that interferes with cell replication by reacting with lipids, proteins, and nucleic acids—resulting in lipid peroxidation, depletion of sulfhydryl-containing peptides, and DNA damage [18]. The cytotoxic action of anthracyclines works through three major mechanisms [30]:
1.
Inhibition of DNA and RNA synthesis by intercalating between base pairs of the DNA/RNA strand, thus preventing the replication of rapidly growing cancer cells.
2.
Inhibition of the topoisomerase II enzyme, preventing supercoiled DNA from relaxing and thus blocking DNA transcription and replication.
3.
Creation of iron-mediated, free-oxygen radicals that damage the DNA and cell membranes.
The most commonly hypothesized mechanism concerns the generation of free radicals and superoxides, known as radical oxygen species (ROS; Fig. 7.1) [31].
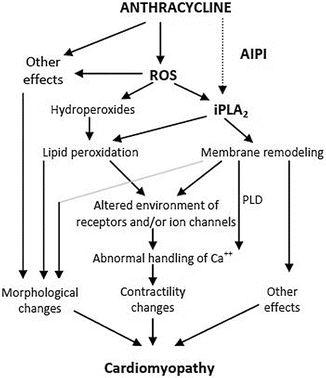
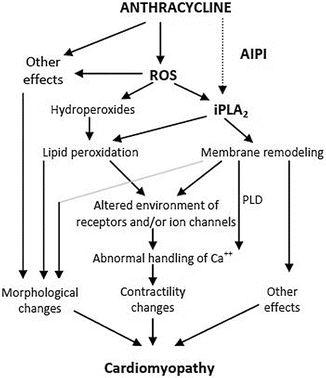
Fig. 7.1
Proposed pathways of anthracycline-induced cardiomyopathy. ROS reactive oxygen species, AIPI anthracycline-induced iPLA2 inhibition, iPLA 2 independent phospholipase A2, PLD phospholipase D. Adapted from McHowat et al. 2004 [31]. Recreated with permission from American Society for Pharmacology and Experimental Therapeutics
Cardiomyocytes have low levels of free radical scavenging systems and a highly oxidative metabolism, making them more susceptible to injury by this mechanism [21, 32]. Although the oxidative effects of anthracyclines may not be limited to cardiomyocytes, rapidly dividing intracardiac non-muscle cells may be able to replace those lost to apoptosis or necrosis leading to fibrous replacement even though anthracyclines retard cardiac fibroblast proliferation. Cardiomyocytes, which divide very slowly, if at all, may not sufficiently replace cells damaged during treatment [26].
The degree and progression of anthracycline-related toxicity varies widely among individuals, suggesting a genetic predisposition and the presence of modifiable and non-modifiable risk factors [26].
Three types of anthracycline-induced cardiotoxicity have been described (Table 7.3).
Table 7.3
Characteristics of the types of anthracycline-associated cardiotoxicitya
Characteristic | Acute cardiotoxicity | Early onset, chronic progressive cardiotoxicity | Late onset, chronic progressive cardiotoxicity |
---|---|---|---|
Onset | Within the first week of anthracycline treatment | <1 year after the completion of anthracycline treatment | >1 year after the completion of anthracycline treatment |
Risk factor dependence | Unknown | Yes | Yes |
Clinical features in adults | Transient depression of myocardial contractility; myocardial necrosis (cTnT elevation); arrhythmia | Dilated cardiomyopathy; arrhythmia | Dilated cardiomyopathy; arrhythmia |
Clinical features in children | Transient depression of myocardial contractility; myocardial necrosis (cTnT elevation); arrhythmia | Restrictive cardiomyopathy and/or dilated cardiomyopathy; arrhythmia | Restrictive cardiomyopathy and/or dilated cardiomyopathy; arrhythmia |
Course | Usually reversible anthracycline is discontinued | Can be progressive | Can be progressive |
Acute or sub–acute cardiotoxicity is a rare form of cardiotoxicity that may occur immediately after a single dose or a course of anthracycline therapy. It may be characterized by transient electrophysiological abnormalities, a pericarditis-myocarditis syndrome, or acute LV dysfunction occurring within a week of treatment [34]. Acute changes during anthracycline infusion range from minor electrocardiographic abnormalities (non-specific ST segment and T-wave changes and QTc interval prolongation) to fatal arrhythmias, including transient systolic LV dysfunction and potentially fatal congestive HF [22, 35].
Early–onset chronic progressive cardiotoxicity is characterized by depressed myocardial function after 1 week of treatment or within the first year after treatment. The depression may persist or progress even after therapy is discontinued and may evolve into a chronic dilated cardiomyopathy in adults or children, and also a restrictive cardiomyopathy in children [27]. Early-onset cardiotoxicity is likely related to cardiomyocyte damage and death.
Late–onset chronic progressive cardiotoxicity is marked by deteriorated myocardial function occurring at least 1 year after completion of anthracycline therapy [10, 36, 37]. This deterioration has been attributed to impaired myocardial growth, as reflected by an inappropriately small increase in LV wall thickness in relation to somatic growth; with this, the loss of cardiomyocytes results in a progressively increased LV afterload and reduced LV contractility. The anthracycline-induced loss or damage to a critical number of cardiomyocytes might decrease the number of residual myocardial cells required to generate a normal myocardial mass, despite a marked increase in the size of the remaining cardiomyocytes [10]. Cardiomyocyte loss subsequently leads to LV wall thinning and, in some cases, to progressive LV dilation [38]. Beyond inadequate LV hypertrophy is the fact that many residual cardiomyocytes have abnormal intracellular structure and function that may persist and affect cardiac structure, function, and outcome. Cardiomyocyte mitochondrial structure and function are particularly affected by anthracycline exposure, and this may be persistent.
7.2.1.2 Alkylating Agents
Alkylating agents have also been associated with cardiotoxicity (Table 7.2). Cyclophosphamide is a non-cell-cycle-specific alkylating agent, and part of the core of many pre-transplant conditioning regimens. Its metabolite forms DNA crosslinks between (during inter-strand cross linkages) and within (during intra-strand cross linkages) DNA strands to cause irreversible cell damage, or cell death. The pathogenesis of cyclophosphamide-induced cardiotoxicity is not well understood, but it is thought to involve direct endothelial damage leading to leakage of plasma proteins and erythrocytes. Abnormal LV wall thickness from interstitial edema and hemorrhage may reduce LV diastolic compliance, creating LV diastolic dysfunction and presenting as restrictive cardiomyopathy [39].
In contrast to anthracycline-induced cardiotoxicity, high-dose cyclophosphamide can cause an acute myopericarditis peaking at 2–3 weeks after therapy and cardiac dysfunction independent of cumulative dose [40]. Acute HF has been reported as early as 1–3 weeks after administration [41, 42]. The total dose of cyclophosphamide per course has been described as a risk factor [40]. The combined incidence of symptomatic cyclophosphamide-induced cardiotoxicity from two studies [41, 42] was 22 %, and the incidence of fatal cardiotoxicity was 11 % [40]. A total dose greater than about 170 mg/kg over 4–7 days was a risk factor for cardiotoxicity [40–42]. In 52 patients who had never received anthracycline therapy, the overall incidence of symptomatic cyclophosphamide-induced cardiomyopathy was 25 %, and mortality was 12 % when the dose exceeded 1.55 g/m2 daily [40]. When the dose was <1.55 g/m2 daily, the incidence of symptomatic cardiotoxicity was 3 % with no mortality [40]. Since younger children, have a relatively higher body surface area, dosing of cyclophosphamide based on body surface area results in a much lower relative dose, which may partially explain the lower incidence and severity of cyclophosphamide-induced cardiotoxicity in younger children than that in adolescents and adults [43].
7.2.1.3 Mediastinal Radiation
Radiation therapy has greatly improved survival for children with Hodgkin’s disease and other malignancies of the chest. Successes with radiation therapy used either alone or in conjunction with other treatments have resulted in a cohort of survivors of childhood cancers who are subject to late complications from treatment, in which the therapeutic benefit is offset by potentially delayed cardiac effects.
Mediastinal radiation therapy has the potential to damage any structure of the heart. This damage may affect the heart valves, including valvular stenosis and regurgitation (primarily of the aortic and mitral valves), injury to the endothelium of the coronary arteries, fibrosis of the conduction system with subsequent arrhythmias or heart block, acute or chronic involvement of the pericardium, and interstitial fibrosis leading to inflammation of the myocardium [44]. This population is specifically vulnerable to chronic pericardial disease; premature coronary artery disease and atherosclerosis (primarily of the left anterior descending and right coronary arteries); cardiomyopathy, including LV systolic and diastolic function (restrictive cardiomyopathy) leading to HF; valvular disease; and conduction abnormalities as described above, which can appear years or even decades after treatment [45].
The means by which radiation produces atherosclerosis are not well understood, but it is likely that endothelial injury initiates the process [46]. The generation of reactive oxygen species and inflammation in response to endothelial injury that decreases the availability of nitric oxide may also promote atherosclerosis [47]. Myocardial dysfunction after radiation is caused by small-vessel ischemic disease and myocardial fibrosis leading to restrictive cardiomyopathy.
Risk factors for radiation-associated heart damage include: a mediastinal dose that includes the heart of greater than 30 Gy, a dose-per-fraction greater than 2 Gy, large amounts of irradiated heart, younger ages of exposure, cytotoxic chemotherapy, endocrine therapy, trastuzumab treatment, as well as the traditional risk factors for heart disease such as diabetes, hypertension, dyslipidemia, obesity, and smoking [48]. Radiation-associated atherosclerotic heart disease rarely occurs without other cardiovascular risk factors [49].
Most data regarding the late cardiac effects of radiation therapy are derived from experience with survivors of Hodgkin’s disease and breast cancer [46, 50]. Survivors of Hodgkin’s disease and breast cancer survivors treated with radiotherapy after mastectomy are among the best studied populations for radiation-associated cardiovascular disease and appear to be at high risk for radiation-associated cardiovascular disease. The relative risks range between 2.2 and 7.2 for fatal cardiovascular events post-mediastinal irradiation for Hodgkin’s disease, and 1.0–2.2 after irradiation for left-sided breast cancer. This increased risk is life-long, yet the absolute risk appears to increase with time since exposure. Radiation-associated cardiovascular toxicity may, in fact, be progressive. Hull and colleagues analyzed data from 2,232 patients of all age groups with Hodgkin’s disease. The relative risk for death from acute myocardial infarction was 3.2, showing that patients younger than 20 years of age who received high-dose radiation had the highest relative risk and that risk decreased with age at the time of radiation exposure, suggesting the importance for earlier surveillance in those exposed at young ages [46]. In another study of 1,474 Hodgkin’s disease survivors treated mostly with radiation therapy, with or without adjunct chemotherapy, hypercholesterolemia was identified as the most important independent risk factor for the late development of coronary heart disease, suggesting that aggressive modification of coronary risk factors is warranted in patients who have received mediastinal radiation therapy [51].
Although symptomatic heart disease occurs in only about 5 % of patients within 10 years after radiation treatment for Hodgkin’s disease, the more striking data has come from asymptomatic survivors [52]. Adams et al. assessed cardiovascular status in 48 survivors of Hodgkin’s disease (median time since the diagnosis of Hodgkin’s disease was 14.3 years) who had received mediastinal radiation (median 40 Gy) with no symptomatic heart disease at the time of evaluation [53]. All but one patient had cardiac abnormalities, including echocardiographic evidence of restrictive cardiomyopathy (decreased LV dimension and mass without increased LV wall thickness); 20 (42 %) had marked valvular defects; 36 (75 %) had conduction defects, including persistent tachycardia and autonomic dysfunction; and 14 (30 %) had substantially reduced peak oxygen uptake during the exercise, that could be a marker of subclinical HF [53].
Heidenreich and colleagues evaluated 294 asymptomatic patients treated with at least 35 Gy to the mediastinum for Hodgkin’s disease and found that valvular disease was common and that the incidence increased with time [54]. Compared to patients within 10 years of treatment, patients who had received radiation more than 20 years before evaluation were more likely to have mild, moderate, or severe aortic regurgitation (60 vs. 4 %), moderate or severe tricuspid regurgitation (4 vs. 0 %), and aortic stenosis (16 vs. 0 %). Mildly reduced LV fractional shortening (less than 30 %) was significantly more prevalent than in the Framingham Heart Study population (36 vs. 3 %). Furthermore, LV mass adjusted for age and sex was lower in the patients treated for Hodgkin’s disease [54]. Similar to the gradual progressive nature of anthracycline-related cardiac toxicity, which may remain latent for up to 20 years and is usually diagnosed by screening for subclinical disease, radiation-related damage may develop slowly, and patients who have various degrees of damage may remain asymptomatic for years.
Late radiation-induced pericarditis can occur months to years after mediastinal irradiation but currently has a very low incidence [53]. Most cases of radiation-induced pericarditis and pericardial effusion resolve spontaneously, usually within 12–18 months [55]. Surgery is not typically indicated for patients with occult constrictive pericarditis, and patients who have delayed pericarditis rarely have symptomatic constriction. Overall, patients with radiation-induced pericarditis have a good prognosis.
7.3 Signs and Symptoms
Early cardiotoxic effects may include electrocardiographic abnormalities, arrhythmias, and evidence of acute HF Electrocardiographic abnormalities—which include nonspecific ST segment and T-wave changes—decreased QRS voltage, and prolongation of the QT interval, may occur in up to 30 % of patients [56]. Sinus tachycardia is the most common rhythm disturbance, but tachyarrhythmias, including supraventricular, junctional, and ventricular, have been reported. Right and left bundle branch and atrioventricular block have also been described [57]. Fortunately, these electrocardiographic changes are transient and are seldom a serious clinical problem. The corrected QT interval should be monitored carefully, and if other antimicrobial, neurologic, or gastrointestinal agents that may prolong the QT interval are used, serial electrocardiograms must be obtained. A prolonged QT interval may predispose to ventricular tachyarrhythmias, such as Torsade de Pointes, a potentially life-threatening clinical condition.
Other symptoms of acute cardiotoxicity include: hypotension, hypertension, myocarditis, pericarditis, cardiac tamponade, acute myocardial infarction, acute HF, and cardiogenic shock. Sub-acute cardiotoxicity may include acute HF, pericarditis, or a fatal pericarditis-myocarditis syndrome in very sporadic cases. This rare syndrome manifests as fever, acute pancarditis (inflammation of the pericardium, myocardium, and endocardium), and symptomatic HF, and may be quickly fatal. The risk of developing early anthracycline toxicity is also high in adults, where risk factors include age over 70 years, prior mediastinal irradiation, abnormal ventricular function before therapy, a history of congestive HF or myocardial infarction, and chronic hypertension [58].
Children treated with anthracyclines or alkylating agents are also subject to acute congestive HF. Younger age at treatment is a particular risk factor. Heart failure is a progressive syndrome caused by cardiovascular and non-cardiovascular abnormalities and characterized by edema, respiratory distress, growth failure, and exercise intolerance and accompanied by circulatory, neurohormonal, and molecular derangements [59]. Symptoms of acute HF vary by age and may include tachypnea, respiratory distress, feeding intolerance, diaphoresis, tachycardia, hepatosplenomegaly, edema, ascites, jugular venous distension, cool extremities, fatigue, failure to thrive, exercise intolerance, dizziness, and syncope.
Heart failure symptoms have been classified in several ways to stratify patients by risk and to better delineate treatment strategies, though these classifications are not uniformly applied. The New York Heart Association (NYHA) classification [60], which is based on functional limitations, is not applicable to most children and infants. However, the Ross Heart Failure classification [61], a modified version of the New York Heart Association (NYHA) classification, and the New York University Pediatric Heart Failure Index (PHFI) [62] were designed to assess and grade the severity of HF in this younger population. When these three classifications were compared in children undergoing surgery for rheumatic valve disease, the PHFI correlated statistically significantly with electrocardiographic (the Cardiothoracic and Sokolov indexes) and most echocardiographic (end-systolic wall stress, left atrium to aorta diameter, LV mass) markers, and with the cardiomyopathy biomarker NT-proBNP [63]. There were no correlations between echocardiographic or biochemical markers and the Ross and NYHA scoring systems [63]. None of these classification indices have been validated as a surrogate clinical end-point in large numbers of children with HF, and have not been reported in children with cancer.
Pericardial effusions stemming from cancer and its therapies are rare but are among the most common causes of cardiac tamponade in children. The pathophysiology includes altered vascular permeability, an abrupt increase in intrapericardial fluid or bleeding associated with the inflammation of the pericardium, and lymphatic obstruction by mass effect. Under normal conditions, with inspiration, negative intrathoracic pressures increase systemic venous return to the right heart, but an even greater volume of blood is accommodated by the pulmonary vascular bed, which reduces left-sided output [64]. In cardiac tamponade, as fluid accumulates, the rise in pressure is transmitted across the myocardial wall and decreases diastolic filling, resulting in a greater reduction in cardiac output, in which case emergent pericardiocentesis (draining of pericardial effusion) is indicated, with or without surgical creation of a pericardial window.
Mediastinal radiation therapy commonly affects the pericardium and may cause acute pericarditis and tamponade [65]. More acutely, electrocardiographic changes, including T-wave abnormalities and atrial arrhythmias, have been reported in patients receiving mediastinal radiation therapy [66].
As previously mentioned, early-onset cardiotoxicity may persist or progress even after therapy is discontinued and can evolve into a chronic cardiomyopathy—which is defined as chronic progressive or late-onset cardiotoxicity, appearing years to decades after chemotherapy has been completed [10, 12].
7.4 Current Pediatric Guidelines
7.4.1 Advanced Screening and Management
Table 7.4 shows many of the common modalities used for screening and monitoring cardiotoxicity in pediatric cancer patients.
Table 7.4
Most commonly used methods for evaluating and monitoring cardiotoxicity
Method | Measure(s) of interest |
---|---|
Echocardiography | • Structure (LV wall thickness and LV internal diameter) |
• LV systolic function (fractional shortening and ejection fraction) | |
• LV diastolic function (early and atrial diastolic flow rate, E/A flow ratio, isovolumic relaxation time, strain) | |
• LV afterload (end-systolic wall thickness) | |
• LV load independent contractility (stress-velocity index) | |
Radionuclide angiography | |
Radionuclide ventriculography | • LV structure (internal diameter and volume) |
• LV function (ejection fraction and systolic and diastolic pattern of motion) | |
Electrocardiograms | • Cardiac rhythm (arrhythmias) |
• Conduction abnormalities (QT intervals) | |
Stress test (in combination with above methods) | • Pharmacologic using infusion of angiotensin II or dobutamine |
• Physiologic using treadmills and ergometer bicycles | |
Standard exercise | • Integrated results of cardiac and pulmonary function and muscle work capacity |
Endomyocardial biopsy | • Quantifies anatomic, histopathologic, cardiac toxicity |
Doppler myocardial imaging | • Velocity, strain, and strain rate |
• Myocardial function and perfusion evaluation | |
Cardiac magnetic resonance imaging | • Myocardial function and perfusion evaluation |
Blood cardiac biomarkers | |
Troponins | • Cardiac injury, myocyte death |
NT-proBNP | • LV wall stress associated with pressure and volume overload |
We have found a poor correlation between echocardiographic measurements of LV systolic performance during treatment of childhood high-risk acute lymphoblastic leukemia and the presence of dead and dying cardiomyocytes, as measured by blood cardiac troponin-T concentrations [67]. Five years after receiving chemotherapy, these same children had statistically significant correlations between the blood cardiac troponin-T concentrations measured during therapy and the echocardiographic measurements of LV structure and function measured 5 years later; however, there was no significant relation between the measures of LV systolic performance during therapy with the echocardiographic findings 5 years later [68]. This suggests that blood concentrations of cardiac troponin-T during therapy predict which survivors will have normal or abnormal LV structure and function as long-term survivors. This is not true for cardiac function measurements during therapy. This makes sense since many children who are acutely ill during active treatment for leukemia have high levels of circulating myocardial depressant cytokines that may lead to depressed LV systolic performance during therapy that is transient and not associated with dead and dying cardiomyocytes. If cardiomyocyte death or permanent impairment has occurred during therapy in some children, those children are more likely to have chronic abnormalities of LV structure and function. The same is true for abnormalities of heart rate, LV preload, and LV afterload which may be abnormal during active cancer and its treatments including chemotherapy. These abnormalities of heart rate and LV loading conditions lead to changes in LV load-dependent contractility, the intrinsic health of the cardiomyocyte, that may not reflect intrinsic LV contractility [10, 27, 69].
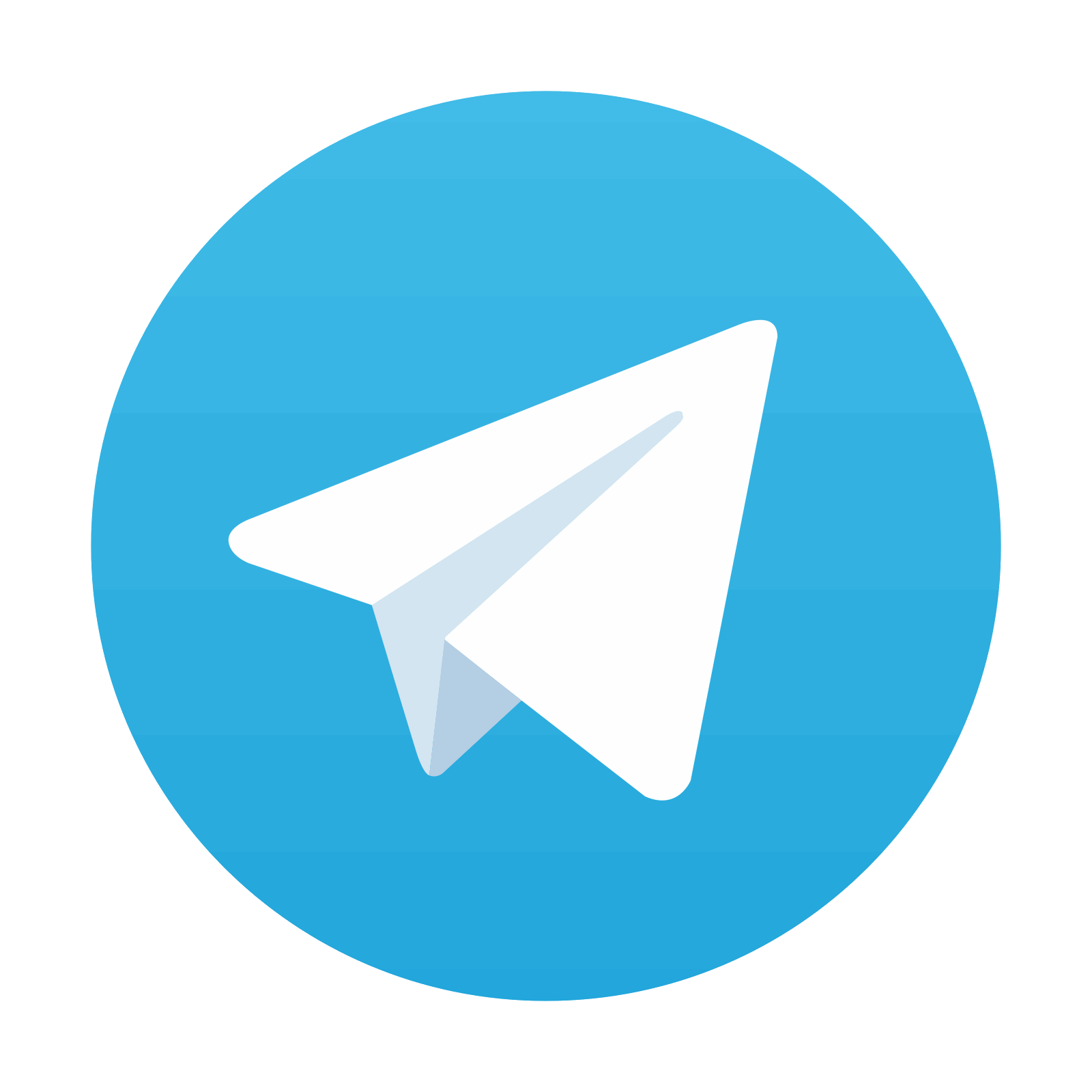
Stay updated, free articles. Join our Telegram channel

Full access? Get Clinical Tree
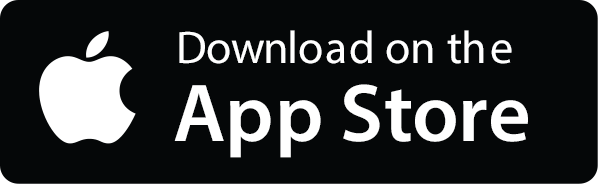
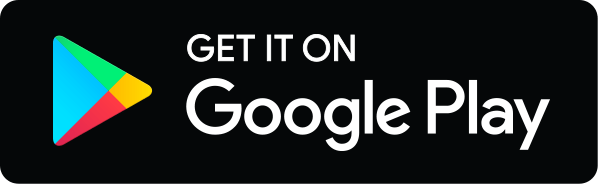