Physiologic consequence of aging
Clinical importance
Impact on treatment and care
Decreased intrinsic heart rate, maximal heart rate, and response to beta-adrenergic stimuli
Reliance on stroke volume increase and ventricular filling to augment cardiac output during stress
Utilize early aggressive fluid resuscitation for optimization of ventricular filling-
Decreased baroreceptor sensitivity
Blunted stress response to injury
Beta-adrenergic inotropes likely more useful than vasoconstrictors; afterload augmentation may be necessary
Poor tolerance of hypovolemia
Tachycardia an unpredictable response to hypovolemia
Left ventricular hypertrophy
High rates of occult diastolic/lusitropic dysfunction
Maintenance of sinus rhythm and volume repletion within a narrow range of utmost importance.
Left atrial dilatation
Increased reliance on atrial contribution to overall slowed ventricular filling
Dysrhythmias must be rapidly diagnosed and effectively treated
Right ventricular dilation
Poor tolerance of sustained tachycardia and dysrhythmias
Preserved EF poorly predictive of preserved overall cardiac function
Increased left ventricular mass to volume ratio
Predisposition for atrial fibrillation
Hypersensitivity to overaggressive fluid administration
Preserved or increased ejection fraction with overall decreased ventricular volumes and contractility
Stiffening of the central arterial tree
Systolic hypertension syndrome with increased pulse pressure, pulse wave velocity, augmentation index
Increased sensitivity of coronary perfusion to low diastolic pressures
Increased prevalence of occult or overt cardiac disease and/or cardiomyopathies
May reduce cardiac function during stress of acute injury and illness
Maintain a high index of suspicion and employ liberal use of diagnostic modalities such as ECG, ECHO, and serial troponin measurements – especially during unexplained occurrence of sudden vital sign derangement
Atypical presentation of MI
Increased sensitivity to myocardial ischemia and acute coronary syndrome during periods of increased demand
Higher rates of “silent” MI
Cardiac Output
It had previously been held that in elderly adults free of coronary disease, there was no change in cardiac output or pump function despite age-related increases in systolic blood pressure and arterial stiffness [26]. This was thought to be due to adaptations including moderate increase in LV thickness, prolonged contraction times, atrial enlargement, and increased atrial contributions to LV filling [26]. It was further postulated that prolonged contraction during systole maintained the load-bearing capacity of the heart in the face of increased afterload that came with age-related increase in arterial stiffness. Most of these data, however, came from older technology such as M-mode echocardiography and gated blood pool scans. More recently newer technology such as cardiac MRI, 2-D ECHO, 3-D ECHO, and pulsed tissue Doppler echocardiography demonstrates that these previously held theories may not be as accurate as once thought. Indeed contemporary authors have shown that echocardiography is not as efficient as cardiac MRI in distinguishing between concentric remodeling and concentric hypertrophy [27–29].
Newer studies utilizing cardiac MRI demonstrate that left ventricular hypertrophy, once thought to be adaptive, may be suboptimal. Although older myocytes do increase in size, there is overall myocyte depletion (dropout) that is associated with increased collagen deposition and nonenzymatic cross-linking [30–33]. The older ventricle, while increasing in overall mass, does not increase in overall functional mass, as demonstrated by cardiac MRI findings that reveal increasing left ventricular mass to volume ratios and associated declines in LVEDV in relation to left ventricular mass. Additionally, although the EF is preserved, absolute stroke volume is not [7]. Although LVEDV and LVESV both decrease in age, the decrease in LVEDV is proportionally greater than the decrease in LVESV, which leads to an overall age-related decline in stroke volume [7]. The phenomenon of ventricular adaptation and decreased function over time is not unique to the left ventricle. Echocardiography with pulsed tissue Doppler technology demonstrates that right ventricular systolic and diastolic function is similarly affected by an age-related decline [13]. It was previously held that elderly patients could respond to injury nearly as well as their younger counterparts based on lack of change in overall cardiac function at rest [26]. Recent findings of cardiac MRI and newer echocardiographic modalities show this to be false; however, the degree to which this affects the injured elderly patient has yet to be determined.
Baroreflex Function in Aging
Gribben and colleagues were among the first to investigate the effect of aging on baroreflex function in a study relating pulse interval to change in systolic blood pressure after phenylephrine injection. There is a linear relationship between pulse interval and change in systolic blood pressure and a distinct decrease in the baroreceptor reflex sensitivity in the elderly. Shimada and colleagues found that an age-related decline in baroreflex sensitivity is independent of systolic blood pressure and systemic noradrenaline levels [34]. Similar findings of decreased baroreceptor reflex sensitivity are found in a study of healthy volunteers examining cardiac response to angiotensin II (ANG II) infusions. The elderly, unlike their younger counterparts, do not exhibit decreases in heart rate when blood pressure is increased via ANG II infusion [35].
Beta-Adrenergic Response
Another inevitable consequence of normal aging is a decreased responsiveness to beta-adrenergic stimuli. Age-related decreases in maximal heart rate (HR max) are responsible for decreases in aerobic work capacity [7, 36, 37]. This decline is independent of gender, regular exercise, and other factors [7, 36, 37]. This decrease in chronotropic responsiveness to exercise contributes largely to age-related reduction in maximal cardiac output and is key in determining aerobic exercise capacity [37].
Although otherwise healthy, older adults exhibit decreased chronotropic responsiveness (HRmax) to exercise, the exact mechanism responsible has not been fully elucidated [37]. The elderly have decreased chronotropic and inotropic response to beta-adrenergic stimulation, and this decreased responsiveness to sympathoadrenal stimulation may explain the observed decrease in HRmax [37]. However, historical studies of B-adrenergic responsiveness consist of poorly matched heterogeneous groups and have inconsistent results. Additionally, differences in vagal tone do not seem to contribute at all to differences in HRmax in normoxic conditions. In fact, the elderly exhibit decreasing vagal tone when compared to younger counterparts [38], and incremental decreases in vagal tone accompany increased workloads during exercise. The net sum of these observations would theoretically increase the elderly patient’s HRmax, not reduce it.
Insight into B-adrenergic responsiveness and resultant decreases in HRmax can be drawn from recent work from Christou and Seals. A 2008 study of a cohort of young and elderly men examined the role of reduced intrinsic heart rate (HRint) and decreased B-adrenergic responsiveness in age-associated decreases in HRmax. Maximal heart rate was tested during treadmill exercise to exhaustion. HRint was measured at rest after complete ganglionic blockade, and B-adrenergic responsiveness was likewise tested at rest via exogenously administered isoproterenol after ganglionic blockade. This study reveals that elder men are significantly more likely to have higher serum levels of norepinephrine at rest, lower HRmax, lower HRint, and decreased chronotropic responsiveness. Both decreased HRint and decreased B-adrenergic responsiveness are strongly related to decreased HRmax; however, HRint shows a higher degree of correlation (r = 0.87 vs. r = 0.61). These authors conclude that decreased maximal heart rate observed with aging correlates with reduced B-adrenergic responsiveness but is largely explained by a lower intrinsic heart rate in healthy elderly adults [37].
Decreases in HRint are thought to be clinical manifestations of occult sinoatrial node (SA node) dysfunction, possibly related to age-related myocardial fibrosis and collagen deposition at the SA node [39]. However, SA node dysfunction does not always accompany remodeling and may in fact indicate a molecular change in the pacemaker cells [39, 40]. Recent studies have shown reductions in calcium channel proteins which may lead to decreased sinus node depolarization reserve and thus suppression of action potential formation and propagation [41]. Additionally, connexin [42], a primary gap junction protein, is reduced in aged cells [42]. This reduction is hypothesized to restrict conduction of SA node action potentials within the myocardium.
Effects of Aging on Cardiovascular Performance After Injury
Although there is very little information regarding the impact of aging on the cardiovascular response to injurious stress, much is known of the cardiovascular response to exercise. Much of our understanding of the cardiovascular response to injury is extrapolated from these data.
The cardiac output response in the elderly during exercise is significantly reduced. However, subsequent studies excluding patients with coronary artery and cardiac disease show no significant age-related change in cardiac output during exercise. Cardiac output modulation is affected by age. Older patients cannot increase cardiac output with increases in heart rate due to decreases in HRint and B-adrenergic responsiveness. The elderly utilize the Frank-Starling mechanism and increase their end-diastolic volume and stroke volume during exercise, thereby increasing cardiac output without substantially increasing heart rate. The elderly do augment their stroke volume during exercise; however, the increase in ejection fraction is less than that observed in younger counterparts secondary to a decreased ability to reduce end-systolic volume. This is similar to what is seen in young patients given exogenous beta-blockade and then stressed with increasing exercise loads. In this scenario, the young similarly increase cardiac output by increasing stroke volume without appreciably increasing heart rate. Interestingly, the elderly have increased plasma levels of epinephrine and norepinephrine during exercise loading. This finding further underscores the elderly patient’s lack of beta-adrenergic responsiveness.
Severe injury often leads to profound states of hypovolemia through blood loss and capillary leak. Critically ill elderly patients are also susceptible to further intravascular depletion from fever (free water loss), poor intake, pharmacologic vasodilation (home medications), and decreased plasma oncotic pressure (poor nutrition). Given the elderly dependence on the Frank-Starling modulation of cardiac output rather than chronotropy, the elderly patient is particularly sensitive to preload reductions secondary to hypovolemia. This is evident in work done by Shannon and colleagues in which elderly patients mount a blood pressure increase and slight HR increase similar to younger patients in tilt test. However, when the same test is performed subsequent to preload reduction with diuretics, elderly patients sustain a symptomatic fall in blood pressure due to an inability to mount a tachycardic response, whereas younger patients exhibit a profound increase in both heart rate and blood pressure [43].
Therefore, based on exercise data, elderly patients are capable of maintaining cardiac output in response to the stress of injury. This is not by heart rate augmentation but rather by effecting stroke volume by increasing end-diastolic volumes and contractility. Research from the early 1980s suggests that elderly patients were unable to mount a cardiac output response to stress. However, subsequent studies of carefully screened healthy elderly patients demonstrate an absence of significant changes in age-related cardiac output variables during exercise in the elderly [6, 62]. Newer technology including cardiac MRI pulsed tissue Doppler echocardiography in 2D and 3D demonstrates that while elderly patients can mount a cardiac output response to stress, this may be much less in magnitude than their younger counterparts due to decreased cardiac reserve [13, 25, 44, 45].
In a study of critically ill trauma patients, Belzberg and colleagues document age-related differences in cardiovascular response to injury [46]. In their 2007 study, they utilized noninvasive primarily transcutaneous devices to collect hemodynamic and oxygen transport variables on 625 consecutive trauma patients at the time of emergency department (ED) admission. Of these, 259 were deemed “high risk” and had pulmonary artery catheters placed for invasive hemodynamic monitoring. Hemodynamic patterns for elderly (>65) and non-elderly (<65) patients were analyzed. Data were stratified by survivors and non-survivors and then further divided by age (elderly vs. non-elderly survivors). Elderly trauma patients had significantly lower CI, HR, arterial oxyhemoglobin saturations (SpO2), hematocrit (Hct), and oxygen delivery. Interestingly, while elderly survivors have significantly lower values of these parameters than young survivors, elderly and young non-survivors exhibit very similar hemodynamic and oxygen transport values. This study concludes that aggressive resuscitation titrated to hemodynamic and oxygen transport variables may improve outcomes and that the cardiac response to injury seen in elderly patients is much less robust than that of their younger counterparts [46]. It is obvious that elderly patients generate increased cardiac performance in the face of the stress such as surgery and injury; however, the magnitude of this response is attenuated and less robust than that of younger counterparts. It is unknown whether this is due to normal age-related decline in cardiovascular function or to an increased prevalence of cardiovascular disease. Extrapolation from exercise data suggests that it is a combination of both.
Post-injury Myocardial Depression
Real-time assessment of global cardiovascular function using dense data capture technology and bedside assessment of ventricular-arterial coupling demonstrates the occurrence of myocardial depression in the critically injured and posttraumatic septic shock patient [47]. Prior work by Tacchino and Siegel describes similar phenomena in multiply injured patients [5]. The authors reported a 32 % incidence of myocardial depression, as evidenced by a reduction in cardiac index (CI) and ejection fraction (EF), in patients 65 years and older, and a 21 % incidence in those 13–30 years of age. Furthermore, mortality rate rises to 60 % in the elderly group when myocardial depression occurs.
Cardiac Performance in Aging and Disease: Effect of Comorbidities on the Cardiovascular Response to Injury
Atrial Fibrillation
In critically injured elderly patients, atrial fibrillation (AF) is particularly troublesome because age-related changes in pump function and cardiac output rely heavily on prolonged contraction times and increased atrial contribution (“atrial kick”) for adequate left ventricular filling. Age-related increases in left (and right) atrial size in healthy older patients are a risk factor for the development of atrial fibrillation [48]. Additional age-related risk factors include inflammatory cytokines, local and systemic stress, altered calcium handling, and electrical remodeling [49]. In the acute setting, pulsatile mechanical atrial stretch and inflammatory cytokines (from injury, sepsis, or ischemia) contribute to arrhythmogenesis [49]. Numerous cytokines may contribute to the development of AF. Postoperatively, elevated interleukin 6, interleukin 8, and hsCRP levels are highly correlated with AF [49]. Interestingly, these same cytokines are present in high levels in the serum of injured patients and can be used to predict progression to multiple organ dysfunction and failure in the multiply injured patient [50, 51]. In addition to inflammatory cytokines, injured patients are exposed to other risk factors that predispose to atrial fibrillation: large volume resuscitation causing increased atrial stretch, increased endogenous catecholamine release, rapid fluid and electrolyte shifts, hypoxia, hypercarbia, pulmonary artery catheter placement, as well as specific injury patterns [52, 53]. Another risk factor is withdrawal from chronic beta-blockade in the elderly [54].
Although this dysrhythmia is common in critically ill and injured patients, its exact pathophysiology in injured patients is unknown. Although the occurrence of AF after blunt thoracic injury with cardiac contusion is well described [55, 56], until recently there has been sparse data regarding atrial fibrillation in the injured patient. A 2011 study has given some insight into AF in trauma patients. In a retrospective review of trauma patients admitted to a large level one trauma center, AF occurred in 6 % of patients and was an independent risk factor for mortality. The sole risk factor for AF development in these patients was age greater than 55. Interestingly, there is a mortality benefit if these patients were “exposed” to beta-blockers sometime during their hospital stay. Unfortunately, the exact dosages and duration have yet to be defined. There is currently not a superior algorithm for treatment of AF in the elderly. AF should be treated according to current ACLS protocols with preference of beta-blockade for treatment if efficacious. AF should be diagnosed and managed expeditiously in the elderly due to greater expected reductions in cardiac output secondary to loss of atrial kick and need for longer diastolic filling times.
Ischemic Heart Disease
Injured patients are uniquely at risk for acute myocardial ischemia given that trauma and critical illness are associated with endogenous catecholamine release, systemic inflammation, and increased myocardial oxygen demand. Additionally, hyperdynamic blood flow during acute resuscitation and its resultant turbulent and non-laminar blood flow increase shear forces. This increased shear may theoretically confer instability on coronary and arterial atherosclerotic plaques and predispose to myocardial infarction (MI) [57]. This is further compounded in the elderly in whom arterial pulse wave indices do not favor diastolic filling of coronary vessels and in whom arterial stiffening only exacerbates conditions of turbulent arterial blood flow. Elderly patients are additionally at risk from age-related coronary artery disease and preexisting cardiac disease. MI represents an important disease entity to address as it is associated with poorer outcomes especially in the aged [58–60].
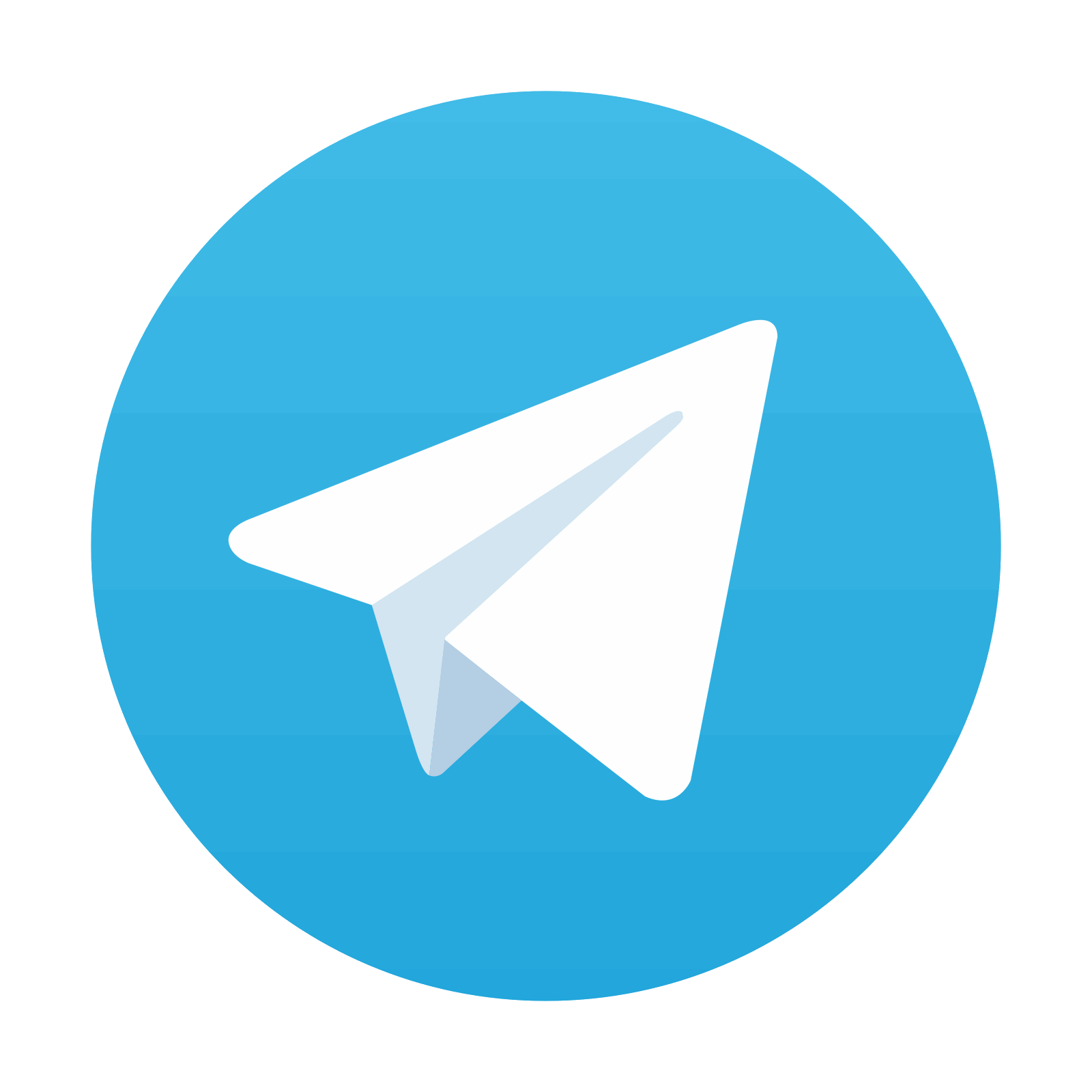
Stay updated, free articles. Join our Telegram channel

Full access? Get Clinical Tree
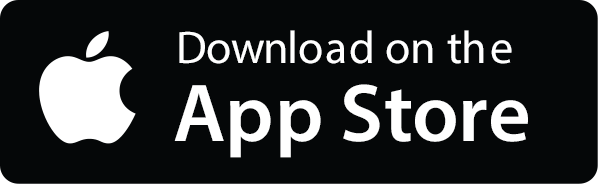
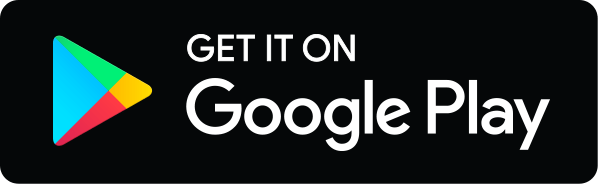