Atherosclerosis
Cardiovascular disease
Heterozygous familial hypercholesterolemia
Hyperlipidemia
Risk prevention
Triglycerides

Classification of lipoproteins: Five major classes of lipoproteins act as transport systems for cholesterol and triglycerides. They differ in physical and chemical characteristics and function, as well as in amounts of cholesterol, triglyceride, phospholipid, and protein. The lipoproteins can be separated by ultracentrifugation or electrophoresis, on the basis of differences in densities and surface properties. Ultracentrifugation yields chylomicrons, very-low-density lipoprotein (VLDL), low-density lipoproteins (LDLs), and high-density lipoproteins (HDLs).
Estimation of lipoproteins: Non-HDL Cholesterol (non-HDL-C) represents the cholesterol content of all plasma lipoproteins and may soon replace LDL-C in risk assessment, particularly when non fasting lipids are used. NonHDL-C
is calculated as follows: Total cholesterol minus HDL-C = nonHDL-C.
The calculation of the proportion of LDL is made with the following formula:
Total cholesterol = LDL + HDL + VLDL
HDL is measured directly, and VLDL is estimated by dividing the fasting triglyceride concentration by 5 (true, so long as the triglyceride concentration is <400 mg/dL). Therefore,
LDL = Total cholesterol – HDL – (triglycerides/5)
Each lipoprotein has a characteristic apolipoprotein profile. These apolipoproteins serve as cofactors for enzymes involved in lipoprotein metabolism, they help in the binding of lipoproteins to cellular receptors, and they facilitate lipid transfer between lipoproteins. Apolipoprotein B-100 (apoB-100) is an important component of VLDL and is the only apolipoprotein in LDL cholesterol (LDL-C). Uptake of LDL by cells is dependent on its binding to the LDL receptor, which is regulated by apoB-100. Abnormalities in both quality and quantity of these proteins, even in the absence of an elevated cholesterol concentration, may contribute to atherosclerosis.
Lipoprotein circulation and sources: (Fig. 14.2)
Exogenous: Chylomicrons are formed after absorption of dietary fat. They are secreted into the lymph. Fatty acids are stored in adipose tissue, or are used in skeletal muscle and myocardium, where they release diet-derived triglycerides. This reaction is catalyzed by lipoprotein lipase. The chylomicron remnants are rapidly absorbed in the liver by specific receptors for these particles. In liver cells, the remnants are degraded to free cholesterol, which is excreted into bile.
Endogenous: The endogenous transport system includes VLDL, intermediate-density lipoprotein (IDL), LDL, and HDL. Excess calories from carbohydrates and fatty acids are metabolized in the liver into triglycerides. The lipoproteins carrying these triglycerides are primarily VLDL, which moves to adipose tissue; the result is the formation of IDL and LDL. The IDL particles are rapidly removed from circulation by LDL receptors in the liver.
LDL transports cholesterol to peripheral tissues. In addition to the lipid component, LDL particles contain a single apoB-100 molecule, the protein that binds to LDL receptors. After binding to LDL cell-surface receptors, the LDL particles deliver cholesterol for synthesis of cell membranes in all cells; for steroid hormones in the adrenal glands, ovary, and testes, and for bile acids in the liver. The LDL-C found in macrophages and smooth muscle cells of atherosclerotic lesions enters by additional mechanisms. This LDL-C is modified by intravascular oxidation and is taken up in lesions by oxy-LDL receptors and scavenger receptors. This process may provide alternative pathways for therapeutic intervention.
HDL is secreted from the liver or intestine in a lipid-poor form or is made de novo in the plasma. As it matures, HDL accumulates cholesterol from tissues, including blood vessel walls, and therefore has a major role in removing excess cholesterol and delivering it to the liver by means of the triglyceride-rich lipoproteins and cholesterol ester transfer protein.

Epidemiological evidence:
In populations throughout the world, there is a direct correlation between serum cholesterol levels and CVD rates. The prevalence of hyperlipidemia has been well documented.9 Traditional risk factor assessment has focused on parameters derived from the Bogalusa10,11 and Framingham Heart Study12 (age, hypertension, cholesterol, family history, and cigarette smoking). New emerging risk factors, both biological and genetic, are reshaping the understanding of heart disease and the approach to risk stratification. The Pathobiological Determinants of Atherosclerosis in Youth Study described the relationship between atherosclerosis and serum lipoprotein cholesterol concentrations and smoking in AYA males.13
Genetic evidence (characteristics of inherited hyperlipoproteinemias are listed in Table 14.1):
Familial hypercholesterolemia (FH): In its most common form, FH is an autosomal dominant condition resulting in defects in the LDL receptor and elevated levels of LDL-C. About 1 in 300 to 500 AYAs in the US is heterozygous for the abnormality; these individuals account for 15% of cases of premature CVD. Homozygous individuals (˜1 in 1,000,000) lack LDL cell-surface receptor activity, have very high cholesterol levels, and may develop severe atherosclerosis in the first two decades of life. Clinical manifestations such as xanthomas and other signs of cutaneous lipid deposition are generally seen in the fourth decade of life in heterozygotes and during adolescence in homozygotes (Fig. 14.3).14
Familial combined hyperlipidemia (FCHL): It is the autosomal dominant syndrome that affects approximately 1% to 2% of the population. Most, if not all, patients with this condition have elevated levels of LDL apoB. Abnormal metabolism of VLDL and partial lipoprotein lipase deficiency have also been described in association with this syndrome. Individuals with FCHL account for a significant proportion of cases of early-onset CVD.
Apolipoprotein E (apoE)15: Three common alleles of apoE, at a single-gene locus on chromosome 19, code for three isoforms of apoE: designated as apoE-II, apoE-III, and apoE-IV. Increased cardiovascular risk is associated with apoE-IV, in comparison with the more common apoE-III.
Novel biomarkers:
High-sensitivity C-reactive protein (hs-CRP) assays: Inflammation is an important risk factor for atherogenesis. C-reactive protein (CRP), the most studied biomarker of inflammation in CVD, is secreted by foam cells in the arterial wall. CRP is implicated in the development of CVD through its actions: induction of a prothrombotic state, increasing leukocyte adhesion, and promotion of intimal damage. CRP has been shown to be a lipid-independent risk factor for CVD. CRP assays have traditionally been used to detect inflammation and/or infection. Hs-CRP assays detect low levels of CRP and may have utility in predicting a healthy person’s risk of CVD. While guidelines have been proposed for the use of hs-CRP in asymptomatic adults at intermediate risk of CVD, there are currently no clear guidelines for the use of hs-CRP assays to predict CVD risk among AYAs.
Apolipoprotein-associated phospholipase A2 (Lp-PLA2)16: The enzyme Lp-PLA2 is a member of the phospholipase A family. It is actively expressed in unstable atherosclerotic plaques, suggesting its role in increasing plaque vulnerability. Lp-PLA2 is both less variable than CRP and a more specific marker of vascular inflammation.
Myeloperoxidase (MPO) and oxidized LDL17: Products of the MPO pathway may be good markers of CVD, and possibly therapeutic targets as well.
Interventional trials: Interventional trials support the conclusion that lowering total and LDL-C concentrations reduces the incidence of CVD events. The degree of benefit is greatest in individuals who have other associated risk factors, such as cigarette smoking, diabetes, and hypertension.
Relationship of particular lipoproteins to cardiovascular risk:
LDL-C: Studies show a positive relationship between the level of cholesterol, and the frequency of CVD.
HDL-C: Population studies suggest an inverse relation between HDL-C and CVD. An HDL-C level of <30 mg/dL carries a significantly increased risk of CVD. A level >50 mg/dL is associated with low risk, and >75 mg/dL is associated with very low risk. The Framingham Heart Study demonstrated a 10% increase in CVD for each 4 mg/dL decrease
in HDL. In addition, low HDL-C levels have been correlated with an increased number of diseased coronary arteries. There also appears to be a higher rate of restenosis after angioplasty in individuals with low HDL-C levels. HDL has two components, HDL2 and HDL3. The former is considered a better indicator of negative CVD risk than total HDL. Exercise raises the level of cardioprotective HDL2, whereas ethanol raises the level of HDL3. Unfortunately, raising HDL-C is not currently a therapeutic option. Pharmaceutical trials have so far failed to show reduction in CVD and some have been discontinued because of higher CVD in drug-treated than placebo groups.
TABLE 14.1 Characteristics of Inherited Hyperlipoproteinemias
Hyperlipoproteinemia
Phenotype
Cholesterol
Triglyceride
Xanthomas
Frequency (%)
Risk of CVD
Familial lipoprotein lipase deficiency
I
Normal
↑
Eruptive
Very rare
0
Familial hypercholesterolemia
IIa
↑
↑
Tendon
0.1-0.5
4+
IIb
Tuberous xanthelasma
Polygenic hypercholesterolemia
II
↑
Normal
Tuberous
5
2+
Familial dysbetalipoproteinemia
III
↑
↑
Palmar
Rare
4+
Planar tuberous tendon
Familial combined hyperlipoproteinemia
IIa
↑
↑
Any type
1-2
3+
IIb
IV
Rarely V
Familial hypertriglyceridemia
IV
Normal
↑
Eruptive
1
1+
Rarely V
CVD, coronary artery disease; NL, normal.
From Arky RA, Perlman AJ. Hyperlipoproteinemia. In: Rubenstein E, Federman DD, eds. Scientific American medicine. New York, NY: Scientific American, 1988, with permission.
Apolipoproteins: Apolipoproteins A-I (apoA-I), A-II (apoA-II), and apoB are used in predicting the risk of CVD. Elevated concentrations of apoA-I and apoA-II are associated with a lower risk, and an elevated apoB concentration is associated with a higher risk of CVD. Isoforms of apoE have also been implicated in cardiovascular risk, as noted previously. In addition, the measurement of the apoB-100 to apoA-I ratio may provide another assessment of cardiovascular risk.
Ratios: CVD risk rises sharply when the LDL:HDL ratio exceeds 3.0. Another ratio predictive of CVD risk is that of total cholesterol to HDL-C. A ratio of <4.5 denotes below-average risk, whereas the optimum ratio is 3.5:1. The AHA recommends that the absolute numbers for total blood cholesterol, LDL-C, HDL-C, and non-HDL-C be used. The AHA suggests that these numbers are more useful than ratios to the physician determining appropriate treatment for patients.
Other experimental work: Proprotein convertase subtilisin/kinexin type 9 (PCSK9)16: PCSK9 is a serine protease. It binds the low-density lipoprotein receptor (LDL-R) in circulation and promotes LDL-R degradation. A missense mutation in PCSK9 leads to higher circulating LDL. Several, new therapeutic options targeting PCSK9, including monoclonal antibody-based therapies, have entered trials with promising results in subjects with both familial and nonfamilial forms of hypercholesterolemia.

TABLE 14.2 Metabolic Classification of Dyslipoproteinemia in Children and Adolescents | |||||||
---|---|---|---|---|---|---|---|
|

Table 14.4 lists elements of the family history and risk factors.
Table 14.5 lists special risk conditions that confer high- and moderate risk for CVD in the Integrated Guidelines for Cardiovascular Health and Risk Reduction in Children and Adolescents.
Table 14.6 lists major risk factors that modify LDL-C goals in the ATP III guidelines.
TABLE 14.3 Screening Elements for Cardiovascular Risk Assessment | ||||||||||
---|---|---|---|---|---|---|---|---|---|---|
|
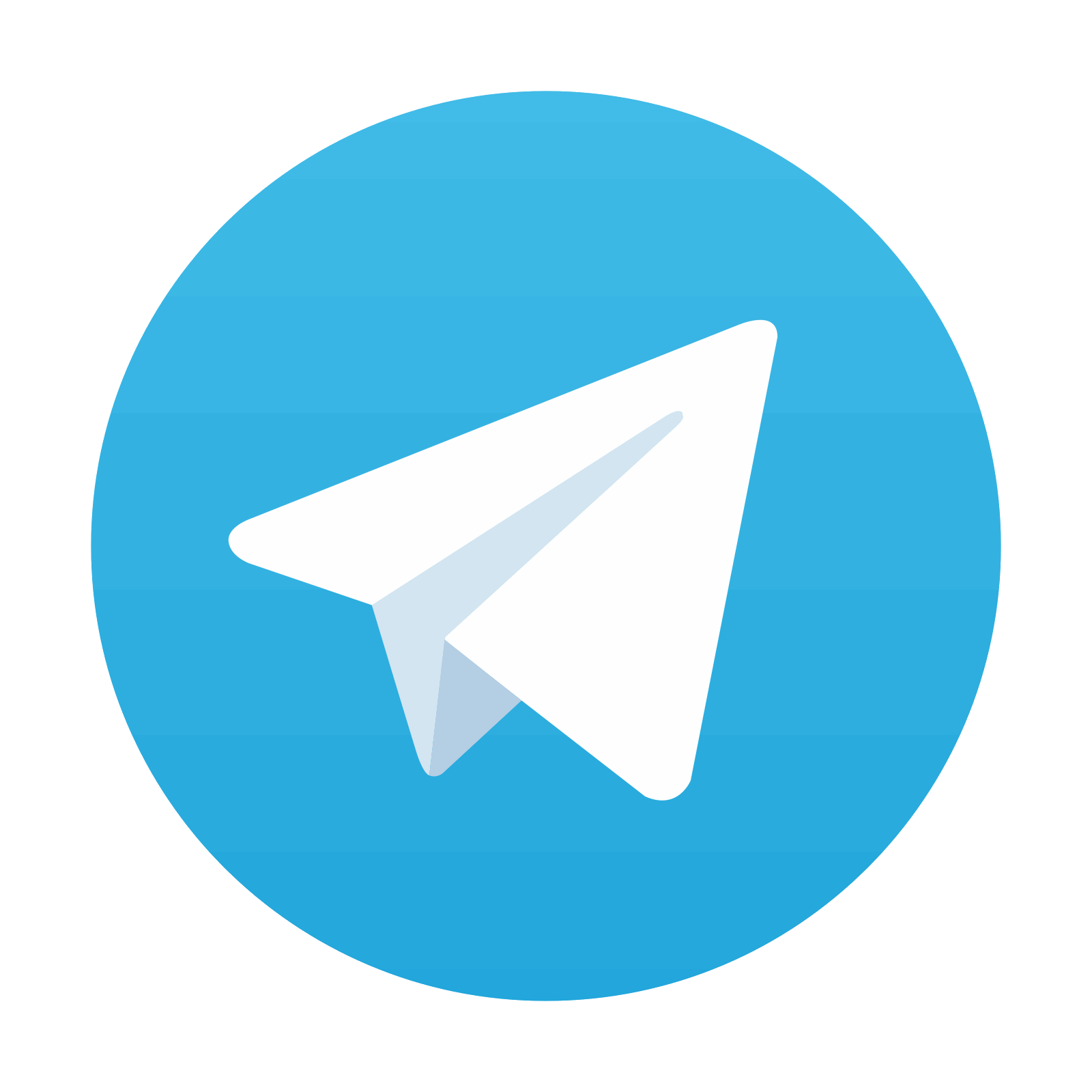
Stay updated, free articles. Join our Telegram channel

Full access? Get Clinical Tree
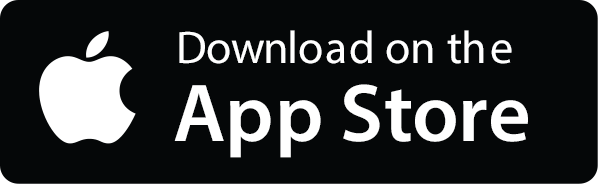
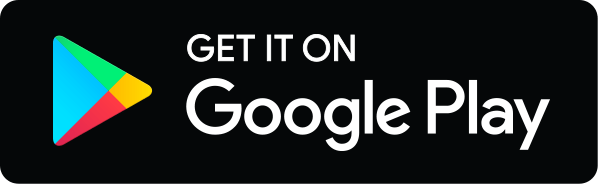