Fig. 8.1
Main signaling pathways involved in the molecular mechanisms underlying CSC control. The activation of these pathways following different stimuli results in the enhanced transcription of several genes (i.e., cyclins, c-Myc, EGF, VEGF) involved in physiological cell processes, including cell proliferation, growth, and survival. FZD Frizzled (Wnt receptor), γ-secretase enzyme responsible of the release of the Notch intracellular domain, SHh Sonic Hedgehog Homolog, DHh Desert Hedgehog Homolog, IHh Indian Hedgehog Homolog, PTCH1 Patched 1 (Hedgehog receptor), Smo smoothened, Gli Gli transcription factors, PI3K phosphatidylinositol 3-kinase, Akt also known as Protein Kinase B (PKB), mTOR mammalian target of rapamycin, JAK Janus kinase, STAT signal transducer and activator of transcription, NF-kB nuclear factor-kappa-light-chain-enhancer of activated B cells, IkB inhibitor of kB, IKK IkB kinase (responsible of IkB degradation)
8.2.5.1 Wnt/β-Catenin
The Wnt/β-catenin signaling pathway is a pivotal developmental pathway [76], reported to control proliferation vs. differentiation in normal stem cell maintenance and growth [2, 77–80]. Aberrant Wnt activation is a key factor for the initiation and progression of various tumors [81–83]. Furthermore, increasing evidence suggests the involvement of Wnt signaling in the molecular mechanisms underlying CSC control. It has been reported that Wnt pathway triggers a response to DNA damage [84] and that genomic instability may drive the malignant transformation of nontumorigenic stem cells to CSCs [85–87].
Wnt pathway-related genes such as FZD6 and WNT7B are highly expressed in undifferentiated mouse mammary tumor cells that are grown in mammosphere to enrich for progenitor-like cells, compared with the differentiated population [88].
The importance of Wnt signaling pathway in CSC control has been strengthened by Vermeulen et al. [89], who demonstrated that Wnt signaling activation was a marker for colon CSCs. In addition, a role for Wnt pathway in cutaneous CSCs has been highlighted which appeared enriched for Wnt signaling: ablation of the β-catenin gene resulted in the loss of CSCs and complete tumor regression in a model of squamous cell carcinoma [90].
Connections between Wnt signaling and epithelial–mesenchymal transition (EMT)—the process by which cells acquire a mesenchymal identity, losing cell–cell adhesion properties and polarity [91]—have also been suggested in numerous studies, but the exact role of Wnt pathway in promotion or reversal of EMT is still unclear.
8.2.5.2 Notch
The Notch family regroups four single-pass transmembrane protein receptors involved in cell development [92]: aberrant expression and dysregulation of Notch proteins, ligands, and targets has been described in hematological malignancies [93] and in a multitude of solid tumors [93, 94].
Notch is activated via γ-secretase-mediated cleavage, which releases the intracellular domain from the membrane, allowing it to translocate into the nucleus, where it forms a short-lived transcription complex.
Crosstalk between Notch and other oncogenic pathways has been described to exert a mutual regulation on TGF-α [95, 96], VEGF [97], Wnt [98], and PEA3 [99] pathways. In recent years, Notch activity has been reported to be implicated in the maintenance of CSCs in various cancers [100–108]. The Notch pathway is an important factor in the linkage between angiogenesis and CSC self-renewal; thus, Notch pathway targeting is increasingly considered a therapeutic strategy for cancer treatment, by eliminating CSCs [109]. Accordingly, selective blockage of Notch reduced self-replication and tumor formation capacity of leukemic CSCs [110] and impaired mammosphere formation in vitro [111]. Moreover, the inhibition of Notch pathway in association with trastuzumab has been proven effective in preventing tumor relapse in ErbB2-positive xenograft murine model of breast cancer [112]. Additionally, accumulated evidence has demonstrated that Notch signaling might contribute to cancer metastasis [113].
8.2.5.3 Hedgehog
The hedgehog (Hh) signaling pathway coordinates development of tissue progenitors and expansion of stem cells [114]. Pathway activation is initiated by binding of one of the three ligands, Sonic (SHh), Desert (DHh), and Indian Hedgehog (IHh), to the patched receptor (PTCH1), disabling its constitutive repression of smoothened (Smo), that leads to activation of the Gli transcription factors [115] and, hence, to the enhanced transcription of several genes involved in cell proliferation, such as cyclins, c-Myc, epidermal growth factor (EGF), and vascular endothelial growth factor (VEGF) [116].
Aberrant Hh signaling has been described both in individuals affected by predisposing genetic syndromes [117] and in tumors displaying sporadic mutations involving PTCH loss [118]. Emerging data from many human tumors suggest that Hh is required to maintain self-renewal, proliferation, and tumorigenic potential of CSCs in a complex fashion involving both intracellular signalization and interaction with differentiated tumor cells and with the microenvironment [119–121].
Hh signaling has been found to be preferentially activated in clinical specimen-derived colon [122] and breast [116] CSCs, as evidenced by the increased expression of GLI1, GLI2, and PTCH1.
8.2.5.4 mTOR
The phosphatidylinositol 3-kinase (PI3K)/Akt/mammalian target of rapamycin (mTOR) signaling pathway is a key regulator of physiological cell processes, including growth, cell proliferation, and survival [123–125]. mTOR signaling aberrant activation is frequently observed in human cancers [126]. Recent studies showed that mTOR pathway may be involved in the regulation of CSC biology, notably cell cycle progression and survival [123, 127].
Furthermore, Akt1/2 proteins were more expressed in mammosphere cells than in more differentiated cells [116]. Akt downregulates glycogen synthase kinase-3β (GSK-3β), thus enhancing β-catenin-induced CSC self-renewal. Hence, inhibition of the Akt signaling pathway could be an effective tool to reduce CSCs [128].
8.2.5.5 STAT and NF-kB
Several studies have demonstrated that chronic inflammation is a key factor in initiation and progression of various cancers [129–131].
Pivotal molecular links between inflammation and cancer are the signaling pathways of signal transducer activator of transcription (STAT) and nuclear factor (NF)-κB.
STAT and NF-κB are crucial transcriptional regulators of activation of genes associated with cell proliferation, angiogenesis, metastasis, and suppression of apoptosis [132–134].
TNF-α activates NF-κB by phosphorylation of the inhibitor IkB by IKK. After the dissociation of the inhibitor IkB, free NF-κB migrates into the nucleus and activates the expression of downstream genes, some of which are antiapoptotic.
Recent studies demonstrated that IL-6/JAK2/STAT3 pathway is required for the maintenance of CD44+CD24− CSCs in breast cancer [135] and that ovarian CSCs are characterized by constitutive activation of NF-κB [136]. Therefore, these pathways have been suggested as potential therapeutic targets in cancer stromal cells, in tumor cells, and also in CSCs [137–139].
Accordingly, it has been reported that STAT3 inhibition disrupts proliferation and maintenance of glioblastoma stem cells [140, 141], reduces CD133+ALDH+ colonic CSCs—thus affecting colonosphere formation [142]—and reduces the frequency of ALDH+ CSCs in prostate cancer [143]. Similarly, inhibition of the NF-κB pathway results in CSC apoptosis and induces cell death in the chemoresistant ovarian CSCs [139].
Actually, the identification of signaling pathways is an active area of investigation, but at present, none of these pathways showed a CSC-specific activity.
Although combinations of these different markers have improved reliability to some extent, their limited specificity is a major obstacle to a definitive validation: advances in transcriptional and proteomic profiling could be helpful to provide more reliable tools to identify CSCs [144].
8.3 A Dynamic Cancer Stem Cell Concept
At present, CSCs are suggested to be those cells, usually sorted by flow cytometry on the basis of the expression of a particular cell surface marker, that have the tumorigenic ability to form a new tumor in an in vivo xenograft assay and/or ability to form cell spheres when plated at low density in nonadherent culture [145]. Despite this broad definition, it is still hard to define CSCs as a single universal entity, suggesting that the CSC phenotype may vary substantially across different tumors and, more interestingly, could not fit to a steady definition across the natural history of cancer. Experimental observations led to the hypothesis that “stemness” may not be a fixed “immutable” property, but rather a more dynamic condition, with cells evolving to more or less differentiated states [146–148]. Indeed, it has been suggested that CSCs can undergo reversible fluctuations in their stem cell status [149–151]. These findings account for the possibility that cancer stemness can be acquired by modification in the gene expression programs. Although the molecular pathways underlying these effects are largely unknown, the concept of phenotypic reversion suggests the presence of a balance between CSCs and differentiated cells. Moreover, in recent years, multiple studies confirmed the presence of an important degree of intra-tumor heterogeneity, with several clones coexisting in the same tumor mass [152], resulting from genetic instability. Two major, not mutually exclusive, mechanisms explain intratumoral heterogeneity [19]:
1
Different genetic or epigenetic mutations occurring within the same target
These observations turn our traditional view of the CSC model from a “hierarchical” structure (a cancer stem cell on the top of a differentiated tumor cell population) to an “oligarchic” structure with multiple genetically different cell lineages competing with each other [27], in particular in advanced stage disease where cancer therapy exerts a powerful selective pressure on cancer cells.
8.4 The CSCs Niche
Normal stem cells (SCs) are located in a specialized microenvironment called “stem cell niche” that provides SCs with molecular signals necessary to maintain them in a stem-like state and to reach the right balance between self-renewal, activation, and differentiation [154–156].
Emerging evidences suggest that CSCs are also regulated by a similar microenvironment [145]; therefore, tumorigenicity likely depends not only on the biology of tumor cells but also on the cross talk between tumor cells and the neighboring nonmalignant cells [157]. Such an intimate connection between CSCs and the surrounding microenvironment is supported by the observation that CSCs localize to the tumor–stroma interface in different cancers [158, 159].
The CSC niche is composed of several stromal cell populations including mesenchymal, vascular, and immune cells and myofibroblasts, as well as extracellular components [160–162].
Actually, the presence of three different niches in tumor tissue has been hypothesized: a perivascular niche, a hypoxic niche, and a niche at the invasion front.
The perivascular niche has been described as a microanatomical unit composed of proliferating endothelium and other stromal cells (i.e., pericytes and macrophages) and of extracellular components [163–166], closely interacting with cancer cells.
The existence of a hypoxic niche has been suggested since recent studies showed that stem cells are also located in the necrotic areas of the tumor [167] and that they are regulated by local oxygen concentrations [168]. Lastly, the observation that cancer cells undergoing EMT at the invasion front exhibit some of the phenotypic characteristics typical of CSCs (i.e., marker expression or microRNA) [169, 170] has raised the possibility of an invasive niche.
8.4.1 Functions
It is now well accepted that niche cells are not malignant per se but that the CSC niche supports cancer growth and has an essential role for tumor survival [171].
As already mentioned, the microenvironment is a crucial component that controls CSC functional features, such as stemness, proliferation, and apoptosis resistance [156, 172, 173]. In fact, it has been observed in several tumors that CSCs rely on niche signals to remain in their stem-like state, while maintaining their self-renewal properties and their capacity to originate differentiated cells [165, 172].
The importance of the interaction between CSCs and their microenvironment is confirmed by the observation that the loss of a niche usually leads to the loss of the CSC pool [156].
Several factors are involved in the cross talk between CSCs and niche microenvironment. For instance, the key role of CD44 [174, 175], CXCR4 (expressed by tumor cells), and CXCL12 (SDF-1, expressed by niche stromal cells) [76, 145] for the homing and engraftment of CSCs to the niche and the importance of CD133 for the CSC selective adhesion to the endothelial cells of perivascular niche [165] have been demonstrated. Moreover, endothelial cells of the vascular niche have been shown to stimulate CSC stemness in part through Notch signaling [109, 165, 176].
However, the observation that CSCs promote blood vessel formation by secreting VEGF has raised the hypothesis that the cross talk between CSCs and tumor vasculature could probably be a bidirectional process [177].
It has also been observed that in colon CSCs, Wnt signaling activity is increased by stromal fibroblasts, through secretion of hepatocyte growth factor (HGF) [89]. Furthermore, the niche microenvironment has been reported to support CSCs in different tumors through the release of inflammatory molecules, such as interleukin (IL)-6 [178, 179]. Also physiologic conditions existing within niches, such as hypoxia and low pH, are critical determinants in the maintenance of the CSC pool [180, 181]. Indeed, the hypoxia-inducible factor 2-α (HIF2α), the main mediator of hypoxia effects, promotes self-renewal and tumorigenicity of glioma CSCs [180]. It has yet to be fully elucidated whether these factors are really essential for the maintenance of CSC homeostasis; however, recent data seem to demonstrate that their loss reduces the clonogenicity of CSCs in vivo [165, 182]. Although a major role of the niche in CSC self-renewal has been suggested, clues from microenvironment may be sometimes negative for CSCs. Indeed, tumor growth can be inhibited by immune-mediated signals [50] or by differentiated tumor cells after xenotransplantation [10]; the latter observation leads to the interesting hypothesis that differentiated tumor cells control CSC spread.
Despite the similarities between normal SC and CSC niche, the microenvironment seems to regulate CSC behavior in a way more favorable to cell proliferation and growth as compared to normal SCs. In fact, while normal SC proliferation and differentiation are usually inhibited by the niche and tissue regeneration can be activated only after a transient proliferating signal, it is believed that internal mutations and niche signals can lead CSCs to proliferate without control [183].
Interestingly, the niche not only plays a pivotal role in the preservation of the CSC population and tumor growth enhancement but also has the ability to induce a CSC phenotype in more differentiated tumor cells [89, 156].
8.4.2 CSC Niche as Therapeutic Target
Given the fundamental role of microenvironment in supporting tumor growth and in affecting CSC response to therapy, the CSC niche, together with the factors involved in niche–CSC interaction, has become an attractive therapeutic target [185]. This proposition is encouraged by the observation that microenvironment deletion leads to tumor’s drug sensitivity restoration [186, 187]. However, even if physiological stem cell niches are known to play important roles in the maintenance of CSC quiescence and resistance to stress-inducing treatments [188], the mechanisms by which modulations in the local milieu regulate CSC behavior have yet to be fully clarified [189].
8.5 CSCs in Tumor Invasion and Metastasis
Metastasis, the path through which a tumor cell exits the primary tumor and colonizes in distant organs, is a complex process with many questions still unanswered [190].
This multistep process [191] can be summarized in two major phases:
(a)
First, the translocation of a cancer cell from the primary tumor to a metastatic site has to occur: cancer cells have to escape from the primary tumor mass and enter the microvasculature of the lymphatic and blood systems. Cancer cells moving through the circulation exhibit anchorage-independent survival features and are called circulating tumor cells (CTCs) [192]. CTCs usually head to organs where an environment suitable for their survival has been previously primed; they can be attracted by chemoattractive signals coming from certain tissues and also adhere to the wall of blood vessels through specific surface adhesion molecules. Sometimes they even settle in the bed of the capillaries of target organs, with a diameter too small to let them pass through [192]. Then, cancer cells have to exit the circulation and survive in the new microenvironment of the metastatic site.
Mechanisms controlling colonization still remain largely unknown; nonetheless, experimental and clinical data support the hypothesis that disseminated cancer cells adapting to the new microenvironment can be found as solitary viable cells in a dormant, nonproliferative state. These dormant cells can then become more responsive to proliferative signals arising from the primed microenvironment, thus forming micrometastases—whose size is maintained small by a balance between proliferation, apoptosis, and phagocytosis by the target tissue immune system—or even proliferating macrometastasis, after recruitment of an adequate blood supply [192].
8.5.1 CSCs, EMT, and Metastasis
It is now largely believed that only a small fraction of cancer cells have the capability to form metastases [157].
The definition of CSCs as the only self-renewing tumor cells capable of initiating a new tumor implies that CSCs likely have the major responsibility in invasion and metastasis [192]. This hypothesis is supported by the observations that CSCs are the only cells capable of giving rise to distant metastases and to the growth of new tumors following tail vein injection in mice [194, 195] and that cancer cells that had spread to the bone marrow display a CSC marker profile [196, 197]. The notion that CSCs are responsible for initiation of metastasis is then strengthened by the established association between CSCs and EMT [146, 147, 198]. It has been recently shown that EMT plays a key role in tumor progression and metastasis [199, 200] and that cancer cells have to activate the EMT pathway and acquire a migrating CSC phenotype in order to disseminate and metastasize [156, 171]. The acquirement of this mesenchymal-like phenotype requires cues from the tumor stromal components that secrete EMT-inducing factors (such as TGF-β) [171]. Indeed, EMT can be induced by autocrine or paracrine secretion of mediators such as cytokines and growth factors, due to the cross talk between tumor cells and the microenvironment [156, 185, 199, 200].
When they arrive at the metastatic site, cancer cells have then to undergo the reverse process of mesenchymal–epithelial transition allowing them to initiate the growth of a new tumor [201, 202].
In addition to transforming differentiated cancer cells in migrating cells with self-renewing features and enhanced proliferative capacity, EMT induces proliferation and spread of the existing CSC population, thus further increasing the chances for seeding at distant sites and forming metastases [146, 203, 204]. Moreover, cells generated by EMT show an enhanced resistance to apoptosis that certainly potentiates their capability to survive to the adverse conditions met during the translocation from the primary tumor to metastatic sites [205]. In line with these results, EMT has been associated with poor prognosis in several tumors [156, 206]. However, the role of EMT in facilitating the metastatic spread still remains to be fully demonstrated, especially since there are technical difficulties in detecting this transitory process in human cancer patients [192].
8.5.2 Signaling Pathways Involved in Metastasis
CSCs are believed to share physiological SC trafficking mechanisms; thus, migration of CSCs is probably regulated by several redundant and overlapping pathways, similarly to SC homing [194].
One of the critical regulators of metastatic spread is hypoxia that has been shown to increase the expression of Snail—a pivotal EMT-inducing transcription factor [155] shown to be expressed at the tumor–stroma interface in several cancers [156]—via the Wnt signaling activation. The Wnt/β-catenin pathway is thought to be a critical factor in the regulation of metastatic process [185, 198, 207]. Accordingly, colorectal cancer cells, residing at the host–tumor interface and thus suggested to be CSCs on the verge of metastasizing [158], exhibit a high nuclear β-catenin expression [208].
In addition, hypoxia is able to increase the expression of c-MYC, OCT4, and NANOG, important stem cell factors, in differentiated cancer cells [185, 199, 200].
Furthermore, expression of HIF2α facilitates the metastatic spread both by enhancing the tumorigenic potential in differentiated cells and by inducing proliferation and dissemination of the preexisting CSCs [146, 203, 204].
Interestingly, HGF, which has been recently shown to induce CSC properties and high tumorigenic potential in differentiated colon cancer cells [89], was used to induce cell scattering of MDCK cells in the initial studies on EMT [209].
In addition, HGF enhances migration and metastasizing capability of bone marrow hematopoietic cells, by activating the receptor tyrosine kinase MET [210], involved in metastases establishment. MET expression has been shown to be induced in marrow cells from highly metastatic melanomas by the tumor-derived factors exosomes that thus may stimulate the formation of metastases [210].
Other factors involved in CSC metastatic and invasive behavior include growth factors, VEGF receptor1 signaling, as well as cytokines and chemokines, such as the SDF-1/CXCR4 migration axis [194, 211].
The observation that SDF-1 is often highly expressed in typical sites of metastasis as lung, liver, bone marrow, and lymph nodes suggests that it is associated with a metastatic process [212]. CSCs, that express the SDF-1-specific receptor CXCR4, can migrate along a gradient of SDF-1, thus facilitating metastasis [145]. The involvement of SDF-1/CXCR4 axis in metastasis has been, indeed, described in various tumor models, such as lung, breast, colorectal, and pancreatic cancers [12, 213–217]. Several studies reported that SDF-1/CXCR4 signaling increases cancer invasion and metastasis also by enhancing the expression of metalloproteinases (i.e., MMP-2 and MMP-9) and integrins (i.e., α5-, β1-, β3-integrins), enzymes known to promote tumor dissemination by extracellular matrix degradation [218]. More recently, an alternative receptor for SDF-1 has been identified and named CXCR7, that shows a high affinity for SDF-1 and for another chemokine, I-TAC [219]. The correlation between CXCR7 expression and tumor aggressiveness, adhesion, invasion, and survival increase was observed in both in vivo and in vitro studies [220].
Another cytokine appearing to be connected to metastasis is IL-8, which, together with its receptor CXCR1, has been demonstrated to be related to ALDH+ CSCs invasion in breast cancer cell lines [221]. However, the involvement of this chemokine in the metastatic process has not yet been completely clarified. Furthermore, a recent work [222] suggests that the capability of CSCs to express the matrix protein tenascin C (TNC) demonstrates a direct relationship between CSCs and the metastatic process, since TNC expression by CSCs seems to be required to form metastases in the lungs of an animal model. TNC is described to regulate two key pathways for metastatic spread, Notch and Wnt [83, 195, 223–225], through the increase of the expression of musashi homolog 1 (MSI1) and leucine-rich repeat-containing G protein-coupled receptor 5 (LGR5), respectively [222]. The matrix protein osteopontin (Arg-Gly-Asp (RGD)-containing sialoprotein) was also reported to be involved in metastasis [226], since it interacts with CD44, α4-integrins, and α5β1-integrins, markers of cell adhesion typically expressed by stem cells [227–229].
CD44 is considered to support the recruitment of cancer cells into secondary tumor sites in lymph nodes, lungs, and bone marrow [230], and CD44+ breast cancer cells (displaying a breast CSC phenotype) appeared to have enhanced metastatic properties in xenograft models [10, 231]. However, data on CD44 are contradictory: in colorectal cancer, tumor progression has shown to be associated to a loss rather than a gain of membranous CD44 [91].
8.5.3 Premetastatic Niche
In addition to its role in controlling CSC stemness and proliferation and saving CSCs from depletion [232–234], microenvironment is suggested to be of crucial importance in metastatic spread as well. Growing evidences suggest that disseminated cancer cells are able to initiate a secondary tumor only if they migrate in a favorable microenvironment (“seed and soil” hypothesis). Thus, metastasis is likely not a random process, but it selectively takes place in specific organs, such as lungs, liver, brain, and bones, whose microenvironment appears to be more responsive to migrating cancer cells, as compared to other organs [156]. Accordingly, the engraftment of cancer cells in different organs seems to be enhanced by the establishment of a so-called pre-metastatic niche that allows secondary tumors’ initiation and growth. The cells of this pre-metastatic niche have shown to release factors (i.e., SDF-1, S1000A8, S100A9) that can attract disseminating cancer cells, thus enabling their successful homing to distant organs and metastasis development [156, 192]. It has been hypothesized that these pre-metastatic niche cells can be educated within primary tumor and then migrate to form distant metastases or activated locally or in the circulation by tumor-secreted factors [192]. The capability of the primary tumor to prime pre-metastatic niches, in preparation for and before the disseminating tumor cell arrival, by secreting systemic factors (i.e., cytokines, VEGF-A, PlGF, PSAP), has been observed in several experimental models [235–238]. The presence of bone marrow-derived cell clusters in pre-metastatic sites before the arrival of green fluorescent protein (GFP)-labeled cancer cells has been proved by flow cytometry and immunofluorescence studies [239]. These bone marrow-derived hematopoietic progenitor cells (HPC) have been demonstrated to express VEGFR1 and other hematopoietic markers, such as CD34, CD11b, c-kit, and Sca-1 [236, 240–242], thus making pre-metastatic microenvironment more hospitable for metastases. Moreover, the VEGFR1 agonist, placental growth factor (PlGF), secreted by the primary tumor, is reported to enhance the production of fibronectin by tumor stroma in pre-metastatic niches [156]; since fibronectin appears to bind to VLA-4 (α4β1), a fibronectin receptor expressed on HPC, its increased expression likely primes HPC to these sites, to establish the clusters in preparation for metastasis [210, 236]. Furthermore, an experimental model of melanoma showed that tumor fibroblasts participated in pre-metastatic niche formation by inducing the stroma remodeling that was necessary for the establishment of liver metastases [243].
Despite the increasing studies on CSC metastatic process, it is still debated whether the pre-metastatic niche only keeps metastasized CSC properties or it is also able to induce a CSC identity in differentiated cells [156]. As already mentioned, the central role of the CSC microenvironment in tumor growth and progression and metastasis formation, combined with its CSC protection against genotoxic damages, strongly highlighted CSC niche and mediators of the cross talk between CSCs and CSC niche as important therapeutic targets [156]. Thus, a better knowledge of CSCs is necessary, in order to develop improved therapies without the risk of tumor recurrence.
8.6 Tracking CSCs
Advances in imaging technology could allow to track the subpopulation of CSCs in a noninvasive manner in order to monitor migration, engraftment, and morphological differentiation and to assess their response to treatment.
Cell tracking can be performed with two molecular imaging approaches:
1.
Direct stem cell labeling by tracers, such as magnetic particles, luminescent nanoparticles, or radionuclides to directly mark cells [244].
2.
Indirect labeling by reporter-gene imaging: cells are transfected with a reporter gene that encodes for molecules that can be detected by imaging after administration of a reporter probe [245].
Both techniques can be applied to integrate existing radiodiagnostics, in order to implement their ability to provide functional information about biodistribution and activity of CSCs. Initially intended for cancer research, these tools have been most extensively tested in baseline research on human physiological stem cells and immune cells, and their use in CSCs tracking is still under investigation at an initial stage.
Bioluminescence imaging (BLI) requires incorporation of a reporter gene, such as firefly luciferase (Fluc), in the stem cells: light emission is triggered by interaction with an intravenously administered optical probe, d-luciferin. In a recent experience, breast CSCs were labeled through the expression of optical bifusion reporter genes, to facilitate their visualization in a human xenograft model on NOD/SCID mice [231]. Ethical issues concerning genetic manipulation in vivo represent a severe limitation to this technique.
Fluorescence imaging employs administration of organic fluorophores (such as fluorescein or rhodamine) that emit specific wavelengths following exposition to visible light; another valuable approach that resulted in improved stability of the compound is indirect labeling with genetically encoded fluorescent proteins. Such color-coding of cancer cells growing in vivo could allow the monitoring of cell–stroma interactions, subcellular processes, and distinction of different cell types with single-cell resolution [246]. Whole-body imaging with fluorescent proteins could represent a powerful technology to follow the dynamics of cancer development and metastasis [247], but low resolution and technical limitations linked to light penetration in depth call for further advancement in technology, like fluorescence-mediated molecular tomography [248].
Quantum dots (QDs) are inorganic fluorescent semiconductor nanoparticles with superior optical properties as compared with organic dyes: QDs have been used to study extravasation of intravenously injected, QD-labeled tumor cells in preclinical research [249]. It is still controversial whether their use could affect CD133 expression [250].
In order to increase accuracy, superparamagnetic iron oxide (SPIO, 50–500 nm) nanoparticles, ultrasmall superparamagnetic iron oxide nanoparticles (USPIO, 5 nm) [251], and manganese oxide (MnO) nanoparticles [252] have been tested as contrast agents for MRI [253]. Gadolinium–rhodamine nanoparticles, which provide a stronger positive signal, have been also tested for labeling and tracking cancer cells in vivo in rodents [254].
However, metal nanoparticles are not able to discriminate viable cells. This limitation can be overcome by radionuclides, such as fluorine-19 [255]: it was found that CD34+CD133+CD31+ stem/progenitor cells readily internalized these agent nanoparticles, without the aid of adjunctive labeling techniques, and remained functional in vivo.
Radionuclide imaging can follow the distribution and concentration of radioactive-labeled molecular tracers introduced into a subject. There are two main modalities for radionuclide imaging: positron emission tomography (PET) and single-photon emission computed tomography (SPECT). SPECT tracers directly emit a gamma ray in one direction, while PET tracers send two gamma rays in opposite directions, providing higher spatial resolution. Longitudinal tracking is dependent on the specific half-life of decay of the chosen isotope. Disadvantages include leakage of radiotracers from labeled cells and nonspecific uptake by normal tissues. Targeting stem cell surface markers with radiolabeled antibodies could provide information; longitudinal tracking could be performed with appropriate choice of radioisotope, according to its half-life of decay. For example, 64Cu-diacetyl-bis (N4-methylthiosemicarbazone) (64Cu- ATSM), a PET imaging agent, selectively accumulated in regions of CD133+ high expression in a preclinical model [256].
MicroCT is similar to the conventional CT systems but is capable of achieving a spatial resolution about three orders of magnitude lower (0.3 μm) [257]. Radio-opaque contrast agents, like gold nanoparticles attached to specific ligands, could be useful to target cell population, in order to acquire information on cell topography and behavior [258].
The imaging modalities reviewed in this chapter are characterized by different sensitivity, tissue penetration, and spatial resolution: integration of multiple diagnostic tools in a single imaging session would allow to combine the advantages of each technique.
In summary, CSC-based clinical imaging is a promising goal in the improvement of diagnostic and prognostic tools in cancer therapy. However, current developments do not allow immediate application in the clinical setting; moreover, it is debated whether large-scale use of such techniques would raise ethical issues related to genetic manipulation in patients.
8.7 CSC Resistance: Clinical Implications
Cancer stem cells account for a minor fraction of a tumor population; nevertheless, they could play a central role in treatment failure and relapse. At present, neoadjuvant treatment targets the proliferative potential of the tumors by killing rapidly dividing cells within the bulk of the tumor. However, even in the event of a considerable shrinkage of the tumor burden following a highly effective therapy that successfully affects the vast majority of tumor cells, CSCs could be unaffected. In glioblastoma, CD133+ CSC population was found to be enriched after radiation and exhibited lower rates of apoptosis in response to chemotherapy [259] in comparison with CD133−. In breast cancer, significantly increased levels of cells expressing CSC markers have been reported in residual tumor cell populations of patients after conventional chemotherapy [260, 261]. Moreover, in pancreatic cancer, CD133+ cells showed increased resistance to chemotherapeutic agents [12]. Indeed, CSCs display enhanced resistance to conventional cytotoxic agents (i.e., chemotherapy and ionizing radiation, inducing cell death mostly by DNA damage) due to numerous strategies: quiescence propensity, enhanced DNA repair, upregulated cell cycle control mechanisms, free-radical scavenging mechanisms, and specific interaction with stromal microenvironment. Development of targeted therapies based on inhibition of these features could allow to overwhelm treatment resistance, in order to eradicate CSCs and achieve long-lasting tumor remission.
8.7.1 Enhanced DNA Repair
Cancer cells improperly activate DNA repair pathways, implied in preservation of genome integrity, in order to overcome standard anticancer treatments. DNA repair pathways include [262]:
Nucleotide excision repair (NER) that corrects massive helix-distorting lesions
Base excision repair (BER) that targets point base modifications
Mismatch repair (MMR), removing mispaired nucleotides in the event of replication errors
Monoenzymatic direct repair, involving O6-methylguanine methyltransferase (MGMT), that performs a one-step methyl transfer reaction
Double-strand break (DSB) recombinant repair, a complex and cycle-dependent mechanism that encompasses homologous recombination repair (HRR), prevalent in cycling phases, and nonhomologous end-joining (NHEJ) that is eminent in G1 phase. This pathway can consequently lead to activation of secondary effectors, such as ataxia telangiectasia mutated (ATM), ataxia telangiectasia/Rad3-related kinase (ATR), and checkpoint kinases (Chk1 and Chk2)
The connection between DNA repair signals and CSC chemoresistance has been highlighted in glioma cell lines, showing that enhanced activation of ATM and Chk1 in CD133+ resulted in increased survival after irradiation, while radiosensitivity was restored with pharmacological inhibition [263]. Enhanced DNA repair ability has been also observed in breast CSCs [10]. Early clinical trials with DNA repair inhibitors, such as the MGMT-depleting agents O6-benzylguanine, in association with chemotherapy showed disappointing results [264, 265]. Attention has been drawn on PARP inhibitors, in reason of PARP-1 and PARP-2 involvement in single-strand repair via the BER pathway: these molecules have been particularly tested in breast cancers harboring BRCA mutation that relies on BER due to impaired HRR [266, 267].
A phase II multicenter study conducted in patients with advanced, refractory, BRCA-mutation carrier breast cancer evaluated two different schedules of the oral PARP inhibitor olaparib (AZD2281) in two sequential cohorts: overall response rates were 41 % (11 patients) and 22 % (6 patients) with olaparib at 400 and 100 mg, respectively, with an acceptable toxicity [268]. Iniparib (BSI-201) has been tested in a randomized, phase II study trial in association with carboplatin/gemcitabine doublet for treating metastatic triple negative breast cancers, showing a significant clinical benefit in the experimental arm [269]. However, the subsequent phase III trial failed to confirm any efficacy [270]. Chk1 inhibitors (AZD7762, PF-477736, SCH900776, LY2606368) are undergoing early phases of clinical development.
Research on the contribution of polycomb group proteins, such as BM1, to the DNA damage response pathways is another promising area of investigation [271].
8.7.2 Free-Radical Scavenging
Hypoxia is involved in radioresistance, as cells located in areas of low-oxygen tensions are less exposed to reactive oxygen species (ROS)-mediated damage [272]. It has been reported that lower levels of oxidative radicals are detected after irradiation in CSC-enriched MCF-7 breast cancer cell mammospheres in comparison with monolayer cultures [273], which could be explained by improved free-radical scavenging pathways. For example, overexpression of glutathione-related genes (Gclm and Gss) has been observed in CSCs: selective inhibition by buthionine sulfoximine (BSO), a glutamate-cysteine ligase inhibitor, induced a decrease in the colony-forming ability and restored radiation sensitivity in CSC models [274]. As previously stated, also HIF expression, in its two isoforms HIF1α and HIF2α, is implicated in CSC promotion and maintenance [275]. In some hematological malignancies, CSCs are sustained by high levels of HIF1α (under normoxia) that promotes gene expression of the stem cell transcription factor Hes1, via the stimulation of the Notch pathway. Echinomycin, an HIF1α inhibitor, was selectively effective on these CSCs [276]. Recent experiences by Lee et al. suggest that sorafenib, in combination with radiotherapy, could enhance the efficacy of irradiation on CSCs, by inhibition of HIF-1α in an in vitro breast cancer model [277].
8.7.3 Quiescence
Experimental evidences indicate that, both in vitro and in vivo, subpopulations of slow-cycling tumor cells are mostly spared by DNA-damage-induced death as compared to the bulk of tumor cells [278]. It is known that cells change in their sensitivity to DNA-damaging agents all along the division cycle, ranging from extreme sensitivity in the mitotic phase and increased resistance in late S-phase [279]. Specific therapies might induce CSCs to differentiate into more mature tumor cells, thus limiting their tumorigenic and invasive potential. Salinomycin has been described as the first “quiescence-disrupting” compound that is able to decrease the proportion of CSC phenotypic breast cancer cells and to selectively eradicate the tumor, by inducing terminal epithelial differentiation [280]. It has been also suggested that histone deacetylase (HDAC) causes a lysin residues epigenetic modification and is responsible of chromatin condensation of CSCs [281]. A novel class of therapeutic agents, the epigenetic-acting histone deacetylase inhibitors (HDACi), is currently under investigation in this setting [282].
8.7.4 Signaling Pathways
Notch-targeting agents like gamma secretase inhibitors (GSIs), a category of compounds blocking the release of the Notch intracellular domain, showed promising activity in preclinical studies and are currently undergoing clinical evaluation [283]. RO4929097, a new Notch inhibitor [284], has been extensively studied for toxicity in a number of settings, both as a single agent [285] and in combination with standard chemotherapy [286]; phase II trials are ongoing for patients with recurrent or progressive glioblastoma (http://clinicaltrials.gov: NCT01122901); nevertheless, disappointing results were observed when used as a single agent in metastatic colorectal cancer [285]. Another approach exploiting antibodies against delta-like 4 ligand (DLL4), a component of Notch signaling pathway, achieved inhibition of the expression of Notch target genes and reduced proliferation of tumor cells in a mouse model of human colon cancer, either alone or in combination with irinotecan [287].
Hedgehog signaling could be pharmacologically inhibited by targeting Smo. Preclinical studies with pancreatic cancer models showed that pharmacological Smo inhibition was effective against CD133+ pancreatic CSCs, with enhanced apoptosis, probably associated with Fas and death receptor (DR) overexpression [288], while combined treatment with Sonic Hedgehog and mTOR inhibitors, together with standard chemotherapy, has proved to be capable of eliminating pancreatic CSCs in in vitro and in vivo models [289]. Preliminary experience with Smo antagonist GDC-0449 (vismodegib) showed significant efficacy, with mild toxicity, in patients affected by basal cell carcinoma, leading to FDA approval in this setting on January 2012 [290]; nevertheless, clinical activity in other solid tumors was controversial [291] and rapid onset of Smo acquired mutation was observed in one patient treated for medulloblastoma that progressed after initial stabilization of disease [292]. Other strategies to achieve Hedgehog inhibition encompass Gli antagonists [293] or, indirectly, modulation of other signaling pathways, like EGFR and TGFβ [294, 295].
WNT: antibodies directed toward Wnt receptor Frizzled7 (FZD7) reduce clonogenicity and tumorigenicity in preclinical models of Wilms’ tumor; a synergistic gain in efficacy could be obtained with the addition of the analogues of Dickkopf1 (Dkk1), a secretase related to the differentiation of CD44+CD24 low breast CSCs [296] that prevents the formation of the Frizzled-Wnt-LRP6 complex [297]. Another strategy could imply direct inhibition of LRP6 or FZD7, which proved effective in suppression of tumor growth in in vitro models of triple negative breast cancer [298]. Kendizorra et al. described enhanced radioresistance in rectal cancer cell lines overexpressing Wnt transcription factor T cell factor (TCF-4), while sensitivity was restored by silencing TCF-4 [299]. RO4929097, an investigational Wnt pathway inhibitor, is currently being evaluated in a phase I trial recruiting breast cancer patients (http://clinicaltrials.gov: NCT01351103). Further advances in the knowledge of the Hippo pathway that intersects both the Wnt and Notch pathways could allow the development of new generation targeted therapies [300].
mTOR/AKT: inhibition of the PI3K/AKT/mTOR signaling pathway could be effective in restoring sensitivity to chemotherapy and radiation in CSCs that aberrantly activate this pathway [301]. Strategies to achieve this goal encompass pharmacological abrogation of AKT, mTOR inhibitors, and PI3K antagonists. Novel AKT inhibitors have shown to be promising in in vitro/in vivo antitumor activity, in combination with chemotherapeutic agents [302], and are currently undergoing phase I/II trials. mTOR inhibitors have been extensively studied in association with standard chemotherapy, but their activity on CSCs has not been specifically investigated yet. On stem cells from HER2-overexpressing primary breast cancer cells and on BT474 breast cancer cell line, it has been recently highlighted that everolimus, in combination with trastuzumab, provides a rationale for strategies that overcome resistance to HER2-directed agents [303].
8.8 Perspectives in Radiation Oncology
Advances in CSC biology would not only prove useful in the development of targeted drugs but represent a major challenge in the creation of new paradigms of treatment in radiotherapy.
Definition of total tumor volume and prognostic stratifications could be implemented with integration of data upon total number of CSCs, spatial distribution of CSCs, and detection of CSC niches: this information could be integrated in the treatment planning and dose prescription process. Mapping distribution of neural stem cells in human brain led to the observation—in a retrospective cohort of 55 patients affected by glioblastoma—that patients receiving higher doses to the subventricular zone (SVZ) and the subgranular layer (that could act as a reservoir for brain CSCs) experienced a benefit in progression-free survival [304].
Histopathologic reports, or imaging with radiolabeled antibodies directed to specific CSC markers, could be useful to forecast the likeliness of metastatic spread and, according the ratio of CSCs on total tumor volume, to predict radioresistance: these information could be subsequently applied to determine the correct pattern of care, for example, schedule and timing of associated chemotherapy. Biomolecular profiling on CSCs from pre-therapeutic biopsy or postoperative specimens could further drive the choice for altered fractionation schedules or appropriate target therapy, to restore radiosensitivity by pharmacological abrogation of aberrant signaling pathways, concomitant with or prior to radiotherapy. Real-time CSC-specific imaging [305] could be useful to improve feasibility and effectiveness of adaptive radiotherapy.
Moreover, assessment of therapeutic response in neoadjuvant treatments adding CSC-specific imaging could significantly improve the accuracy of restaging [306]. Ion therapy could yield a major benefit in this setting: preclinical data suggest that, in in vitro NSCLC models, protons may be more effective than photons, at the same biologically effective dose, to eradicate CSCs, despite producing equivalent effects in normal bronchial epithelial cells [307]. In a recent publication, carbon ions showed superior biological efficacy, in terms of lower CSC fraction, as compared to classical photon beam therapy [308].
8.9 Concluding Remarks
According to the CSCs theory, cancer is sustained by a small subgroup of self-renewing cells that exhibit stem cell-like properties such as asymmetrical division and ability to shuttle to a quiescent state. Despite their elusive nature, there is a growing body of data that accounts for their role in cancer initiation, progression, and resistance to conventional cancer therapies such as chemotherapy and radiation therapy. Efforts are ongoing to deeply understand CSCs biology, to refine detection and to elucidate the complex network of interactions CSCs establish with their microenvironment.
A better knowledge of the mechanisms underlying CSCs peculiar behavior could help to target this cell population, their eradication representing a valuable strategy to overcome resistance to cancer treatments and to prevent relapse.
References
1.
Clarke MF, Eaves CJ, Jamieson CH, Jones DL. Cancer stem cells – perspectives on current status and future directions: AACR workshop on cancer stem cells. Cancer Res. 2006;66:9339–44.PubMed
2.
Kanda Y. Cancer stem cells-Fact or fiction? In: Dittmar T, Zänker KS, editors. Role of cancer stem cells in cancer biology and therapy. Boca Raton: CRC Press; 2013. p. 1–22.
3.
Collins AT, Berry PA, Hyde C, Stower MJ, Maitland NJ. Prospective identification of tumorigenic prostate cancer stem cells. Cancer Res. 2005;65:10946–51.PubMed
4.
O’Brien C, Williams B, Webb RJ, Denhardt DT, et al. A human colon cancer cell capable of initiating tumour growth in immunodeficient mice. Nature. 2007;445:106–10.PubMed
5.
Ricci-Vitiani L, Lombardi DG, Pilozzi E, Biffoni M, Todaro M, Peschle C, et al. Identification and expansion of human colon-cancer-initiating cells. Nature. 2007;445:111–5.PubMed
6.
Prince ME, Sivanandan R, Kaczorowski A, Wolf GT, Kaplan MJ, Dalerba P, et al. Identification of a subpopulation of cells with cancer stem cell properties in head and neck squamous cell carcinoma. Proc Natl Acad Sci U S A. 2007;104:973–8.PubMedCentralPubMed
7.
Schatton T, Murphy GF, Frank NY, Yamaura K, Waaga-Gasser AM, Gasser M, et al. Identification of cells initiating human melanomas. Nature. 2008;451:345–9.PubMedCentralPubMed
8.
Eramo A, Lotti F, Sette G, Pilozzi E, Biffoni M, Di Virgilio A, et al. Identification and expansion of the tumorigenic lung cancer stem cell population. Cell Death Differ. 2008;15:504–14.PubMed
9.
Yang ZF, Ho DW, Ng MN, Lau CK, Yu WC, Ngai P, et al. Significance of CD90+ cancer stem cells in human liver cancer. Cancer Cell. 2008;13:153–66.PubMed
10.
Al-Hajj M, Wicha MS, Benito-Hernandez A, Morrison SJ, Clarke MF. Prospective identification of tumorigenic breast cancer cells. Proc Natl Acad Sci U S A. 2003;100:3983–8.PubMedCentralPubMed
11.
Singh SK, Hawkins C, Clarke ID, Squire JA, Bayani J, Hide T, et al. Identification of human brain tumour initiating cells. Nature. 2004;432:396–401.PubMed
12.
Hermann PC, Huber SL, Herrler T, Aicher A, Ellwart JW, Guba M, et al. Distinct populations of cancer stem cells determine tumor growth and metastatic activity in human pancreatic cancer. Cell Stem Cell. 2007;1:313–23.PubMed
13.
Li C, Heidt DG, Dalerba P, Burant CF, Zhang L, Adsay V, et al. Identification of pancreatic cancer stem cells. Cancer Res. 2007;67:1030–7.PubMed
14.
Adikrisna R, Tanaka S, Muramatsu S, Aihara A, Ban D, Ochiai T, et al. Identification of pancreatic cancer stem cells and selective toxicity of chemotherapeutic agents. Gastroenterology. 2012;143:234–45.PubMed
15.
Curley MD, Therrien VA, Cummings CL, Sergent PA, Koulouris CR, Friel AM, et al. CD133 expression defines a tumor initiating cell population in primary human ovarian cancer. Stem Cells. 2009;27:2875–83.PubMed
16.
Wu C, Wei Q, Utomo V, Nadesan P, Whetstone H, Kandel R, et al. Side population cells isolated from mesenchymal neoplasms have tumor initiating potential. Cancer Res. 2007;67:8216–22.PubMed
17.
Bruce WR, Gaag H. A quantitative assay for the number of murine lymphoma cells capable of proliferation in vivo. Nature. 1963;199:79–80.PubMed
18.
Hamburger AW, Salmon SE. Primary bioassay of human tumor stem cells. Science. 1977;197:461–3.PubMed
20.
Lapidot T, Sirard C, Vormoor J, Murdoch B, Hoang T, Caceres-Cortes J, et al. A cell initiating human acute myeloid leukaemia after transplantation into SCID mice. Nature. 1994;367:645–8.PubMed
21.
Hill RP. Identifying cancer stem cells in solid tumors: case not proven. Cancer Res. 2006;66:1891–5.PubMed
22.
Kelly PN, Dakic A, Adams JM, Nutt SL, Strasser A. Tumor growth need not be driven by rare cancer stem cells. Science. 2007;317:337.PubMed
23.
Chen J, Li Y, Yu TS, McKay RM, Burns DK, Kernie SG, et al. A restricted cell population propagates glioblastoma growth after chemotherapy. Nature. 2012;488:522–6.PubMedCentralPubMed
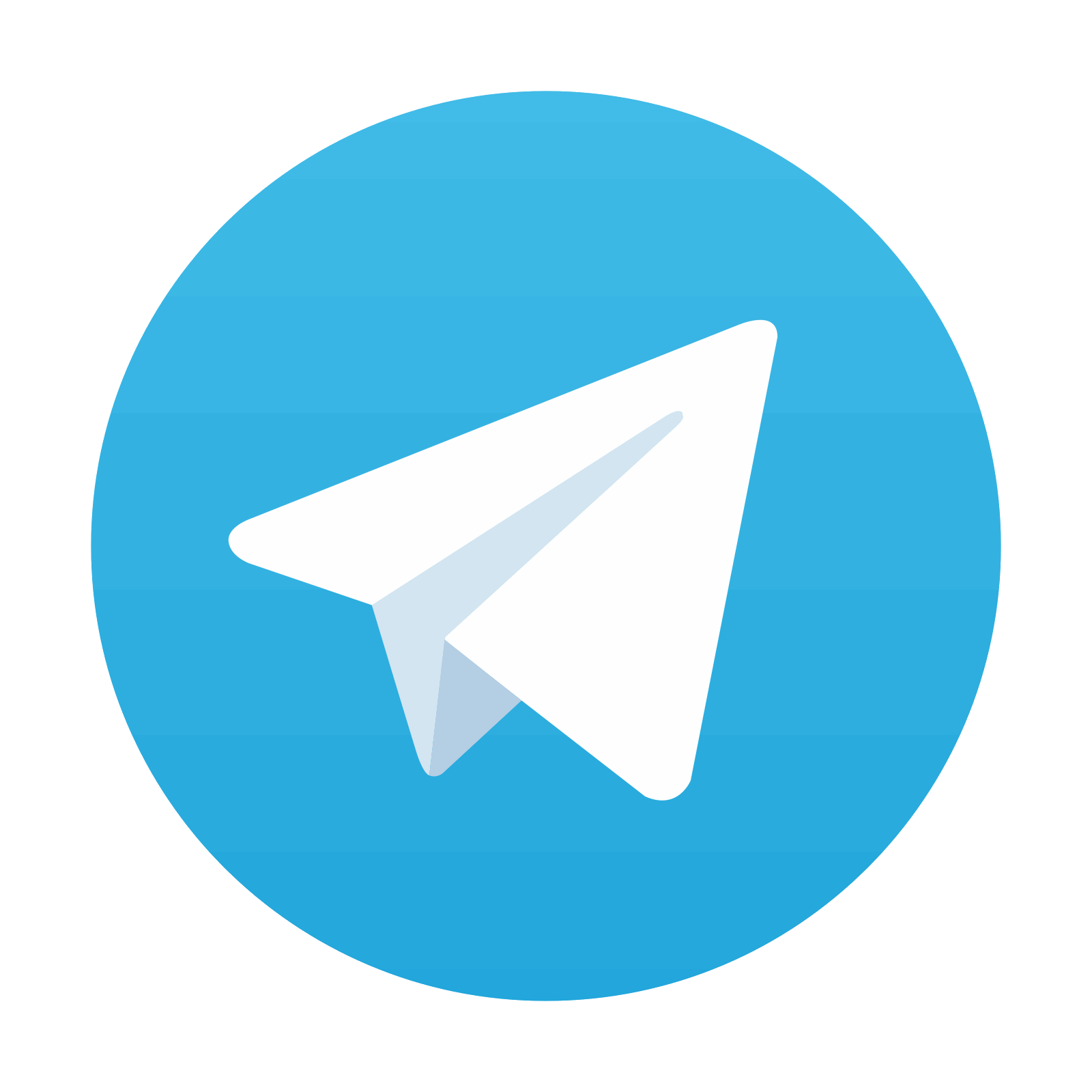
Stay updated, free articles. Join our Telegram channel

Full access? Get Clinical Tree
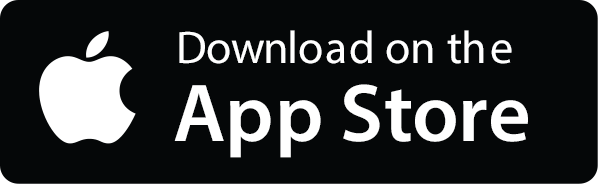
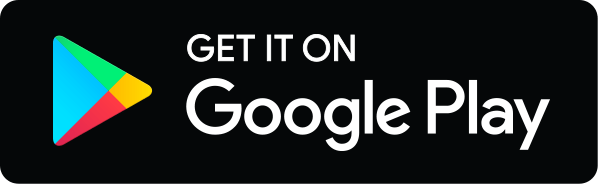