Fig. 1.1
Estimated age-standardized incidence of overall cancers per 100,000 in different countries (Extracted from)
More than the environment, host-related characteristics are also major determinants of cancer incidence. Since cancer development is the result of immune system defeat in the war against tumor cells, immune-deficient states are believed to predispose subjects to several cancers. The most common form of immune deficiency is secondary types, though; both primary and secondary forms are associated with cancer development [8–11]. Infection-related cancers, but not other cancers, have increased incidence in these subjects [9, 11]. Acquired immunodeficiency syndrome (AIDS), as one of the most important causes of secondary immunodeficiency, is associated with increased malignancies [9]. AIDS-defining cancers encompass Kaposi’s sarcoma, cervical cancer, and non-hodgkin lymphoma (NHL) with a standardized incidence ratio (SIR) of 3640.0, 5.8, and 76.7, respectively. Interestingly, Kaposi’s sarcoma risk of incidence is up to 3,640 times higher in patients with AIDS compared to the normal population [9]. More interestingly, subjects who received transplantation and are immunosuppressed with drugs have SIR of 208.0 for Kaposi’s sarcoma and are also at increased risk for other infection-related cancers [9]. Hence, immunodeficiency is an important risk factor for cancer development. All the interventions targeting immune system directly or indirectly to improve immunity will reduce infections in immune-deficient patients and thereby prevent infection-related cancers [10, 11]. In addition to immunodeficiency, chronic inflammatory states and autoimmunities predispose individuals to cancer development [12–14]. Incidence of cancers also varies in different age groups. Total cancer incidence increases by aging either in men or women (Fig. 1.2a) [15]. Overall cancer incidence per 100,000 in adults is 202.8 in men and 164.4 in women, while the corresponding figure is only 9.4 in children below 18 years. It is suggestive of up to 20 times increase in incidence of cancers by aging. Accumulating genetic alterations in a long period of time and gradual deterioration of immune system by aging, regarded as immunosenescence, are all attributed to increased incidence of cancers with increase in age [16, 17]. Interestingly, types and the course of cancers are also different between children and adults. Leukemia is the most common cancer in childhood with incidence of 2.9 per 100,000, while lung, breast, prostate, and colorectal cancers with incidence of more than 20 per 100,000 are the most common cancers in adults. With respect to rapid expansion of elder population all around the world, there is an emerging need for novel strategies to prevent and treat cancers [18].
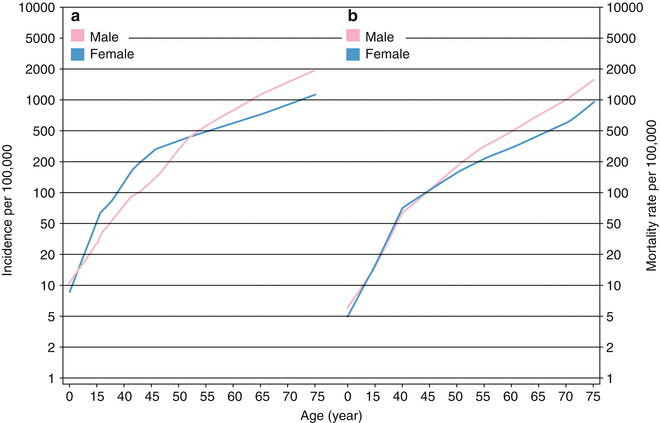
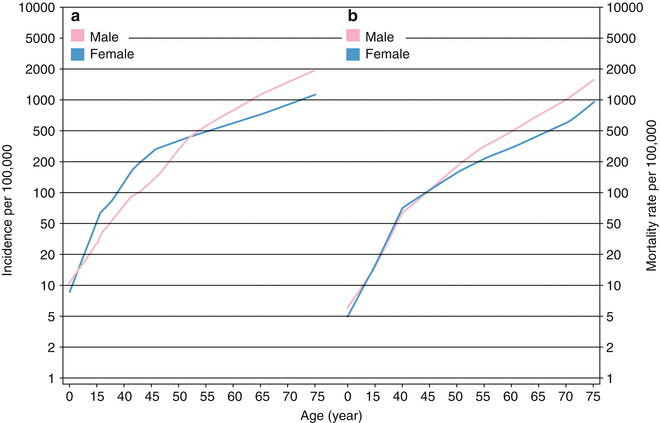
Fig. 1.2
(a) Worldwide overall cancer incidence estimation for different ages; by increasing age, incidence of cancers will increase both in men and women. (b) Worldwide overall cancer mortality rate estimation for different ages; by increasing age, mortality rate of cancers will increase either in men or women
In addition to environmental factors and host characteristics, incidence of cancers varies with respect to cancer sites. Some cancers are so common, while some are relatively uncommon (Fig. 1.3). The highest incidence of cancer is attributed to breast, prostate, colorectal, lung, and cervical cancers with incidence of 38.9, 27.9, 22.9, 17.2, and 15.2 per 100,000, respectively. Beyond the range, testis cancers, multiple myeloma, nasopharyngeal cancers, Hodgkin lymphoma, and Kaposi’s sarcoma are the least common cancers worldwide with incidence of 1.5, 1.4, 1.2, 1.0, and 0.4 per 100,000, respectively. As described in the following, fatality of cancers with high incidence is not low; notably, some like lung cancers have also the highest mortality rates among cancers. As current surgical, radiotherapeutic, and chemotherapeutic approaches failed to improve the outcome of cancers, novel approaches like immunotherapy may be the solution.
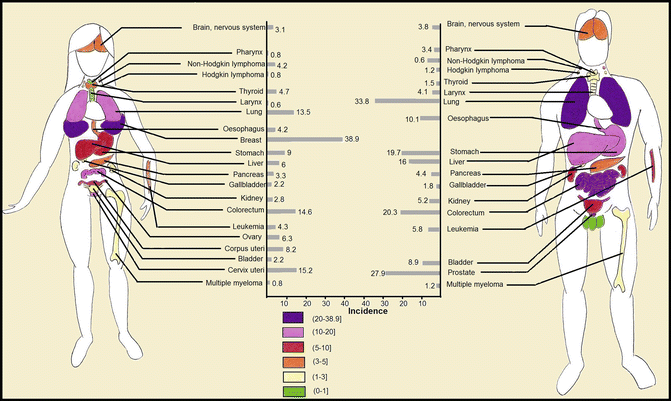
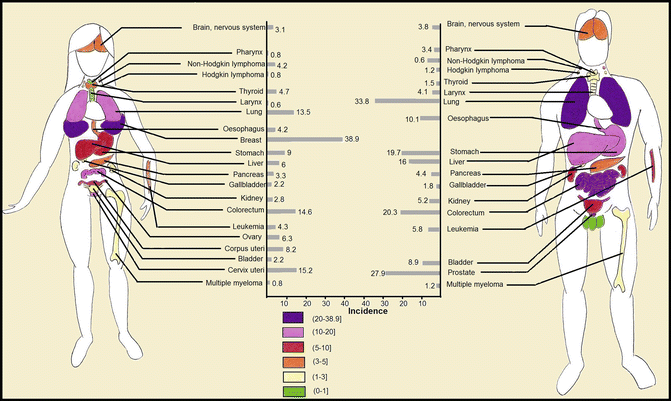
Fig. 1.3
Worldwide age-standardized estimate of incidence of each cancer type per 100,000
1.2.2 Cancer Mortality Rate
Worldwide mortality rate of overall cancers was estimated to be 105.6 per 100,000 in 2008, and it is also predicted to grow in the future if health systems do not improve worldwide. In contrast to higher incidence of cancers in developed high-resource countries, the mortality rate of cancers is higher in low-resource countries (Fig. 1.4). The highest age-standardized mortality rates of cancers per 100,000 are recorded in Mongolia with 185.2, followed by Hungary with 166.1, Armenia with 154.2, Uruguay with 150.6, and Serbia with 142.6. As evident, area of residence affects cancer status. Population composition particularly mean age of people, lifestyle, environmental factors including pollutants and status of infectious diseases in that area, and vaccination and screening programs are determinants of cancer incidence in each geographic area. However, mortality rate of cancers is affected by access to health facilities together with natural course of disease and its fatality. Proportion of cancer death to new cases is the highest in Africa; conversely the least proportional death has been recorded in North America. Disparities in receiving immunotherapy also exist in nationwide perspective in which patients with lower socioeconomic status are less likely to benefit from novel efficacious therapeutic modalities [19]. Access to current standards of care as well as novel treatments affects the outcome of cancers. It implies that our interventions are efficacious in changing the outcome of cancers but should be spread worldwide equally. To reach this goal, spread of knowledge about new therapeutic modalities as well as investment of international and national organizations on cancer are needed.
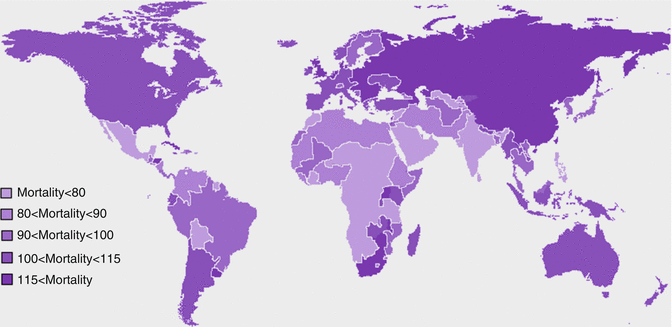
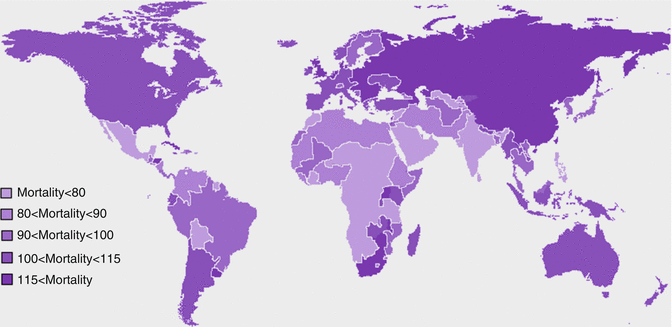
Fig. 1.4
Estimated age-standardized mortality rate of overall cancers per 100,000 in different countries
Similar to incidence, patient’s characteristics affect the outcome of cancer. Immunodeficient patients are at increased risk of cancers and also have higher risk for cancer-related mortality. However, investigations indicate that cancer-related mortality will dramatically decrease by interventions aimed to preserve immune system functions [20]. As expected, mortality rate of cancers also increases with aging (Fig. 1.2b), which may be explained by higher fatality of cancers occurring in elderly as well as deterioration of immune functions in this group of patients [21].
The greatest determinant of cancer mortality is the site of the tumor and its stage at diagnosis. A five-year survival of less than 10 % in patients with pancreatic, liver, esophageal, and lung cancers warrants the need for novel, more efficacious therapeutic modalities. In other words, more than 90 % of patients with these fatal cancers will be deceased in only 5 years. Regardless of sex, the highest mortality rates are seen in patients with lung, breast, stomach, liver, and colorectal cancers with age-standardized mortality rates of 19.3, 12.4, 10.2, 9.9, and 8.2 deaths per 100,000. Age-standardized mortality rate of different cancers in both sexes is depicted in Fig. 1.5.
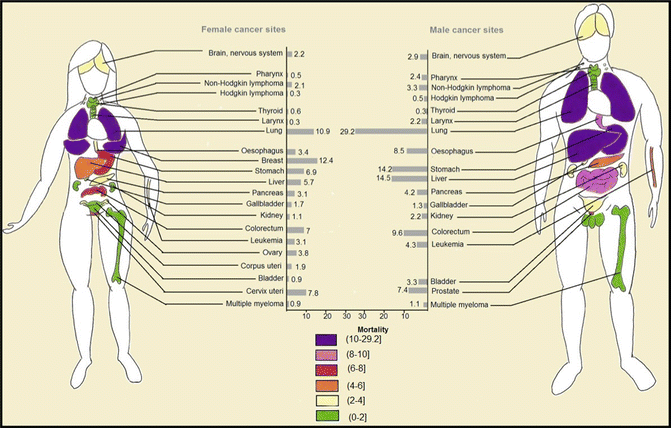
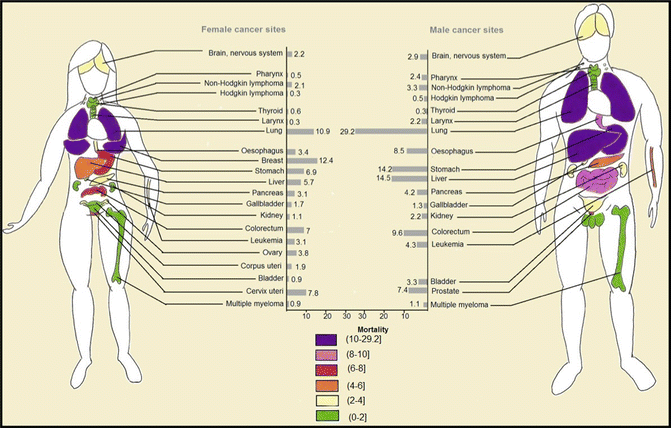
Fig. 1.5
Worldwide age-standardized estimate of age-standardized mortality rate of each cancer type per 100,000
1.2.3 Burden of Cancers
Worldwide, it was estimated that about 169.3 million healthy life years were lost due to cancer in 2008 [2]. Distribution of disability-adjusted life year (DALY) of cancers was similar to distribution of cancer death worldwide since years of life lost (YLL) accounts for higher proportion of DALY than years lived with disability (YLD) [2]. The highest YLLs are seen in Central and Eastern Europe, Southern Africa, and Eastern Asia, respectively (Fig. 1.6). Higher YLD is seen in countries with very high human development index (HDI), while YLD total is lower in countries with low HDI [2] (Fig. 1.7). As in high-resource settings, life expectancy of patients with cancers is higher; thus, patients will remain alive but with cancer-related morbidities. With respect to greater contribution of YLLs to DALYs, approximately 70 % of DALY of overall cancers is attributable to less developed countries (Fig. 1.8). The highest DALY of cancers in men is recorded in Hungary with 4,756 per 100,000, followed by Mongolia with 4,526 per 100,000, Armenia with 4,243 per 100,000, Belarus with 4,171 per 100,000, and Uruguay with 3,891 per 100,000. This figure is a bit different for DALY in women, as the highest DALY denotes to Malawi with 4,416 per 100,000, Uganda with 4,331 per 100,000, Zimbabwe with 3,594 per 100,000, Mali with 3,384 per 100,000, and Zambia with 3,357 per 100,000. Worldwide, lung cancer in men and breast cancer in women have the highest DALY; however, in Sub-Saharan Africa, infection-related cancers have the highest DALY in both sexes [2]. In Sub-Saharan Africa, infection-related cancers of Kaposi’s sarcoma, liver and cervical cancers, and NHL had the 36 % of overall DALYs, which are dramatically higher compared to other regions. Figure 1.9 shows specific standardized DALY of each cancer site in both sexes. Of note, DALYs of overall cancers are 6 % higher in men compared to women. It is in consistence with higher incidence of cancers observed in men with a rate difference of 38.4.
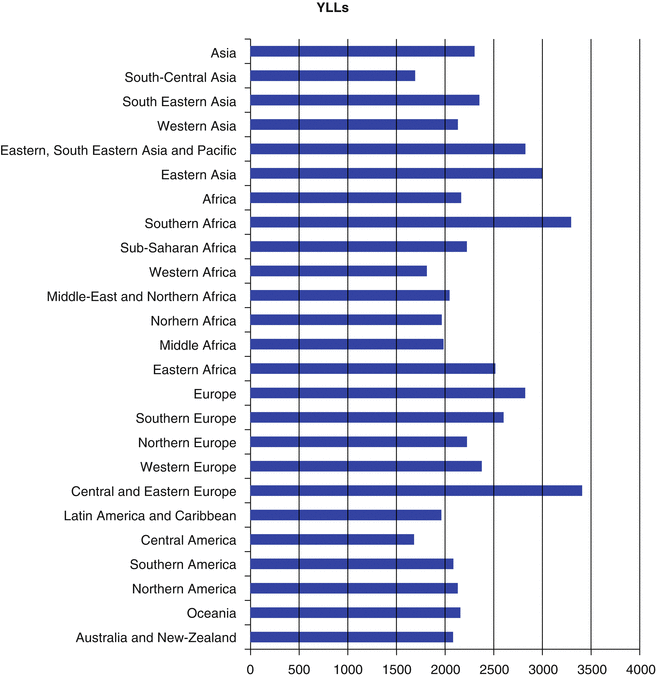
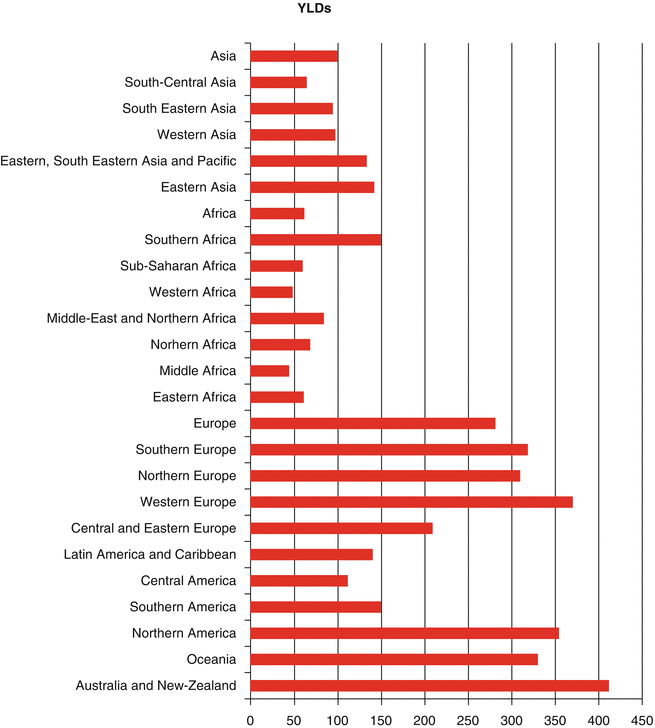
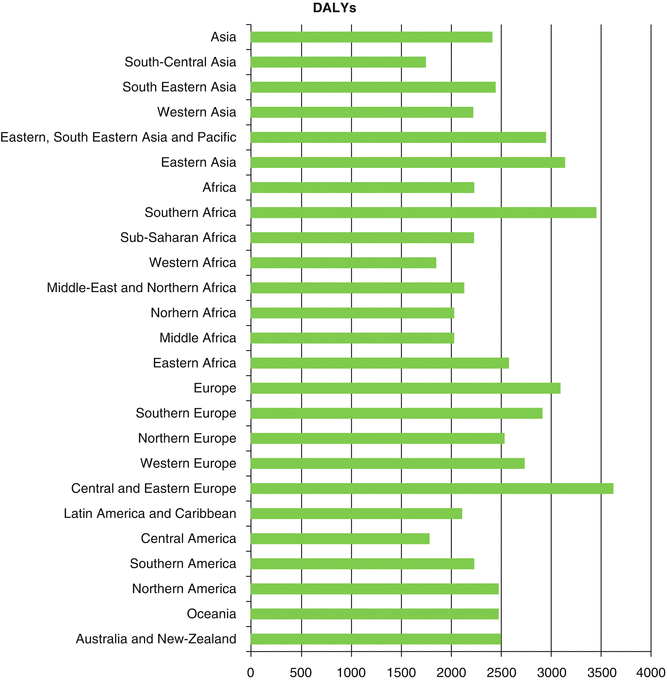
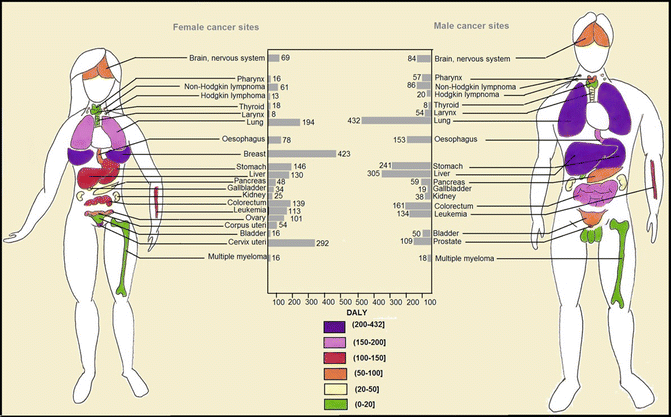
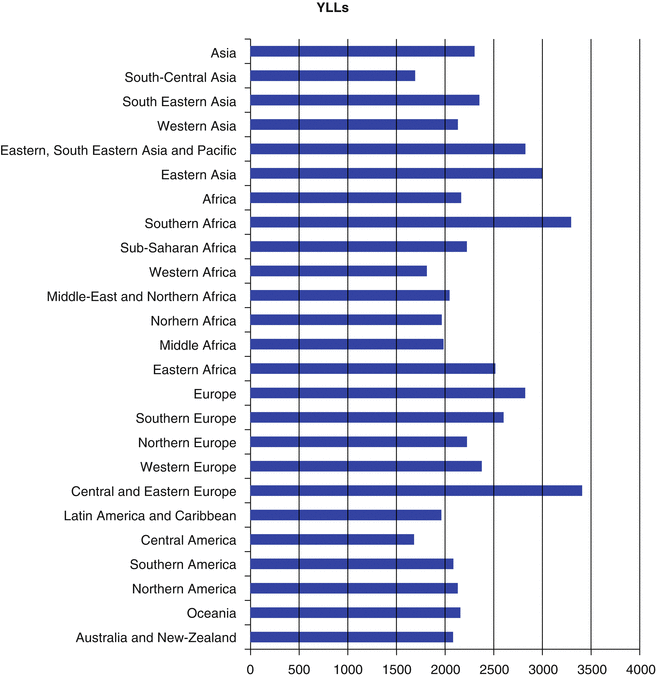
Fig. 1.6
Estimate of age-standardized rate of years of life lost (YLLs) per 100,000 for different areas
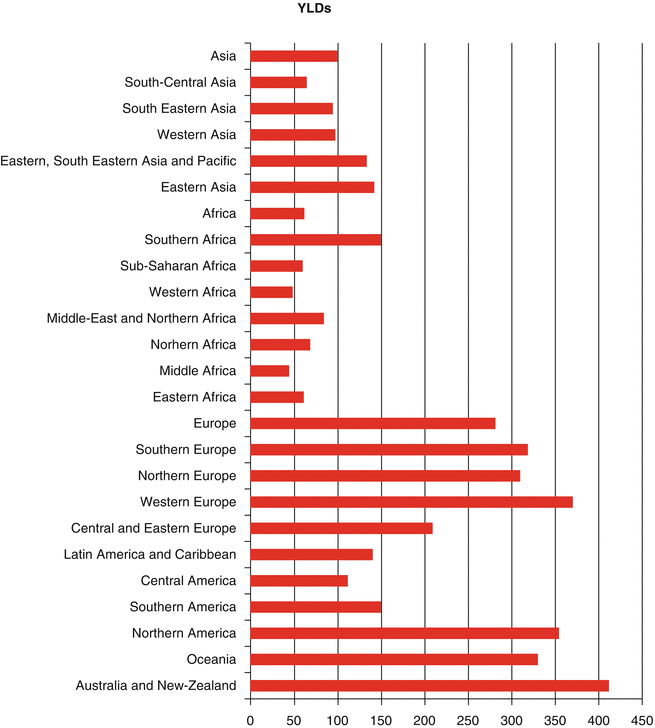
Fig. 1.7
Estimate of age-standardized rate of years lived with disability (YLDs) per 100,000 for different areas
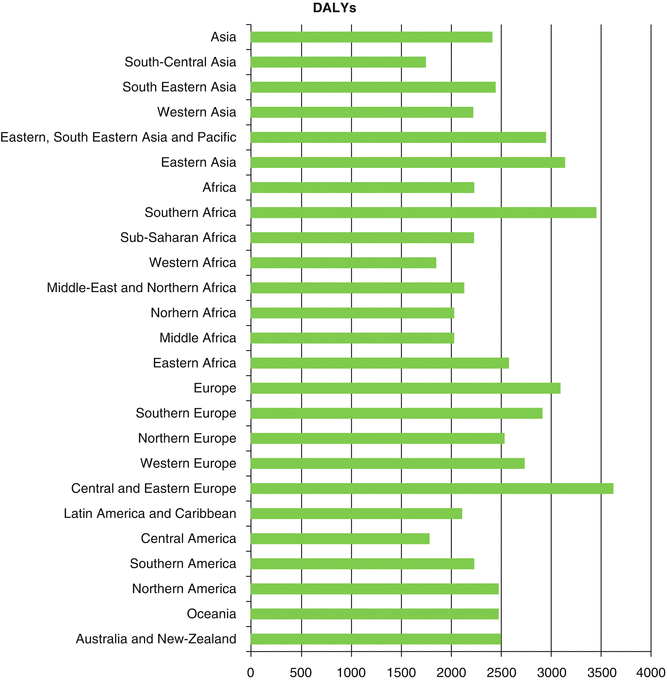
Fig. 1.8
Estimate of age-standardized rate of disability-adjusted life years (DALYs) per 100,000 for different areas
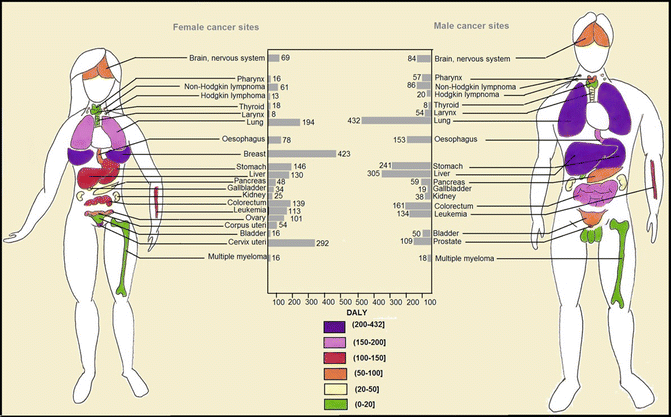
Fig. 1.9
Worldwide estimate of age-standardized rate of disability-adjusted life years (DALYs) per 100,000 of each cancer type
1.3 History of Immunotherapy of Cancers
First experience of cancer immunotherapy dates back to 1898 when William B. Coley succeeded to treat inoperable sarcomas by intratumoral injections of Streptococcus pyogenes and Serratia marcescens toxins [22]. This challenging observation of administration of bacterial products to already cancer patients with weakened immune system constructed the cornerstones of today’s cancer immunotherapy. For ensuing 50 years, the progress of cancer immunotherapy was slow with only sporadic documents of successful treatments that were mostly irreproducible. However, further studies paved the road to immunotherapy of cancers. In this way, another important step was the attempt of Maurice Hilleman to invent hepatitis B vaccine. Hepatitis B vaccine prevents the spread of hepatitis B virus (HBV) infection and its consequences such as development of hepatocellular carcinoma (HCC) by induction of active immunity against HBV. Concurrent with these investigations, in 1976, post-resection intravesical instillation of bacillus Calmette-Guérin (BCG) was shown to prolong survival of patients with bladder cancer. Indeed, cancer immunotherapy was evolving in both prophylactic and therapeutic approaches. Since the 1980s, emerging field of cancer immunotherapy was revolutionized by introduction of cytokines, monoclonal antibodies (mAbs), and adoptive cell therapy in treatment of cancers. Since then, cytokines and mAbs were tested not only as stand-alone therapeutic modalities but also in combinational schedules with chemotherapy [23–25] and radiotherapy [26]. Interferon (IFN)-α was approved for hairy cell leukemia in 1986, and it was the first immunotherapeutic drug approved for use in melanoma patients in 1995, owing to comprehensive studies by Kirkwood and his colleagues [27–29]. Rituximab is the first mAb which received Food and Drug Administration (FDA) approval for NHL in 1997 [30]. Immunotherapy also showed efficacy in postsurgical management of patients with cancers. One of the first reports dates back to 1988 when Grohn et al. employed levamisole adjuvant immunotherapy in patients with breast cancer with equivocal results [31].
Nearby these events, in 1986, recombinant HBV vaccine was developed, and efforts to constructing human papillomavirus (HPV) vaccine were initiated. The first HPV vaccine was approved by the FDA in 2007. Similar to HBV vaccine, HPV vaccine prevents viral-induced cancers, in particular cervical cancers of the genital area and anus and oropharyngeal cancers. Despite common concept about vaccines, cancer vaccination can be applied to treat neoplastic lesions as secondary line of prevention. HPV vaccination was used to treat vulvar intraepithelial neoplasia (VIN) with promising results in 2009 [32]. In addition, dendritic cell (DC) based, peptide based, and combined vaccines were introduced to treat cancer patients in the recent decade [33].
Gene therapy, by providing the opportunity to manipulate the immune system, holds a great promise for cancer immunotherapy [33]. Gene transfer with novel biologic or nonbiologic delivery vehicles enabled scientists to genetically modulate T cells to combat tumors [33]. Combination of adoptive T cell therapy with gene transfer was one the most important steps in the field of cancer immunotherapy. In this way, T cell receptor (TCR) gene transfer was one of the greatest achievements in treating cancers, reached in 2001 [34]. Interestingly, further investigations suggest hopes for combination of cancer vaccines with current mainstays of cancer treatments. One of the most interesting studies was conducted by Antonia et al. on patients with extensive-stage small cell lung cancer in 2007 [35]. The patients received dendritic cells transduced with the full-length wild-type p53 gene delivered via an adenoviral vector as cancer vaccine prior to chemotherapy. Significant clinical response was observed in more than half of the patients owing to pre-chemotherapeutic stimulation of the immune system by cancer vaccine [35]. Another progress was the combination of adoptive T cell therapy with radiotherapy made in 2005. In this experience, combined radiotherapy with intratumoral injection of the cancer vaccine was promising in patients with refractory hepatoma [36]. Vaccine-based therapeutics aim to enhance endogenous immune response against cancers, while adoptive T cell therapy is based on infusion of primed tumor-specific T cells. Finally, sipuleucel-T (Provenge), an active autologous dendritic cell-based vaccine, received FDA approval for patients with castration-resistant prostate cancer in 2010 [37]. Sipuleucel-T is the first and the sole FDA-approved therapeutic cancer vaccine. Unfortunately, no adoptive T cell therapy has yet obtained FDA approval maybe due to its obstacles in providing sufficient amounts of primed specific T cells [38]. However, cancer-testis antigens of MAGE family with restricted expression in tumor cells hold promise for the future of not only cancer vaccines but also adoptive T cell therapies [39, 40].
Almost all the progresses in immunotherapy are owned to progresses in understanding the immunopathology of cancers. This is well reflected in development of novel monoclonal antibodies and immune adjuvants through the past decade. Ipilimumab (also known as MDX-010-20 in clinical trials) received FDA approval for treatment of patients with advanced metastatic melanoma in 2011. Ipilimumab blocks the inhibitory effects of cytotoxic T lymphocyte-associated antigen 4 (CTLA-4) on presenting the tumor antigens and improves cytotoxic T lymphocyte function [41, 42]. Pegylated IFN-α has been approved for treating patients with advanced melanoma in March 2011 [33]. Another progress was the discovery of pattern recognition receptors and targeting them in cancer immunotherapy; imiquimod (Aldara), a Toll-like receptor 7 agonist, was employed in the treatment of VIN since 2008 [43]. Imiquimod has been approved by the FDA for external genital warts, papilloma, superficial basal cell carcinoma, and actinic keratosis [44]. Imiquimod administration results in endogenous induction of IFN production [44]. Figure 1.10 shows a timeline of important events related to understanding either the immunopathology of cancers or immunotherapy of cancers.
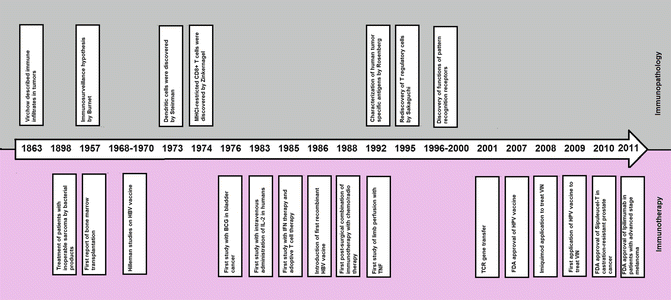
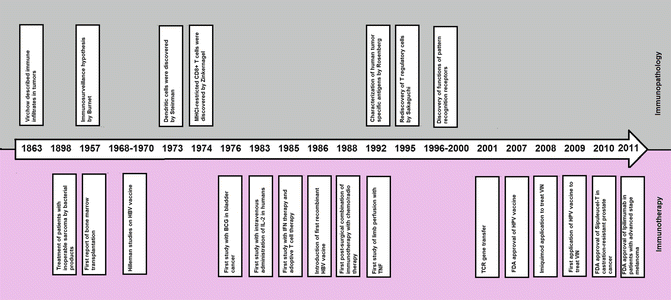
Fig. 1.10
Timeline of important events related to immunopathology and immunotherapy of cancers. Upper side of the arrow shows events related to immunopathology, whereas events related to immunotherapy are shown in the bottom of the figure. TCR T cell receptor, IL-2 interleukin-2, IFN interferon, TNF tumor necrosis factor, BCG bacillus Calmette-Guérin, HBV hepatitis B virus, HPV human papilloma virus, MHCI major histocompatibility complex-1, FDA Food and Drug Administration, VIN vulvar intraepithelial neoplasia
Through more than a century of experience with immunotherapy, scientists and health-care providers aimed to reinstate immune surveillance against tumor cells in either primary lesions or metastases by immunotherapy. Immunotherapy provides a dynamic and specific formation of adaptive immune response that fights tumor-mediated immunoediting. This effect of immunotherapy promises long-term protection against relapse of cancers, while it may not be efficacious as other drugs, radiotherapy, and surgery in immediate debulking of tumor masses. Hence, combinational therapies may be the key to improve both tumor progression and overall survival of the patients. Despite brilliant progresses in the field of cancer immunotherapy, it is still in its infancy and may provide definite treatment for all cancers in its maturity.
1.4 Immunotherapy Is Going Upstream to Combat Cancers
Immunotherapy helps health-care providers prevent not only cancer development but also prevent its further progression and cancer-related complications offering prevention from cancers at all levels. Modulation of immune responses in favor of enhancing tumor cell detection and immune clearance of these cells is what immunotherapy does. In addition, immunotherapy helps recover an injured or completely destroyed immune system after intensive cancer therapies as occurred in intensive chemotherapy schedules.
Chronic inflammation owing to infectious etiologies or continuous sterile inflammation appears to cause cancers of variable origins [45]. Targeting the immune system to control infections known as causes of variable cancer as well as conditions associated with chronic inflammation (i.e., autoimmunities) results in a dramatic decrease in incidence of cancers [46]. This application of immunotherapy prevents cancers at the primary level. Interestingly, not all subjects with predisposing chronic inflammation state develop cancers. For instance, the human herpesvirus 8 (HHV8) causes Kaposi’s sarcoma in the context of HIV caused by immunodeficiency or drug-induced immunodeficiency. Hence, immunotherapeutic approaches prevent the spread of HIV in the community and can be regarded as primordial level of prevention from cancers. A considerable numbers of vaccines and immune adjuvants as well as monoclonal antibodies are developed to combat cancers at primary and primordial stages.
On the other hand, a broad spectrum of immunotherapeutic medications have been developed to treat patients with cancers. At this stage, immunotherapy acts as the second level of prevention from cancers. Adoptive cell therapy, therapeutic cancer vaccines, immune adjuvants, cytokines, monoclonal antibodies, and gene therapies are already established to treat different cancers. Treatment of an already diagnosed patient with any type of cancer is designated as the secondary level of prevention from cancer. This is the most known kind of use of immunotherapy to combat cancers; however, it acts lately after establishing the cancer and usually can eliminate cancer in a limited number of patients. Today, immunotherapy is considered as a first line of treatment of a wide range of cancers. Immunotherapy also offers hope for patients failed with other available therapeutics and patients with end-stage cancers. In addition, combination of immunotherapy with almost all available therapeutic approaches has been tested and holds promises at least to increase progression-free survival of patients. Many new immunotherapeutic drugs are now under development, and many of them are in clinical trials.
Finally, immunotherapy can ameliorate the toxic effects of other available therapeutic modalities, known as supportive immunotherapy. At this stage, immunotherapy prevents further disabilities due to cancer progression or therapies and improves quality of life of patients. Accordingly, immunotherapy also provides a tertiary level of prevention from different cancers.
1.4.1 Prophylactic Implication of Immunotherapy
It is best to prevent cancer development than to prevent cancer progression and its related complications. Immunotherapy is defined by treatment of disease using enhancement or suppression of immune responses. Accordingly, it can be used to treat cancers as well as treat underlying diseases that cause cancers. The latter is prophylactic use of immunotherapy for cancers. Elimination of infections known to cause cancers and improving the immune system by eliminating chronic inflammation states and immunodeficiency are the bases of prophylactic cancer immunotherapy. Despite complex expensive immunotherapeutic approaches employed at other levels of prevention from cancers, immunotherapy at primary level includes simple inexpensive methods. To follow a healthy lifestyle, daily intake of anti-inflammatory drugs, vaccination against oncogenic viruses, and finally immunotherapies aimed to control spread of HIV are all acting to prevent cancers at primary or primordial level.
Increased body mass index (BMI) is believed to increase the risk of several cancers [3]. Correction of lifestyle by doing regular exercise, eating fresh foods full of antioxidants, restricting calorie intake, and eating low-fat foods leads to prevention of cancers [47–49]. The main mechanism is inhibiting tumorigenesis and providing inappropriate microenvironment for tumor growth; however, a healthy lifestyle improves immune function [47, 48, 50]. Indeed, the main antitumor mechanism of having a healthy lifestyle is to prevent tumorigenesis, but combating chronic inflammation state as well as enhancing the function of effector innate cells to eliminate tumor cells should also be acknowledged [50–54]. From this perspective, a healthy lifestyle acts as an old, inexpensive, simple immunomodulatory way of preventing cancers at primary level [47, 48]. Thus, healthy lifestyle can be regarded as the first ancient immunotherapy employed against cancers.
Infection-related cancers are caused by a variety of viral and bacterial agents. Chronic inflammation, mutagenesis by integration of pathogen genes with host genome, and induction of immunodeficiency are all believed to be mechanisms by which infectious agents can cause cancers. HBV is one of the most important agents known to cause HCC. The HBV vaccine is able to induce immunity against HBV infection in more than 95 % of vaccinated subjects. Thereby, it can prevent HBV-caused HCC [55]. In endemic areas of HBV infection like Taiwan, it has been shown that HBV vaccination has resulted in decrease of HCC incidence from 1.08 per 100,000 to 0.49 per 100,000, suggestive of a 50 % decrease in HCC incidence [56]. Interestingly, in some areas like Alaska, known as an endemic area of HBV infection in the United States, HBV vaccination has eliminated HCC in children [57]. In addition, in coinfected patients with HBV and hepatitis C virus (HCV), immunotherapy with IFN-α reduces the risk of HCC development [58]. Hence, active immunotherapy against HBV infection leads to significant reduction in HCC occurrence, and it is logical to assume HBV vaccine as a prophylactic immunotherapy against cancers.
HPV vaccine is another golden step in history of cancer immunotherapy. HPV-16 and HPV-18 are responsible for more than 70 % of cervical cancers worldwide. However, HPV-6 and HPV-11 are also targeted in newer generations of HPV vaccine. The best target group of vaccine administration is young girls who are not still sexually active. Indeed, the vaccine is beneficial for someone who is not infected with the virus. Quadrivalent vaccination against HPV (16, 18, 6, and 11) results in 98 % (95 % confidence interval of 86–100 %) protection against HPV-16 or HPV-18 cancer-related lesions. It also confers weak protection against other cervical neoplastic lesions [59]. As the cervical cancer holds the second place in incidence and DALYs of cancers of women all around the world, its prevention is of utmost importance. HPV vaccine is a cost-effective approach to reduce cervical cancer incidence worldwide [60, 61]. In addition to cervical cancer, HPV-16 and HPV-18 are implicated in the development of other perineal and perianal neoplastic lesions as well as squamous cell carcinoma of the head and neck and oropharyngeal cancers. HPV vaccination can reduce risk of these cancers but with a lesser extent [62]. Vaccination of women also offers protection against HPV-related cancers in men owing to herd immunity; however, covering boys in vaccination programs is not without clinical benefits and needs further investigations [62]. Interestingly, vaccination of women with high-grade VIN with a mix of oncoproteins E6 and E7 from HPV-16 resulted in relief of VIN-related symptoms in 60 % of patients, highlighting the important role of HPV vaccine for cervical cancers [32].
By contrast to HBV and HPV vaccine, other immunotherapeutic approaches to control HIV and Epstein-Barr virus (EBV) are on the way. In the development of anti-HIV agents, targeting the adaptive immune system failed due to progressive involvement of the adaptive system. However, recent studies herald promises in targeting the innate immunity by targeting DCs, pattern recognition receptors, and alarmins [63]. Prevention from spread of HIV and progression of HIV infection to AIDS averts AIDS-associated syndromes and AIDS-defining cancers (Kaposi’s sarcoma, cervical cancer, and NHL). Immunotherapeutic approaches combat HIV spread, and others used to prevent infections in immunodeficient patients whether primary or secondary are regarded as prophylactic implication of cancer immunotherapy [10, 63]. Autosomal recessive hyper-IgE syndrome, X-linked agammaglobulinemia, common variable immunodeficiency, X-linked lymphoproliferative disease, IL-2-inducible T cell kinase (ITK) deficiency, epidermodysplasia verruciformis, and warts, hypogammaglobulinemia, infections, myelokathexis (WHIM) syndrome are primary immunodeficiency diseases with increased risk of infection-related cancers. Granulocyte-macrophage colony-stimulating factor (GM-CSF), intravenous immunoglobulin (IVIG) administration, and allogeneic hematopoietic stem cell transplantation (HSCT) provide benefits for these patients [10]. EBV is one of the most common viral infections with more than 90 % seropositivity worldwide [64]. It is implicated in the development of several cancers including Burkitt’s lymphoma, NK or T cell lymphoma, Hodgkin lymphoma, and nasopharyngeal carcinomas particularly in subjects with incompetent immune system [64]. EBV-associated lymphoproliferative disorders are of utmost clinical importance in patients who undergone transplantation. Immunotherapy with adoptive T cell transfer specific for EBV antigens promises hope to prevent EBV-associated lymphoproliferative disorders in vulnerable subjects with EBV viremia [65–67].
Sterile chronic inflammation and immune dysregulation states like autoimmunities also predispose individuals to cancer development. Sterile chronic inflammation is strongly associated with colorectal cancer development. Patients with ulcerative colitis are at increased risk of colorectal cancers that might be prevented by anti-inflammatory drugs like 5-aminosalicylic acid (5-ASA) [68]. Another group of patients suffering from familial adenomatous polyposis (FAP) has chronic inflammation state and 100 % increased risk of colorectal cancers. Celecoxib, a cyclooxygenase-2 (COX-2) inhibitor, is approved to be used for prevention of colorectal cancer in this group of patients [68]. In subjects with no underlying inflammatory disease of the gastrointestinal tract, nonsteroidal anti-inflammatory drugs (NSAIDs) reduce the risk of colorectal cancer by 18–39 %; however, to date no NSAIDs have been approved to prevent sporadic tumor prevention [69–71]. Similarly, monoclonal antibodies used in the treatment of patients with autoimmunities that predispose patients to malignancies can be regarded as another prophylactic immunotherapeutic approach [72].
Altogether, immunotherapy can be used to prevent development of cancers. Prophylactic use of immunotherapy, also regarded as immunoprevention, offers benefits for a wide range of cancers particularly infection-related cancers. Both active immunizations with vaccines and passive immunizations with monoclonal antibodies and cytokines are employed in prophylactic immunotherapy. Many other prophylactic immunotherapeutic modalities may be introduced in the future.
1.4.2 Therapeutic Implication of Immunotherapy
Immunotherapy currently has been set as a key component of therapeutic regimens of many cancers [33]. Bone marrow transplantation (BMT) following ablative/non-myeloablative bone marrow therapies is now the standard of care of many hematological malignancies. Similarly donor lymphocyte infusion following failed BMT is an accepted immunotherapy for treatment of relapsed hematological malignancies [73–75]. Once cancer develops, immunotherapy helps the patient’s immune system fight with tumor cells to prevent cancer progression and finally elimination of cancer [76]. Benefits of immunotherapy are not only restricted to patients with advanced stages of cancers, and by contrast, patients with early stages of cancers are good candidates for immunotherapy. Bacillus Calmette-Guérin (BCG) for early-stage bladder carcinoma [77–79] and sipuleucel-T immunotherapy for castration-resistant prostate cancer are all examples of approved immunotherapies employed at different stages of urological cancers [37, 80, 81]. In addition, immunotherapy offers hope for approximately all types of cancers. FDA-approved immunotherapeutic drugs are now available for chronic lymphocytic leukemia (CLL) [82, 83], NHL [30, 84, 85], Hodgkin lymphoma (HL) [86, 87], acute leukemia [88], breast cancer [89], lung cancer [90], colorectal cancers [69, 91–93], bladder cancer [78, 79], prostate cancer [80, 81], renal cell carcinoma [29, 94], basal cell carcinoma [44, 95], melanoma [96–98], cervical cancer [5, 32, 59, 60], hepatocellular carcinoma [55, 56, 58], and soft tissue tumors [99, 100]. Promisingly, a large number of immunotherapies are also under investigation. Table 1.1 shows current FDA-approved immunotherapies to treat different cancers.
Table 1.1
FDA-approved immunotherapeutic agents to treat cancers
Category | Drug/vaccinea | Recommended schedule | Indications in cancers | Side effectsc | Mechanism of action | Year of FDA approval |
---|---|---|---|---|---|---|
Immunomodulator mAb | (3 mg/kg IV/3 weeks) × 4 | Metastatic melanoma | Autoimmune reactions, gastrointestinal manifestations, colitis, hepatotoxicity rash, pruritus | Blockade of CTLA-4 | 2011 | |
Immunomodulator mAb | (1.8 mg/kg IV/3 weeks) × 4 | Relapsed or refractory Hodgkin lymphoma and anaplastic large cell lymphoma | Cytopenia (all lineages), peripheral sensory neuropathy, nausea and vomiting, fatigue, URI, pyrexia | Targeting cells bearing CD30 to deliver toxic chemicals | 2011 | |
DC vaccine | A single course with autologous DCs activated with PA2024 and GM-CSF | Castration-resistant prostate cancer | Cardiovascular events, chills, fever, fatigue, nausea, and headache | Increase tumor antigen presentation | 2010 | |
mAb | Denosumab (Prolia, Xgeva) [271] | 120 mg SC/4 weeks | Bony solid’s tumors, bone metastases | URI, UTI, cataracts, rash, gastrointestinal manifestations | Inhibitor of osteoclast maturation by RANK ligand inhibition | 2010 |
Immunomodulator mAb | Dose 1 = 300 mg; doses 2–8 = 500 or 1,000 or 2,000 mg, IV | Refractory chronic lymphocytic lymphoma, phase I/II for follicular lymphoma | Cytopenia (all lineages) | Targeting CD20, complement activation, and ADCC | 2009 | |
mAb | 9 mg/kg IV on day 1 with combined chemotherapy or 6 mg/kg/2 weeks IV with combined chemotherapy, 6 mg/kg twice per week IV in a monotherapy schedule | Colorectal cancer, refractory esophageal cancer, refractory ovarian cancer, and biliary tract cancer | Skin toxicities most commonly dry skin, rash, acne | Targeting epidermal growth factor receptor | 2006 | |
mAb | 400 mg/mb initial dose, then 250 mg/mb per week | Colorectal cancer, head and neck cancers, phase II study for lung cancer | Skin toxicities most commonly acne | Targeting epidermal growth factor receptor | 2004 | |
mAb | 5 mg/kg every 2 week | Colorectal cancers, lung cancer, renal cell carcinoma, and glioblastoma multiformeb | Hypertension, hemorrhage, ischemic heart disease | Inhibitor of VEGF-A | 2004 | |
Immunomodulator mAb, radioimmunotherapy | Dosimetric dose of 5 mCi on day 19 and therapeutic dose of 0.75 Gy on day 12 | Non-Hodgkin lymphoma, diffuse large B cell lymphoma, follicular lymphoma | Hypersensitivity reactions, thrombocytopenia | Targeting cells bearing CD20 for delivery of radiotherapeutic agent (131 I) or complement activation and ADCC | 2003 | |
mAb, radioimmunotherapy | 0.4 mCi/kg following rituximab or chemotherapy | Non-Hodgkin lymphoma | Hypersensitivity reactions, pancytopenia | Targeting cells bearing CD20 for delivery of radiotherapeutic agents, complement activation, and ADCC | 2002 | |
mAb | Alemtuzumab (Campath) [287] | 30 mg three times a week | Chronic lymphocytic leukemia | Infections, pancytopenia | Targeting CD52 bearing cells, ADCC | 2001 |
mAb | 3–5 mg/mb at 2 doses with a 3-week interval | Acute myeloid leukemia | Pancytopenia, respiratory disorders, tumor lysis syndrome, hypersensitivity | Targeting CD33-bearing cells to deliver cytotoxic agents | 2000 | |
mAb | 4 mg/kg loading, then 2 mg/kg/week | Breast cancer | Gastrointestinal side effects, hepatotoxicity, metabolic effects | Targeting ErbB2 | 1998 | |
Cytokine | 1,000 U/ml for a course of therapy | Metastatic renal cell carcinoma and advanced-stage melanoma | Vascular leak syndrome | Booster of antitumor immune responses, T cell growth factor | 1998 | |
TLR7 agonist | Cream of Aldara three times a week | Basal cell carcinoma, actinic keratosis | Hypopigmentation, redness, scarring | Booster of antitumor immune responses | 1997 | |
mAb | 375 mg/m 2 single dose | Non-Hodgkin lymphoma | Neutropenia | Targeting cells bearing CD20 for delivery of radiotherapeutic agents, complement activation, and ADCC | 1997 | |
Cytokine | Peg-IFN-α2b 3 μg/kg/week | Hairy cell leukemia, advanced-stage melanoma | Depression, pancytopenia, bleeding, fatigue, flu-like symptoms | Booster of antitumor immune responses | 1986–1997 | |
Adjuvant | Weekly intravesical instillation of BCG × 6 | Superficial bladder cancer | BCG-osis in case of immunosuppression | Booster of antitumor immune responses | 1980s |
Immunotherapeutic weapons are of wide categories: immunomodulator monoclonal antibodies whether agonistic or blocking, cytokines [interleukin (IL)-2, IFN-α, IL-12, GM-CSF, and tumor necrosis factor-α (TNF)-α], therapeutic cancer vaccines particularly DC vaccines, adoptive T cell transfer, gene therapy, and novel immune adjuvant and delivery vehicles are all available to help cancer patients. Elimination of immunosuppression and boosting of the immune responses against tumor cells are what immunotherapy does. These effects of immunotherapy offer long-term antitumor immune response that fights with already established cancer, prevents its progression, and prevents new metastases. Accordingly, immunotherapy should not logically become restricted to patients with advanced and metastatic cancers. Despite initial experiences with immunotherapy on patients who failed with other therapeutics, today, immunotherapy is set to become the first-line treatment either in combination with other therapeutic modalities or as stand-alone therapy. In addition, to accurately measure the immunotherapy-induced tumor destruction, immune-related response criteria have been developed and should be used in clinical practice and future researches. In the following, different aspects of cancer immunotherapy as therapeutic (second level of cancer prevention) have been described.
1.5 Strategies of Cancer Immunotherapy
Two main strategies of cancer immunotherapy to treat cancer patients are (1) reduction of immunosuppressive milieu and (2) boosting the antitumor responses.
1.5.1 Immunotherapy Acts to Eliminate Immunosuppression
Immunotherapy reduces immunosuppression by blocking the negative regulatory receptors and inhibitory checkpoints [CTLA-4 and programmed death-1 (PD-1)], blocking immunosuppressive cytokines [transforming growth factor-β (TGF-β), IL-10, and TNF], blocking immunosuppressive enzymes [indoleamine 2,3-dioxygenase (IDO)], and targeting the T regulatory cells. Blockade or inhibition of negative regulators of the immune system enhances endogenous antitumor responses as well as antitumor activity of the immune system following other therapeutic approaches.
CTLA-4 (CD152) is expressed on T cells in combination with a wide variety of other immune cells [101]. It acts as a negative immune regulatory receptor that switches off T cell attacks to tumor cells. Indeed, CTLA-4 is one of the main players in establishing peripheral tolerance [101]. It competes with CD28 to bind to B7-1/B7-2 with higher affinity and avidity [101]. Two mAbs have been developed to block CTLA-4: ipilimumab (MDX-010) and tremelimumab (CP-675,206). Both of these antibodies are under investigation for a wide range of cancers. Tremelimumab has been tested in treatment regimen of patients with metastatic or refractory melanoma, colorectal cancer, and prostate cancer. Unfortunately, favorable response in terms of tumor regression and improvement of survival was only detected in less than 10 % of patients [97, 102–104]. Results of trials with ipilimumab were more positive, and ipilimumab has received FDA approval for patients with metastatic melanoma [41, 98]. In addition, ipilimumab is also underway for patients with lung cancer [105, 106] and castration-resistant prostate cancer [107]. PD-1 is an inhibitory receptor present on activated T cells and B cells and has two main ligands PD-L1 and PD-L2. PD-L1 has a broad expression on not only immune cells but also nonimmune and tumor cells, whereas PD-L2 expression is restricted to antigen-presenting cells (APCs). PD-L1 is one of the most important actors in the maintenance of immunosuppressive microenvironment around tumor cells. PD-1 blocking antibody, CT-011, is under investigation for patients with advanced hematological malignancies and multiple myeloma [108, 109].
Anti-inflammatory cytokines IL-10 and TGF-β are produced by tumor cells and suppress antitumor responses. Anti-TGF-β antibodies are now in development for cancer immunotherapy. Initial experiences on animal models of osteosarcoma reduced T regulatory cell numbers and increased number of cytotoxic T lymphocyte nearby prevention from growth of new metastases [110]. Fresolimumab, a fully human anti-TGF-β, is now produced and may be tested in the treatment of cancer patients [111]. Anti-IL-10 antibodies and anti-IL-10 receptor antibodies have potential antitumor activity, but they have not currently entered into clinical trials for cancer patients [112, 113]. In addition, infliximab, an anti-TNF-α antibody, was tested in patients with RCC and resulted in 16 % partial response and 16 % stable disease among recipients [114]. TNF-α is an inflammatory cytokine, and its increased levels has been associated with poor prognosis in cancer patients [114].
IDO catalyzes degradation of essential L-tryptophan amino acid and keeps it away from activated T cells needing it for clonal expansion. Tumor cells as well as plasmacytoid DCs present in tumor-draining lymph nodes express high amounts of IDO leading to indirect suppression of antitumor responses. 1-Methyl tryptophan (1MT) inhibits IDO and prevents tumor cell growth of variable origins, but it is still under laboratory investigations [115–117].
T regulatory cells are a strong source of inhibitory signals, preventing the effect of endogenous antitumor responses and inhibiting sufficient response to immunotherapeutic agents boosting immune system. Denileukin diftitox, a conjugate of diphtheria toxin and IL-2, has efficacy in the treatment of patients with T cell lymphoma, B cell NHL, and melanoma. Further studies demonstrated its efficacy in enhancing cancer vaccine responses by depletion of T regulatory cells. Indeed, denileukin diftitox is a targeted therapy to kill T regulatory cells [84, 118–120]. Other potential targets to weaken T regulatory responses include IL-35 and MFG-E8 that block T regulatory functions [121, 122]. Figure 1.11 summarizes the immunotherapies aimed at inhibiting immunosuppression to treat cancers.
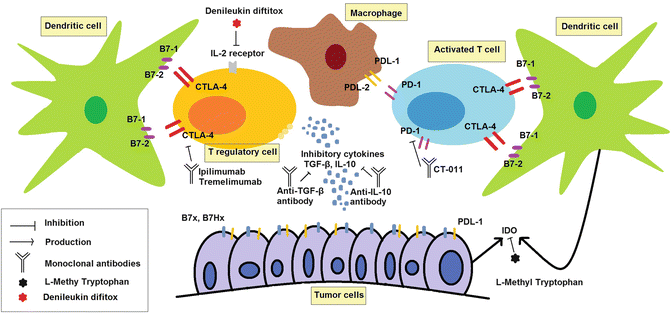
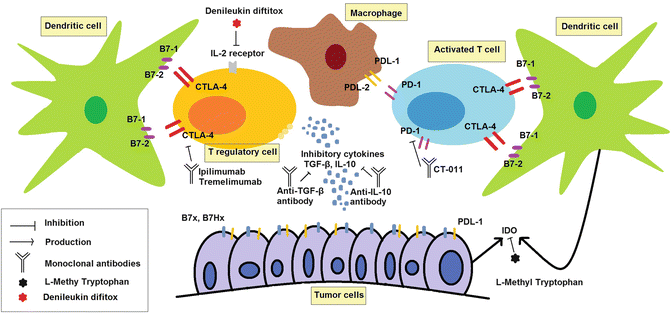
Fig. 1.11
Cancer immunotherapy eliminates immunosuppressive milieu of cancer patients. Cytotoxic T lymphocyte-associated antigen 4 (CTLA-4) and programmed death-1 (PD-1) are expressed by activated T cells and act as T cell checkpoint blockers that inhibit T cell functions. These are blocked by anti-CTLA-4 and anti-PD-1 monoclonal antibodies. Interleukin-10 (IL-10) and transforming growth factor-β (TGF-β) are inhibitory cytokines produced by T regulatory cells and tumor cells. These inhibitory cytokines are blocked by specific monoclonal antibodies that are under investigations in laboratory. Denileukin diftitox, a conjugate of IL-2 and diphtheria toxin, kills T regulatory cells and enhances endogenous or induced antitumor responses. L-Methyl tryptophan inhibits indoleamine 2,3-dioxygenase (IDO). IDO inhibits T cell expansion by degradation of essential amino acid of tryptophan
1.5.2 Immunotherapy Boosts the Antitumor Immune Responses and Enhances Killing of the Tumor Cell
1.5.2.1 Activated DCs and T Cells Are Pivotal in Cancer Immunotherapy
Immunotherapy boosts the immune responses against cancers by providing primed T cells either in vivo or ex vivo. Therapeutic cancer vaccines provide the opportunity to priming the T cells in vivo, while adoptive T cell transfer gifts ex vivo primed T cells to the immune system of cancer patients. DC vaccines constitute the most popular therapeutic cancer vaccines developed to treat a wide variety of cancers. First, DCs should be cultured from patients’ peripheral blood mononuclear cells (PBMCs). Then they should be matured (most commonly with inflammatory cytokine cocktails) and loaded with tumor antigens. Finally, they are reintroduced to the patient’s body to activate T cells and enhance antitumor responses. Some researchers prefer in vivo maturation of DCs by injection of these cells into an inflamed tissue as a simple, inexpensive, and physiologic way of maturation that enhances migration of DCs to draining lymph nodes [123]. Furthermore, antigens can also be loaded in vivo using antibodies that bind DC surface like DEC205 [124, 125]. DC vaccines are under investigation (phase I/II clinical trial) for high-grade glioma [126, 127], glioblastoma [128], hepatocellular carcinoma [129], pancreatic cancer [130], colorectal cancer [131], metastatic melanoma [132, 133], multiple myeloma [134–136], acute leukemia [137, 138], breast cancer [139], ovarian cancer [140, 141], RCC [142], and non-small cell lung cancer [143]. On the other hand, adoptive T cell transfer relies on in vitro expansion of T cells harvested from cancer patients and reintroducing these manipulated and primed T cells into the patient’s circulation. These T cells can be harvested from four major sites: (1) PBMC, (2) resections from draining lymph nodes, (3) malignant effusions, and (4) directly from tumor biopsies. However, the quantity and quality of harvested T cells from each site differ significantly; PBMCs are an easy site to obtain T cells, while biopsy-derived T cells are more reactive against tumor antigens [76, 144]. Thereafter, T cells will be engineered to express T cell receptors (TCR) necessary for tumor recognition or to express T bodies (a chimeric antigen receptor that directly binds tumor antigens) [145]. Finally, T cells can be expanded with exposure to relevant tumor antigens, activating mAbs and T cell growth factors like IL-15 [146]. CD8+ cytotoxic T lymphocyte constitute the main cells produced and transferred in adoptive T cell therapy [147]. Adoptive T cell therapy is now underway for neuroblastoma [148], hepatocellular cancers [149], gastric cancer [150], metastatic melanoma [151–153], hematological malignancies [154–156], colorectal cancer [157, 158], posttransplant lymphoproliferative diseases [159, 160], breast cancer [161, 162], ovarian cancer [163, 164], advanced lung cancer [165, 166], RCC [167], and nasopharyngeal carcinoma [168].
1.5.2.2 Materials of Activating DCs and T Cells
Tumor-specific and tumor-associated antigens as well as immunostimulatory cytokines and immune adjuvants help in activating DCs and priming the T or natural killer (NK) cells in vivo or ex vivo. Cancer-testis antigens are mainly expressed in germ cells and also appear on tumors of variable origin. However, these antigens are rarely expressed by other human cell types under physiologic conditions. Of all cancer-testis antigens, MAGE-1 family has obtained growing interest as potential target for different cancers at variable stages [169–173]. MAGE-A3 and NY-ESO-1, two cancer-testis antigens, have been used to develop cancer vaccines against melanoma [174–176], lung cancer [177], ovarian carcinoma [178], and prostate cancer [179]. In addition to tumor-specific antigens, tumor-associated antigens with narrow distribution in tumor cells are widely used in cancer immunotherapy like tumor lysate antigens in DC-loaded vaccines [37, 180, 181]. Interestingly, antigens of oncogenic viruses also exert immunostimulatory effects able to induce strong antitumor responses [182]. Tumor cells of EBV-associated nasopharyngeal carcinoma express EB nuclear antigen 1 (EBNA1) and latent membrane protein 2 (LMP2) which are EBV antigens [182]. Intradermal administration MVA-EL vaccinations, which encodes an EBNA1/LMP2 fusion protein, results in boosting T cell response against tumors [182]. Indeed, EBV-targeted immunotherapy promises hopes for patients with refractory or metastatic EBV-associated cancers [183].
Antigens can be delivered to DCs via different ways including fusion with tumor cells, loading of tumor lysates, long overlapping peptide mixtures or specific antigenic peptides, exposure with recombinant proteins, and transfection with genes encoding tumor antigens [37, 130, 139, 184, 185]. Recombinant antigens offer specific targeting of tumor cells with high safety and efficacy. Sipuleucel-T is a DC-approved vaccine for prostate cancer which contains ex vivo primed DCs with recombinant PA2024 protein fused with GM-CSF [37]. Interestingly, specific tumor antigens can be selectively delivered to patients to enhance in vivo DC uptake and presentation of tumor antigens. This direct delivery of tumor antigens is known as peptide vaccination. Subcutaneous injection of modified 9-mer WT1 peptides to patients with Wilms’ tumor results in increased frequency of CD14+, CD16+, CD33+, and CD85+ DCs [186]. However, for improving the efficacy of peptide vaccines, addition of cytokines particularly GM-CSF is of significant benefit. GM-CSF acts mainly via enhancement of antigen presentation by promotion of recruitment and maturation of DCs. Autologous or allogeneic irradiated tumor cells engineered to produce GM-CSF (GVAX) have been tested in some cancers like metastatic melanoma, colorectal cancers, non-small cell lung cancer, pancreatic cancer, and castration-resistant prostate cancer with promising results [187–194]. This approach is expensive and technically difficult; thus, it did not gain popularity among researchers and clinicians.
In addition to therapeutic cancer vaccines, cytokine monotherapy is used for the promotion of tumor death and boosting T cell responses. Isolated limb perfusion with TNF-α is approved for treatment of patients with locally advanced soft tissue tumors [99, 100]. TNF-α is a proinflammatory cytokine involved in systemic inflammation, acute phase reaction, and constitutional symptoms of cancer patients like cachexia, whereas it also inhibits tumor growth and promotes apoptotic cell death. To overcome systemic unpleasant effects of TNF-α together with selective use of its antitumor effects, it is approved for local delivery in tumor repertoire [99, 100]. Other immunostimulatory cytokines acting as T cell growth factors, increasing survival of T cells, and enhancing T cell responses to tumor antigens are used in cancer immunotherapy. IL-2 and IFN-α have received FDA approval for patients with unresectable metastatic melanoma and renal cell carcinoma (RCC) [94, 153, 195–197].
With the discovery of pattern recognition receptors, novel immune adjuvants gained considerable popularity among researchers [199]. Toll-like receptor (TLR) agonists have received FDA approval for use as an immune adjuvant for cancer immunotherapy. Monophosphoryl lipid A (MPL), a TLR4 agonist, has been used in Cervarix®. Cervarix® is a vaccine against HPV-16 and HPV-18 and prevents HPV-related cancers [95]. Imiquimod, a TLR7 agonist, received FDA approval for basal cell carcinoma, external genital warts, and actinic keratosis [95]. In addition, the development of new immunotherapeutic agents using other TLR agonists like CpG oligonucleotides continues to be an area of active research [199]. PF-3512676, a TLR9 agonist, is now in phase II clinical trial for patients with metastatic melanoma. Intravenous or intradermal administration of PF-3512676 in melanoma patients results in activation of DCs in sentinel lymph nodes and expansion of cancer-reactive cytotoxic CD8+ T cells [200, 201]. These immunological changes were in association with partial clinical response in 10 % and stable disease in 15 % of treated melanoma patients with PF-3512676 monotherapy [202]. Similarly, PF-3512676 is in phase I clinical trial for patients with basal cell carcinoma and NHL [203, 204]. PF-3512676 is also under evaluation for cutaneous T cell lymphoma, chronic lymphocytic leukemia, metastatic esophageal squamous cell carcinoma, and non-small cell lung cancer [205–210].
Finally, immunomodulator mAbs can act as agonists of stimulatory receptors on immune cells. Stimulatory mAbs have been developed for glucocorticoid-induced tumor necrosis factor receptor (GITR), OX 40 (CD 134), CD 40, and CD 137. These mAbs are now underway for a wide range of cancers from hematological malignancies to solid tumors, but these have not yet entered into clinical trials [211, 212]. Of note that, mAbs act as immunomodulators, trigger complement activation, induce Antibody-dependent cell-mediated cytotoxicity (ADCC) and also are able to provide opportunity for targeted delivery of cytotoxic materials to malignant cells (for instance 131I-tositumomab, known as radioimmunotherapy) [213]. Figure 1.12 summarizes immunotherapies aimed at stimulating antitumor immune responses.
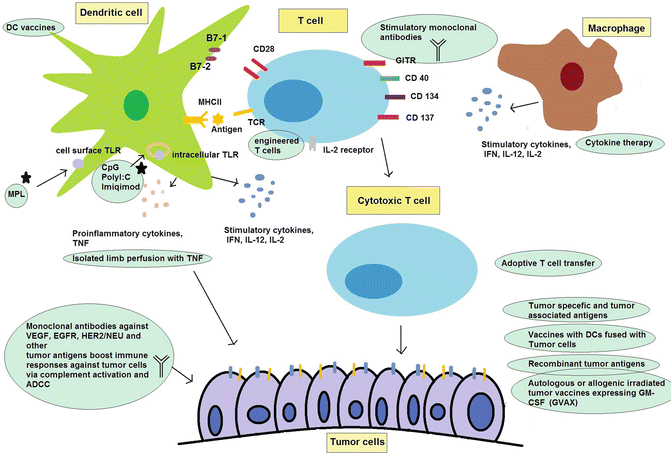
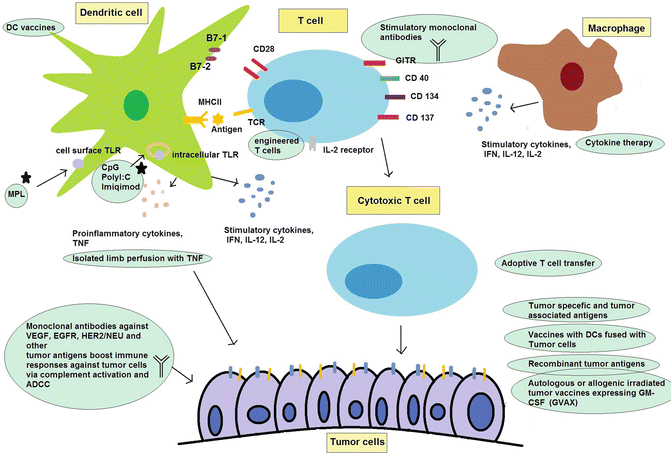
Fig. 1.12
Immunotherapy boosts antitumor immune responses and kills tumor cells. Dendritic cell (DC) vaccines and adoptive T cell transfer are two main ways of enhancing antitumor responses. Tumor antigens, immune adjuvants, and stimulatory cytokines help improve DC functions and provide cytotoxic T lymphocyte. Tumor cells themselves are the best source of antigens to produce cancer-reactive T cells. Monoclonal antibodies act as immunostimulatory agents to direct stimulation of T cells or bind tumor cells and activate complement system and Antibody-dependent cell-mediated cytotoxicity (ADCC). TLR Toll-like receptor, MPL monophosphoryl lipid A, MHC II major histocompatibility complex II, TNF tumor necrosis factor, IFN interferon, IL interleukin, GM-CSF granulocyte-macrophage colony-stimulating factor, VEGF vascular endothelial growth factor, EGFR epidermal growth factor receptor, HER2/NEU human epidermal growth factor receptor 2, GITR glucocorticoid-induced tumor necrosis factor receptor
1.6 At Which Line of Treatment?
Since the introduction of cancer immunotherapy, it has been used mainly as the last line of treatment of patients with advanced disease. It does not mean that cancer immunotherapy is restricted to patients who relapsed with other standards of care. As this emerging field is still in its infancy and its safety and efficacy is not well evaluated, patients who accept to enter into trials are usually at advanced stages, have several metastases, and are inoperable. Indeed, immunotherapy provides hope for who is disappointed from other therapeutic approaches. Nonetheless, the question why it cannot be considered as a first-line therapy of cancer patients at different stages remains to be answered.
For different cancers, it has been shown that considering immunotherapy as the first-line treatment did not compromise patients’ prognosis and their quality of life, but inversely improved progression-free survival and sometimes overall survival of patients. For metastatic colorectal cancer, addition of panitumumab to standard chemotherapy as first-line therapy resulted in significant improvement in progression-free survival of patients without deterioration of patients’ quality of life [214]. Panitumumab is approved for patients with metastatic refractory colorectal cancers, but it has several benefits (at least improvement of progression-free survival) for patients who have not received previous chemotherapy [91]. Similarly, use of ipilimumab in combination with paclitaxel and carboplatin improved progression-free survival of patients with non-small cell lung cancer who had not previously taken any medication [105]. On the other hand, immunotherapy is associated with better results in patients with early stages of cancers. BCG for superficial bladder cancer is a historical example of this claim. In addition, vaccination with HPV-16 oncoproteins in women with high-grade VIN resulted in relief of VIN-related symptoms in 60 % of patients [32]. Accordingly, immunotherapy is of benefit as the first-line treatment of patients with advanced or early stages of cancer. However, this hypothesis should be assessed in future studies.
1.7 Monotherapy or Combined Therapy?
For many years there was a dogma that chemotherapy and radiotherapy have deleterious effects on immunity and thereby effects of combined immunotherapy may be subsided. Initial experiences opposed to this dogma dating back to animal studies in the 1970s when intratumoral injection of cytotoxic drugs enhanced systemic immune response against tumors, cleared distant metastases, and promoted protective immunity with rechallenge with tumor cells [215]. In addition, systemic delivery of chemotherapy enhanced antitumor responses without induction of T regulatory cell depletion [216]. These observations suggested that cytotoxic chemotherapy may not be always immunosuppressive. Further studies unveiled that immunostimulatory or immunosuppressive effects of chemotherapeutic drugs depend on drug/dosage and schedule of treatment [38]. In addition, radiotherapy breaks immunosuppressive tumor microenvironment and enhances tumor antigen presentation. Accordingly radiotherapy with nonfatal doses for the immune system may enhance efficacy of cancer treatment to be combined with immunotherapy [217]. Under schedule that does not suppress effector cytotoxic T lymphocyte, induction of apoptotic death of tumor cells by chemotherapy/radiotherapy results in enhanced tumor antigen presentation and subsequent T cell activation. This is known as immunogenic cell death and constructs the basis of combined immunotherapy with chemotherapy/radiotherapy [218]. In addition, this combined therapy reduces the chance of tumor escape and resistance similar to multidrug therapy. Combined therapies are now on the way for wide varieties of cancers. Immunotherapy can be combined with radiotherapy, chemotherapy, targeted therapy (like tyrosine kinase inhibitors), and surgery [219–223]. Interestingly, radioimmunotherapy is an emerging field with introduction of mAbs bearing radioactive agents. Yttrium-90-ibritumomab tiuxetan is a mAb against CD20 conjugated to yttrium-90 and is used to treat relapsed B cell malignancies [224]. 131I-rituximab and 131I-tositumomab are other radiolabeled mAbs against CD20 [223, 225].
One of the most famous combined chemotherapies/immunotherapies is used in the treatment of hematological malignancies. Combined CHOP (cyclophosphamide, doxorubicin, vincristine, and prednisone) therapy with rituximab as first-line treatment in non-hodgkin lymphoma [226], CHOP plus 131I-tositumomab in non-hodgkin lymphoma [223], and CHOP plus rituximab in untreated mantle cell lymphoma [224, 227] are examples of combined chemotherapy with immunotherapy in hematological malignancies. Other promising results have been obtained in pancreatic cancer, one of the most lethal cancers worldwide. Algenpantucel-L immunotherapy combined with gemcitabine and 5-fluorouracil-based chemoradiotherapy improves progression-free survival of patients with resected pancreatic cancer [228]. Furthermore, yttrium-90-labeled humanized clivatuzumab tetraxetan with gemcitabine resulted in partial response in 16 % and stable disease in 42 % of patients with advanced pancreatic cancer [229]. However, combined immunotherapy with chemotherapy does not always improve clinical outcome. In esophageal cancer patients, intratumoral administration of 111In-labeled dendritic cells (DC) in combination with preoperative chemotherapy did not improve immune nor clinical response [230].
Targeted therapy with tyrosine kinase inhibitors particularly those inhibiting vascular endothelial growth factor receptors (VEGFR) promises hope for treatment of several cancers. Both small molecules inhibiting this receptor like axitinib [231] (received FDA approval for refractory RCC in 2012) and mAbs targeting VEGFR like bevacizumab (received FDA approval for metastatic colorectal cancer, RCC, and glioblastoma multiform in 2004) are now available. Despite axitinib which is a chemotherapeutics, bevacizumab belongs to immunotherapeutic agents due to activation of complement system and ADCC when binding VEGFR [89, 232, 233]. Interestingly, combination of targeted therapy with immunotherapy has also been evaluated in patients with RCC. Combination of SU5416, VEGFR inhibitor, and IFN-α2B (received FDA approval for hairy cell leukemia and advanced-stage melanoma in the 1990s) was tested in patients with RCC with no beneficial effects. By contrast, this combination leads to fatal events in 6.5 % of treated patients and thereby is discouraged [234].
Finally, immunotherapy confers benefits to patients who undergone surgery for complete resection or debulking of cancer. IFN-α2B after resection of melanoma in patients with high risk of relapse improves survival and decreases risk of relapse but jeopardizes quality of life of patients owing to IFN toxicities [235]. In addition to postsurgical benefits, immunotherapy can be employed prior to surgery and resection of tumor masses. Neoadjuvant chemotherapy like induction of resistance cells, difficulty in resection, and false shrinkage of tumor on imaging results in fast growth of residual tumor cells after resection; thus, neoadjuvant immunotherapy is more advantageous compared to neoadjuvant or induction chemotherapy [236]. Neoadjuvant immunotherapy has been tested for several cancers; carcinoembryogenic antigen (CEA)-derived MHCII-loaded DC vaccination prior to resection of colorectal metastases was promising [131]. In addition, adoptive T cell transfer prior or after surgery/radiotherapy of glioblastoma multiforme patients is an emerging solution for this lethal cancer [237, 238].
1.8 Monitoring the Immunological and Clinical Responses to Immunotherapy
It should be borne in mind that immunotherapy-induced tumor destruction may appear with delay after a period of tumor progression and metastasis. By contrast, response to other standards of care of cancer patients including surgery, radiotherapy, and chemotherapy appears early with obvious reduction of tumor size and metastasis [239]. It highlights the significance of the development of immune-related response criteria nearby classic World Health Organization (WHO) criteria and Response Evaluation Criteria In Solid Tumors (RECIST). WHO criteria and RECIST measure response to cancer therapy mostly with respect to tumor shrinkage and appearance of new metastases [240–243]. However, immune-related response criteria should be applied to measure response to cancer immunotherapy considering the distinct biology of tumor cell killing using each cancer therapy approach [242]. One of the evidences of this distinction is immune infiltrate of tumor mass caused by immunotherapy that is responsible for delayed but effective antitumor responses [239]. Major determinants of safety and efficacy of cancer immunotherapy encompass specificity of therapy or quality of primed T cells, quantity of primed T cells against cancer, and half-life of induced response against cancer [33]. This also underscores the significance of a reliable unique assay to measure immunological response to immunotherapy. Such universal standard assays let us compare immune responses in different trials. The minimal information about T cell assays (MIATA) project aims at establishing universal criteria to assess immunological response to cancer immunotherapy [244, 245]. Of note is that the best site of assessment for immune response is tumor microenvironment rather than peripheral blood or distant sites from tumor origin due to immunosuppressive effect of tumor microenvironment [244, 245].
1.9 Limitations of Cancer Immunotherapy
Several obstacles limit the implication of cancer immunotherapy including technical obstacles, side effects of immunotherapeutic drugs, and lack of broad availability of approved immunotherapeutic drugs. Harvesting sufficient amounts of T cells or DCs from cancer patients and activating them are not always easy and inversely are associated with technical difficulties, high costs, and different interindividual efficacies. Similarly, provision of autologous whole-tumor cell vaccines expressing GM-CSF is also difficult and expensive [76, 239]. In addition, novel adjuvants are needed to optimize therapy with cancer vaccines. Despite considerable investment on cancer vaccine development, less is paid on the development of vaccine adjuvant components [246].
On the other hand, bypass of tumor tolerance is inevitably associated with break of peripheral tolerance to self-antigens. Accordingly, autoimmune manifestations are the most common adverse events of cancer immunotherapy. In addition some drugs such as IFN induce fatal toxicities leading to drug discontinuation. As immunotherapy is a systemic treatment, adverse events may appear all over the body. Various side effects are observed with the administration of various immunotherapeutic drugs including gastrointestinal involvement with colitis, nausea, vomiting, and hepatotoxicity; skin involvement with rash and pruritus; endocrine involvement like adrenalitis and hypophysitis; hematological manifestations from pancytopenia to isolated neutropenia; and respiratory or urinary tract infections [39, 96, 247–249]. On the other hand, some drugs are associated with specific toxicities: peripheral sensory neuropathy with brentuximab vedotin [250], skin toxicities with panitumumab and cetuximab [251], and hypertension and hemorrhage with bevacizumab [252]. These side effects restrict the use of immunotherapy as FDA revoked bevacizumab approval for breast cancer due to its fatal side effects.
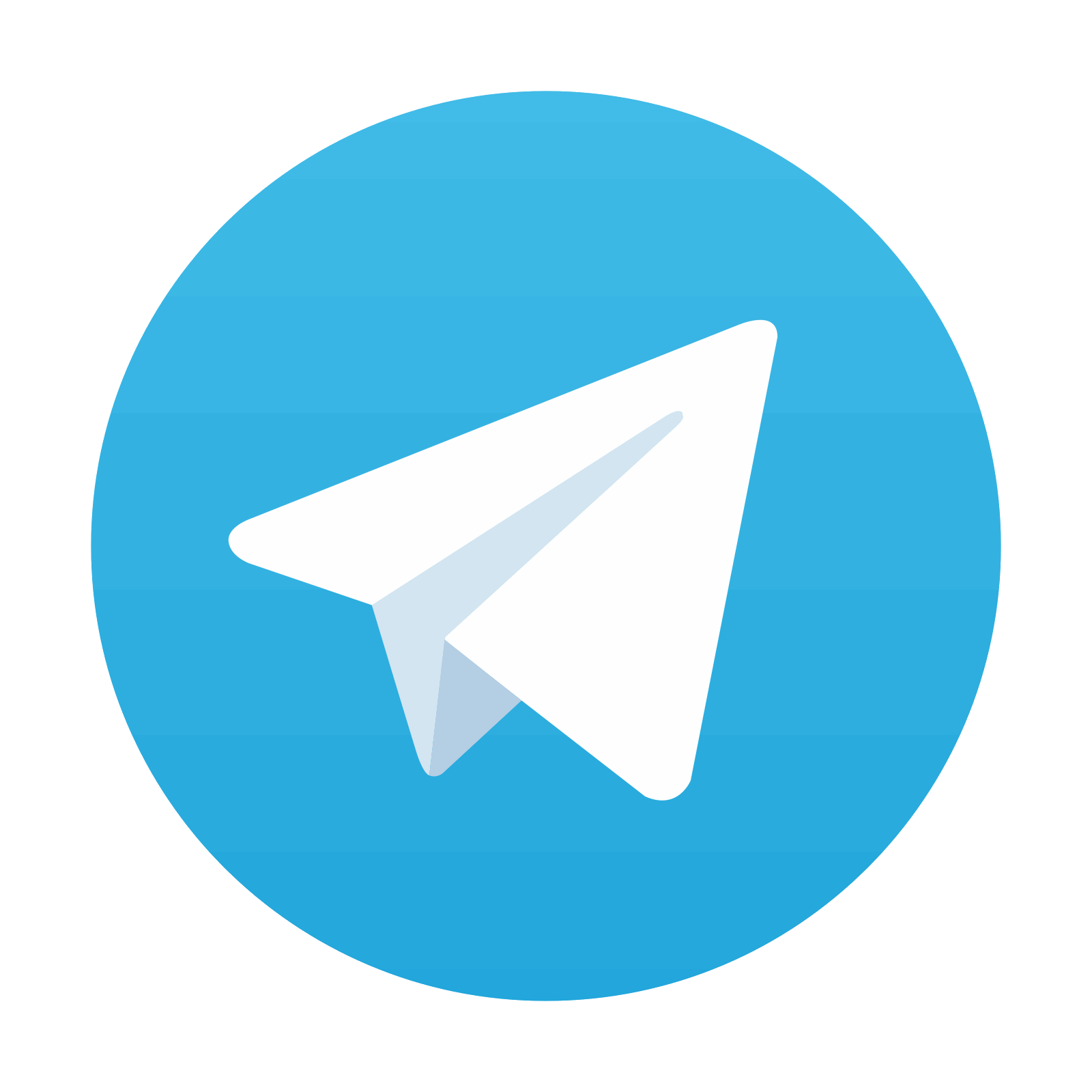
Stay updated, free articles. Join our Telegram channel

Full access? Get Clinical Tree
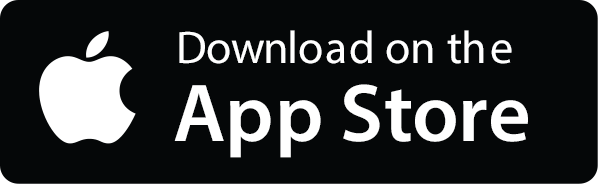
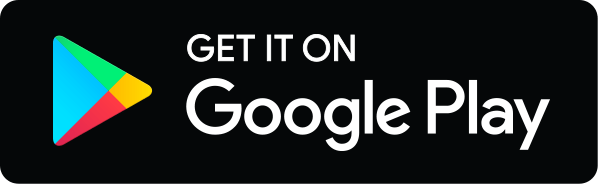