(1)
Pharmaceutical Biotechnology, Faculty of Pharmacy, Anadolu University, Tepebaşı, Eskişehir, Turkey
(2)
Yunusemre Campus, Anadolu University, Eskişehir, Turkey
(3)
Anadolu University, Tepebaşı, Eskişehir, Turkey
Abstract
Breast cancer, one of the most common female malignancy around the world, is a major public health problem. It is estimated that 1 woman in 9 will develop breast cancer during her lifetime. Conventional therapies, such as radiotherapy, chemotherapy, and hormonal therapy, have become more efficient in recent years. However, even if response is relatively good to treatments for cancers detected and treated early, the prognosis remains poor for advanced cancers due to the presence of metastases. As alternative to these conventional therapies, gene therapy is increasingly designed as a treatment solution to treat different types of cancers, such as breast cancer, ovarian cancer, lung cancer, cervix cancer, etc. Gene therapy is to repair a defective gene by introducing a healthy gene having a sequence of genetic information (DNA or RNA) into a cell to modify the expression of specific genetic program of that cell. It permits to target the causes of a disease that it is due to the mutation of a single gene or a more complex disorder. However, this repair usually requires the use of a kind of Trojan horse, which will introduce a healthy gene into the genome of the mutant cell responsible for cancer. Defined in the broad sense, gene therapy includes immunogene therapy, suicide gene therapy, correction of tumor suppressor genes, as well as oncogenes and antiangiogenic gene therapy. At this time, since gene therapy is experimental and far from clinical application running, the current data do not allow to use this approach as a alternative treatment to conventional therapy.
Keywords
Breast cancerGene therapyGenetic informationMutationOncogenesTumor suppressorsDNARNAVectorsIntroduction
Recent knowledge of the human genome and the application of molecular genetics to human biology have allowed new discoveries leading to a better understanding of how to diagnose and treat a variety of diseases. More recently, tremendous advances in molecular medicine, such as immunology, virology, genetics, and tumor biology, have generated considerable enthusiasm for gene therapy [1]. Cancers are genetic diseases that develop in a multistep process resulting from an accumulation of a series of genetic changes, such as mutations in DNA-repairing genes, in oncogenes, and tumor-suppressor genes which regulate normal cellular function in the genome of cells, causing alterations in the communication between cells [2, 3]. Breast cancer is one of the most important malignancies in women that accounts for 21 % of new cancer cases throughout the world [4, 5]. Five percent to 10 % of breast cancer has a genetic origin, i.e., hereditary, and 85–90 % of cases (known as sporadic or nonhereditary) have environmental origins that are yet to be understood [6, 7]. Conventional therapies, such as radiotherapy, chemotherapy, and hormonal therapy, have become more efficient for early detected cancers (Table 26.1). However, they are unable to cure the disease permanently and they still remain poor for advanced cancers. In parallel to these conventional therapies, gene therapy—a therapeutic strategy that embeds genes in cells or tissues of an individual to treat a disease—could be an alternative approach that can be used in the treatment of both genetic and acquired diseases [1, 9–11]. Even though the accessible and practicable vector systems for gene therapy can favorably transfer the genes into cells, the ideal delivery vehicle has not been found yet (Fig. 26.1). In this regard, many studies are ongoing to find more efficient viral and nonviral vector systems. Different therapeutic approaches can be considered when the molecular and pathophysiological basis of disease is disbanded. Pharmacological approach for which one can try to design a specific drug based on the functional properties of the deficient gene product or genetics for which manipulation based on the insertion of “transgenes” compensate the dysfunction of endogenous genes supporting the production of the deficient protein in cells where the anomaly is expressed. The prospects for this type of treatment can be applied to constitutional genetic diseases due to the alteration of a single gene or acquired diseases such as AIDS, cancer, thromboembolic disease, and cardiovascular disease or still some degenerative neurological diseases [12–14]. Insofar as the cancer is linked to the production of multiple mutations in a single cell that causes dysfunction of certain genes and the proliferation is out of control, a gene therapy permits to replace a defective gene responsible for disease by intact gene-encoding proteins. To this end, it can help prevent the action of proteins activating the uncontrolled proliferation of cells or restoring the functions of proteins that control cell division [10, 15]. Gene therapy is not a treatment, but it is one method of treatment. The principle is to “inject” a “normal” gene into a cell, in order either to replace a defective gene or to make a substance to destroy tumor cells, such as interleukin which is still experimental and cannot be prescribed outside clinical trials [16–18]. Cancer gene therapy is the transfer of nucleic acids which may be genes, portions of genes, oligonucleotides, or RNA into cancer or normal cells. Gene therapy for cancer includes different approaches, such as mutation correction, enhancement of the immune response against tumors, suicide gene, and antiangiogenic therapy to correct genetic errors or immunomodulation to reverse the malignant state [19].
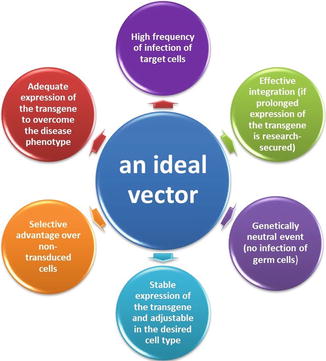
Table 26.1
Conventional therapies for breast cancer
Surgery | Breast-conserving surgery, defined as lumpectomy or partial mastectomy, combined with axillary node dissection and local radiotherapy may be used for early stage (stages I and II) breast cancers |
Radiotherapy | Postsurgical radiation therapy involves irradiation of the anterior chest wall, the ipsilateral internal mammary lymph nodes, the apex of the axilla, and the supraclavicular node |
Cyclophosphamide | An alkylating agent, cyclophosphamide cross-links DNA, which causes a misreading of the DNA template or promotion of degradation by nucleases and cell death |
Doxorubicin and related anthracyclines (daunorubicin, epirubicin, idarubicin) | These exert their cytotoxic effects by a combination of DNA intercalation, interference with topoisomerase II, formation of free radicals, chelation of metals, and damage to cell membranes |
Methotrexate | Methotrexate is an inhibitor of dihydrofolate reductase, an enzyme required to maintain the supply of reduced folates for DNA and RNA synthesis |
5-Fluorouracil (5-FU) | 5-FU exerts its cytotoxic effects through incorporation into RNA as fluorouridine triphosphate (FUTP). This incorporation interferes with RNA synthesis and function. Moreover, as fluorodeoxyuridine monophosphate (FdUMP), it interferes with thymidylate synthase, an enzyme required for DNA synthesis |
Vincristine | Vincristine exerts a cytotoxic effect by binding to tubulin and preventing its polymerization. This action interferes with the formation of the mitotic spindle and mitosis |
Mitomycin C | Mitomycin C is metabolized in vivo to an alkylating agent that binds to DNA and inhibits DNA synthesis |
Mitoxantrone | Mitoxantrone interferes with DNA synthesis by intercalation with DNA or by inhibiting topoisomerase II |
Paclitaxel | Paclitaxel causes mitotic arrest in cells by stabilizing polymerized tubulin and the mitotic spindle |
VP-16 | VP-16 causes DNA strand breaks in cells by binding to topoisomerase II and preventing rejoining of DNA strands |
LHRH analogs | Goserelin is a synthetic peptide analog of LHRH that is used as palliative treatment for premenopausal women with estrogen and/or progesterone receptor positive metastatic breast cancer |
Tamoxifen | Tamoxifen is indicated primarily for the treatment of ER-positive breast cancer. Tamoxifen may decrease the production of TGF-a by ER-positive tumor cells |
Letrozole | The aromatase inhibitors anastrozole and letrozole block estrogen synthesis by inhibiting cytochrome P450 aromatase |
Trastuzumab | Trastuzumab is a humanized, monoclonal antibody that binds specifically to the HER2/neu growth factor receptor. The antibody acts by blocking the ligand activation of the HER2/neu-mediated signal-transduction pathway or by activating antibody-dependent cellular cytotoxicity (ADCC) |
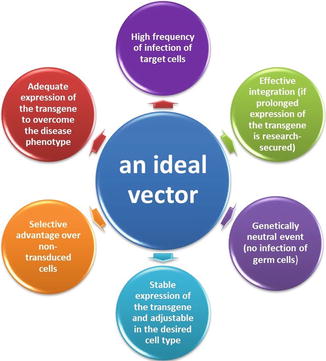
Fig. 26.1
Features required for an ideal vector
Basically two types of gene therapy have been applied to humans, germinal and somatic:
Germinal gene therapy introduces transgenic cells into the germ line as well as into the somatic cell population, not only to achieve a cure for the individual treated, but some gametes could also carry the corrected genotype.
Somatic gene therapy focuses only on the body, or soma, attempting to effect a reversal of the disease phenotype by treating some somatic tissues in the affected individual [20].
For cancer gene therapy, broadly speaking there are three strategies which are used to achieve the goals of gene therapy: (1) in vitro/ex vivo, which initially involves removal of the patient’s target cells in order to culture in vitro and are then incubated for genetic modification with the viral vector carrying the therapeutic gene. The genetically altered cells are then reintroduced into the patient’s body. This method is used particularly with blood cells that are easy to remove and reinsert; (2) in situ consists of placing directly the transfer vector into the target tissue. This technique is used particularly in cases of cystic fibrosis (transfer vectors in the trachea and bronchi), muscular dystrophy (injection into the muscle of a vector carrying the gene for dystrophin), or cancer (tumor injection of a vector carrying the gene for a toxin); (3) in vivo involves injecting the vector carrying the therapeutic gene directly into the diseased cells of the organism via vectors and the vector is then supposed to reach specific target cells. But, of course, gene therapy is more difficult to achieve in vivo [13, 21].
Successful gene therapy requires decisions concerning the choice of suitable biological, chemical, and physical methods in order to modify the numbers of target cells necessary to obtain the desired therapeutic effect (Table 26.2).
Table 26.2
Comparison among biological, chemical, and physical methods of gene delivery
Gene delivery method | Advantages | Disadvantages |
---|---|---|
Biological method | ||
Retroviral vectors | High transfection efficiency in dividing and nondividing cells | Not easy to manufacture and require cold storage |
Adenoviruses | Possible targeted delivery | Cumbersome quality control requirement |
Adeno-associated viruses | Possible systemic delivery | High cost, immunogenicity and oncogenicity risks |
Lentiviruses | Stable expression | Limited size of gene to be inserted |
Other types viral vectors | ||
Physical methods | ||
Direct intramuscular injection of plasmid DNA | Higher local tissue transfection efficiency | Need for a specific instrument |
Direct intracellular microinjection | Direct intracellular microinjection | Need of parameter optimization for different types of cells |
Electroporation | Transfection in all cell types, even in difficult to transfect cells, is achievable | Higher tissue damage observed |
Jet injection | Easy process standardization | |
Particle bombardment (biolistics) | Less limit on gene size | |
Mostly ex vivo applicable | ||
Chemical methods | ||
DEAE-dextran | High in vitro transfection achieved | Low in vivo transfection efficiency |
Calcium phosphate co-precipitation | Possible in vivo organ targeting | Low efficiency in primary and nondividing cells |
DNA-polylysine-cell receptor conjugates | Less costly | Limited clinical success |
Polybrene-DMSO | Less limit on gene size | Consistent reproducible large-scale manufacturing |
Liposome-mediated DNA transfer | Simple to manufacture in small batches and storage conditions are more flexible | |
High commercial interest |
Basis of Gene Therapy for Cancer
Progress in cancer gene therapy is largely depending on the development of novel vectors with maximum therapeutic efficacy at the target site and minimal toxicity to normal tissues [23].
Vector Characteristics for Gene Transfer
Once the gene is selected for its therapeutic potential against a disease, a crucial step in gene therapy is to enter the new genetic information into the targeted tissue or cells [21, 24]. Several hurdles can prevent the efficient uptake sequence of genetic information into cells. Such hurdles include the physical makeup of the proteins and carbohydrates matrix of extracellular milieu, which can physically hinder the movement of exogenous materials. Secondly, immunologically mediated factors, such as opsonins and phagocytes, can recognize and digest materials identified as “foreign.” In addition, both intracellular and extracellular enzymes such as DNAse and RNAse can destroy new genetic material to be transferred. In order to achieve efficient cellular uptake, these “genetic information cargoes” need to be carried within a vehicle. For this purpose, delivery of therapeutic genes involves the use of carrier vehicles, called vectors. For the optimum therapeutic effect, certain conditions must be fulfilled by the vector and the gene(s) to be transferred: (1) the vector should have high transfection efficiency; (2) the vector must particularly target disease cells, such as the tumor cells including metastatic cells; (3) for optimal gene expression, there should be controllable genes which can be combined with appropriate promoter enhancer sequences; and (4) the vector must have low toxicity and low immunogenicity (see Fig. 26.1) [25, 26]. Currently, gene therapy vehicles or vectors can be broadly divided as viral, nonviral, and physical methods. Each has own advantages and disadvantages, as shown in Table 26.3 [26–29].
Table 26.3
Main characteristics of the vectors currently authorized in clinical practice or study
Vectors | Benefits | Disadvantages |
---|---|---|
Retrovirus | Integration into the genome of the host | Only infects cells in cycle |
Prolonged expression in dividing cells | Generally low transfer efficiency | |
Nontoxic in the absence of helper virus | Limited transfer of 8–9 kb DNA | |
Variety of potential target cells | ||
Adenovirus | Transfer of DNA 15 kb | Transient expression due to the non-integration into the genome host |
Variety of potential target cells | Highly inflammatory and immunogenic, making it difficult to repeated administration | |
Ability to produce large amounts of virus | Risk of recombination in the case of exposure to wild virus with possibility of restoring infectivity of the virus used for the transfer | |
Authorizing the stability in vivo | ||
High expression of the transgene | ||
No integration into the host genome: no risk of insertional mutagenesis | ||
Adeno-associated virus (AAV) | Nontoxic (no known association with human disease) | Low efficiency of infection requiring the use of a large number of viral particles |
Specific integration into the host genome at a site known (chromosome 19) | Low frequency of integration into the genome of the host | |
Authorizing the stability in vivo | Requires using molecules produced by adenovirus to be introduced into the target cell | |
Variety of potential target cells | Being introduced into the target cell | |
Preparation is very difficult for large amounts of virus | ||
Difficulty in obtaining preparations containing no helper virus (required for adenovirus manufacture of AAV) | ||
Limited size of the transgene | ||
Lentivirus | Infection of quiescent cells | Theoretical risk of recombination with wild-type virus highly pathogenic |
Integration into the genome of the host | Specificity restricted to CD4 positive (in the absence of modification of the envelope) | |
Targeting possible by modification of the envelope proteins | Expression of the transgene unstable | |
Herpesvirus | Large payload capacity (30 kb) | Lack of integration in the genome of the extended potence |
Easy handling | Significant cytopathic effect (1st generation) | |
High viral titers | High prevalence of seropositive | |
Prolonged latency (neurons) | Possible reactivation of latent wild |
Viral Vectors
Both RNA and DNA viruses are utilized as viral vectors [30]. Having the advantage of high delivery efficiency toward a variety of cells, they were the first vectors employed in gene therapy clinical trials [31]. Viruses with a small number of genes are constructed to introduce new genetic material into the cells. They are surrounded by a protective protein coat which allows transport into the cell where the viral genetic information can be produced using the host cell’s own translational machinery. Hence they are suitable gene delivery vehicles [32]. Even though these properties make viruses extremely attractive as vectors for gene therapy, they are also responsible for their pathogenicity. The viral vectors used for gene transfer are genetically modified viruses by deleting so-called secure. The principle is to eliminate the virus sequences that encode proteins, including those associated with potential pathogenic behavior of the virus, and keep only those that are used to build the viral particle and ensure the infection cycle. The virus genome is rebuilt to carry the therapeutic gene sequences [28, 32, 33].
Retrovirus
Most retroviral vectors are derived from retroviruses and they are the basic tool used to get a good transfer and stable expression of a therapeutic transgene. Retroviruses are the first viruses tested whose genome is composed of single-stranded diploid RNA molecules (ribonucleic acid). The retroviruses genome size is about approximately 8–11 kb base pairs, and they can accept up to 7–10 kb of exogenous gene sequences [34–36]. Currently, 60 % of clinical protocols are based on the use of retroviral vectors derived from murine leukemia retrovirus (MLV, Moloney virus in particular) which is the principal virus used in vectorology [37]. When a retrovirus infects a host cell, it will introduce its RNA together with some enzymes, namely, reverse transcriptase and integrase, into the cell [28, 30, 34]. This molecule RNA retrovirus must produce a DNA copy of the RNA molecule before the integration into the genetic material of the host cell’s DNA. Retroviruses are naturally integrated into the genome of the host cell if it is in mitosis, such as tumor cells [23, 28, 34]. RNA viral vectors under development include oncoretroviruses encoding structural genes of gag, pol, and env, and lentiviruses and spumaviruses, which contain additional viral proteins [38, 39].
Lentiviruses (slowly replicating retrovirus) are another family of retroviruses that have the ability to infect cells that do not divide and have been the most studied retroviral vectors for gene delivery in recent years. This is the type of virus that causes immune suppression of HIV (human immunodeficiency virus) that causes acquired immunodeficiency syndrome (AIDS) [39, 40]. Lentiviral vectors are called “complex” because in addition to gag, pol, and env, genes, they have three to six additional viral proteins such as tat, rev, nef, vif, vpr, and vpu [38, 39]. Since these proteins facilitate an active transport of the pre-integration complex through the nucleopore, contrary to other lentiviral vectors, they do not require the breakdown of the nuclear membrane and so they are also able transduce nondividing quiescent cells [28, 41–43].
Adenovirus
Adenoviruses are double-stranded DNA viruses whose genome consists of 36–38 kb DNA in size [28]. The genetic material is contained in a protein structure called capsid. Fixation of this capsid to target cells is made possible by the presence of long protein fibers that interact with the receptor cell membrane. In order to use human adenovirus for gene transfer, E1 and E3 genes, which are essential for its replication, are deleted and replaced by the therapeutic gene sequence. This type of adenovirus is called defective [45–47]. This recombinant adenovirus is produced in vitro by infecting human cells in culture which have the E1 and E3 genes, thereby allowing the production of recombinant adenovirus. The advantage of adenovirus is its ability to infect many cell types with surface receptors [48, 49]. For this reason, adenovirus vectors are used most frequently (approximately 24 %) in gene therapy, such as for cancer. Adenoviral DNA is not integrated itself, but it is found in the nucleus of the host cell as extrachromosomal, an episome [46, 50, 51]. Although the level of expression of the therapeutic gene is generally higher in the case of adenoviral vectors, they could be associated with an immune reaction at a higher rate. This type of vector, widely used in the 1990s, is now much less considered in gene therapy [46].
Adeno-associated Virus (AAV)
AAV is a member of single-stranded nonpathogenic parvovirus. It requires the presence of helper virus, such as adenovirus or herpes simplex virus (HSV) for their propagation [52]. AAV DNA can integrate stably and efficiently into the genome of infected cells. They have the distinction of integrating specifically into the genome (19q13.3 region), which reduces the risk of activation of proto-oncogene. However, the preparation of AAV is cumbersome since it requires the presence of a helper adenovirus which must be subsequently removed by various purification steps. To this end, a very large amount of AAV is necessary to conduct in vivo tests [53, 54].
Other Types of Viral Vectors
Beyond viral vectors commonly used in clinical practice and described in the previous section, many other viruses are described as vectors, such as herpes simplex virus (HSV), pox virus, and foamy viral vectors, and identified as potential carriers for gene delivery [28].
Nonviral Vectors
Even though viral systems have a number of advantages, they are not currently safe. In recent years, different nonviral gene delivery techniques have been developed trying to combine the advantages of viral vectors while overcoming their shortcoming. Nonviral methods present some advantages over viral methods with low host immunogenicity and big DNA size. Unlike viral vectors, they are easier to produce, to handle, and to store. However, they have an associated lower transfection efficiency than that of viruses to transfer genetic information into a large population of cells, making them difficult to use in some cases such as in the case of modification of a majority of tumor cells [23, 55, 56].
Transfer of Naked DNA
This is the easiest method of nonviral transfection. In this system, the DNA is injected directly into the tissue to form plasmid. The injection is done using a gene gun, to bombard the epidermis with microparticles coated with DNA [57]. DNA reaches the nucleus, where it remains in episomal form, thus allowing a transient expression of the protein of interest. This technique does not seem sufficient to correct genetic abnormalities but may be effective as a vaccination procedure, since low expression transgene is sufficient to trigger an immune response [58]. Other physical approaches such as electroporation, ultrasound, and hydrodynamic delivery are used to transfer naked DNA [59].
Liposome-Based Nonviral Vectors
Since there are many existing barriers to administer DNA alone, in order to improve the delivery of new DNA into a cell, the DNA must be protected against damage and its entry into the cell must be facilitated. To this end, new molecules, lipoplexes and polyplexes, have been created to protect DNA from undesirable degradation during the transfection process. Liposomes were first described in 1965 as a model of cellular membrane [60] and quickly earned their candidacy for gene therapy [28, 56].
Liposome-based gene transfer is based on the encapsulation of the DNA molecule within a vesicle composed of one or more phospholipid bilayers enclosing an aqueous compartment containing different molecules such as pharmacological agents, proteins, and nucleic acids [61, 62]. There are three types of lipids used to prepare liposomes: anionic (negatively charged), neutral, or cationic (positively charged). In gene delivery, liposomes composed of cationic lipids are used most actively [63, 64]. Cationic complexes DNA-liposomes fuse with the cell membrane lipid and follow an endocytosis path. DNA released by endosomes may, in some cases, cross the nuclear membrane and be transcribed in the nucleus of the host cell [65]. Theoretically they have no limit as to the size of plasmid as vector, and since they are poorly immunogenic, they could be administered repeatedly [66].
Polymer-Based Nonviral Vectors
Biodegradable cationic polymers have also been studied for nucleic acid delivery in cancer gene therapy. These positively charged polymers, such as cationic polymers, dendrimers, and chitosan, are able to combine with anionic nucleic acids which are called polyplex. Gene transfer mediated by cationic polymer/DNA complexes (polyplexes) has been accomplished efficiently both in vitro and in vivo. Usually, in vivo gene transfer is less efficient than in vitro, and it is not well predicted by in vitro results [67, 68].
Gene Therapy for Breast Cancer
Today we know that many genes are involved in the transformation of a normal cell into a cancer cell by controlling cell division. They are divided into two major families of opposing action: proto-oncogenes and tumor suppressors (antioncogenes).
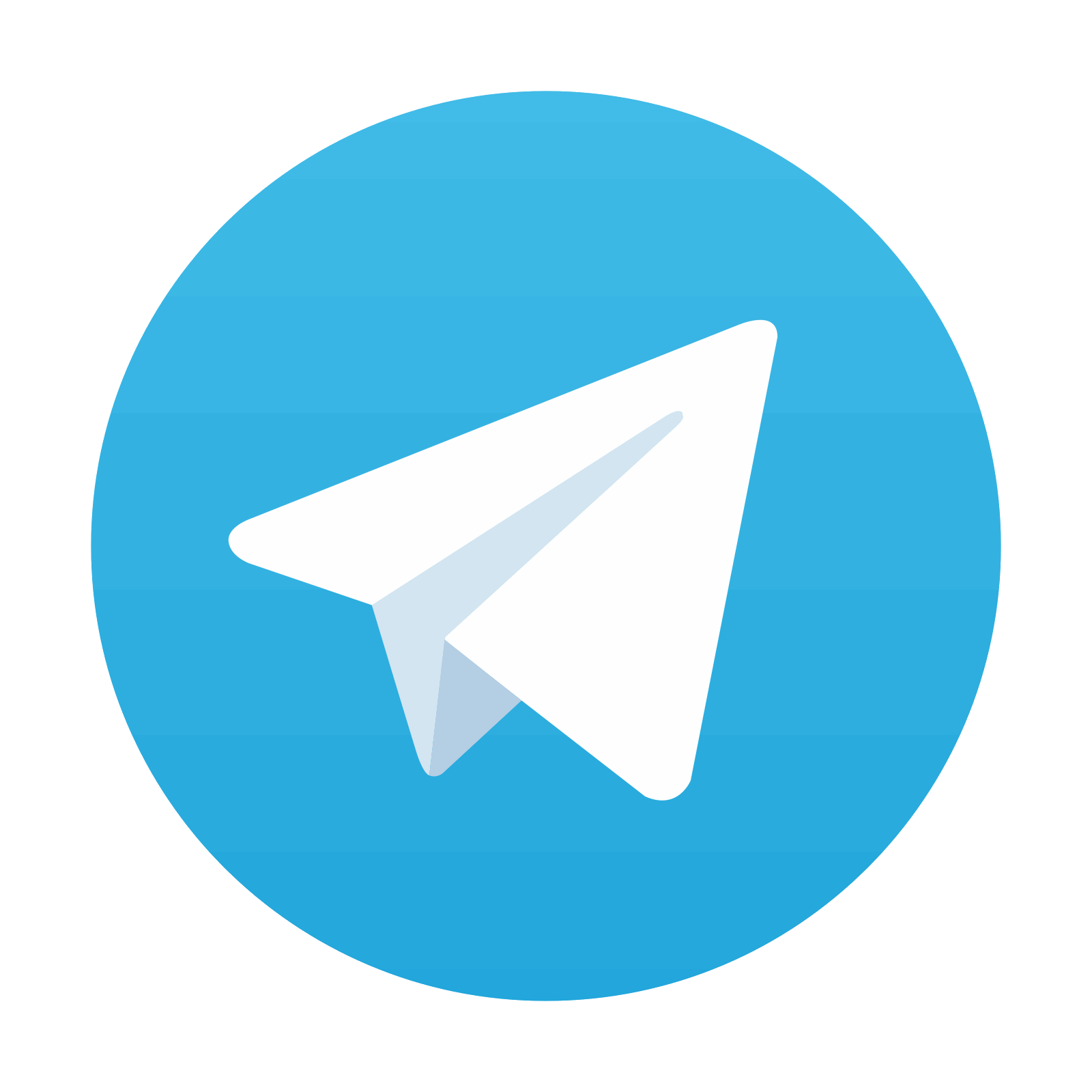
Stay updated, free articles. Join our Telegram channel

Full access? Get Clinical Tree
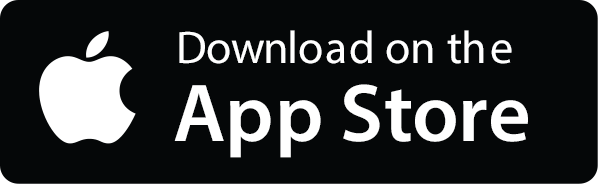
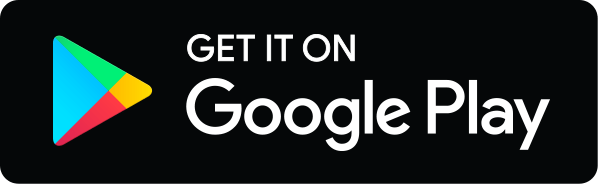