–
pN0
pN+
RT
No RT
RT
No RT
10-year any recurrence
15.6 %
31.0 %
42.5 %
63.7 %
Absolute gain
15.4 %
21.2 %
2p < 0.00001
2p = 0.00001
15-year any death
30.4 %
33.2 %
47.7 %
58.4 %
Absolute gain
2.8 %
10.7 %
n.s.
2p = 0.005
15-year BC mortality
17.2 %
20.5 %
42.8 %
51.3 %
Absolute gain
3.3 %
8.5 %
2p = 0.005
2p = 0.01
Elderly Patients
In the above cited meta-analysis, women who were 60–69 years old had an absolute gain in 10-year any recurrence rate of 14.1 % (2p = 0.00001) and patients >70 years still 8.9 % (2p = 0.00002) (Darby et al. 2011). No subgroup has yet been identified in whom patients did not profit from RT after BCS in terms of improved local tumor control. Hence, omission of RT in patients of advanced age even with favorable prognostic factors (pN0, ER positive, low grade) should only be considered in the presence of comorbidities with a substantial reduction of life expectancy (Belkacémi et al. 2011; Biganzoli et al. 2012; S3 2012; Sautter-Bihl et al. 2012).
17.1.2 Treatment Planning and Technique for WBI
For planning and treatment, patients are usually placed in supine position with elevation of the ipsilateral or both arms in immobilization cradles. Prone position may be an alternative for some patients with regard to sparing normal tissue (Kirby et al. 2011; Leonard et al. 2012). Individual CT-based 3-D planning is mandatory. Several anatomically based instruction guidelines have been published for the definition of the planning target volume (PTV) (Nielsen et al. 2013; RTOG 2014). The PTV includes the mammary gland and the adjacent chest wall. Organs at risk (OAR) like the lung, heart, and contralateral breast are routinely contoured and the esophagus or spinal cord when indicated.
Detailed instructions for contouring of the heart are provided by the atlas of Feng et al. (Feng et al. 2011). Contouring of the whole heart should start directly inferior to the left pulmonary artery. The pericardium should be included as cardiac vessels run within the fatty tissue. Inferiorly, the heart blends with the diaphragm. One of the clinically most relevant structures for late toxicity is the left anterior descending artery (LAD) (Fig. 17.1a, b), as it is the major coronary vessel in the closest vicinity to left- sided tangential RT fields. The LAD originates from the left coronary artery and runs in the interventricular groove between the right and left ventricle and may be difficult to identify without contrast enhancement in the planning CT (Feng et al. 2011).
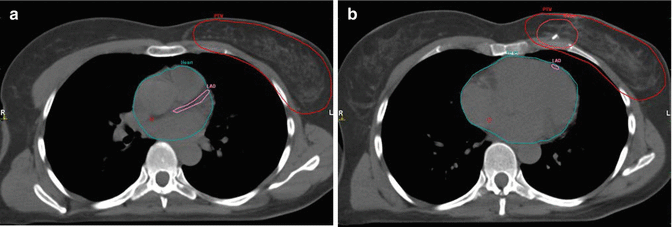
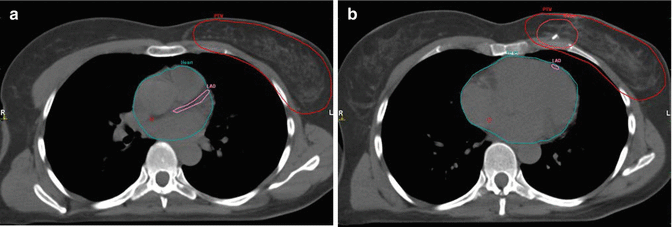
Fig. 17.1
(a, b) Whole breast irradiation: contouring of the planning target volume (PTV), breast and tumor bed with clip, and the heart and left anterior descending artery (LAD)
Homogeneity requirements in dose distribution as described by the ICRU reports 50 and 62 are usually achieved by conformally shaped tangential wedged field techniques, mostly with photons at energies of 4–8 MV. For larger breasts, higher energies and/or intensity modulation (IMRT) within the tangential beams may be appropriate to fulfill the minimum homogeneity criteria (Barnett et al. 2012; ICRU 1993, 1999). However, IMRT increases low-dose exposure to a larger volume of normal tissue and contralateral breast (Lohr et al. 2009). Therefore, sparing of OAR must be weighed against a potentially increased risk of secondary tumor induction (Donovan et al. 2012; Hall and Wuu 2003), and the routine use of multiangle IMRT techniques is critical (Fig. 17.2a, b). However, IMRT may be beneficial for patients with difficult anatomical conditions (i.e., funnel chest) where tangential field arrangements would lead to excessive exposure of OARs. In these cases, WBI in prone position may provide an alternative. For left-sided RT, the use of active breathing control such as computer-assisted breath hold in deep inspiration (Swanson et al. 2013; Zurl et al. 2010) provides another possibility of sparing cardiac dose exposure (Fig. 17.3a–d) as it increases the distance between the target volume and heart (Lin et al. 2008).
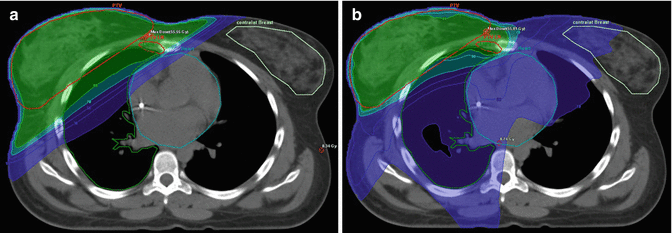
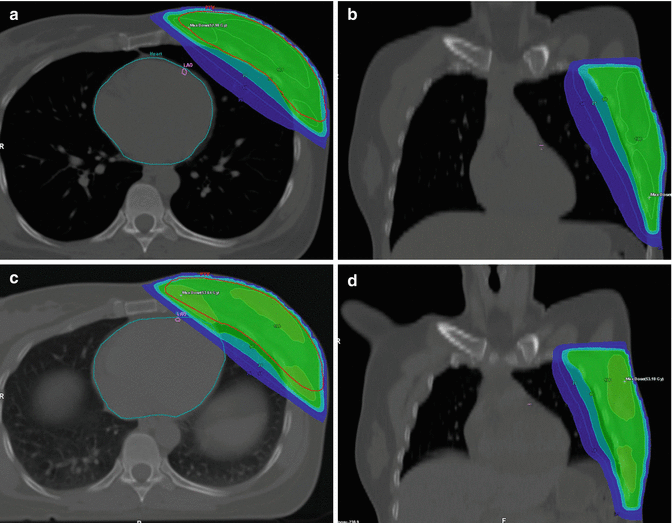
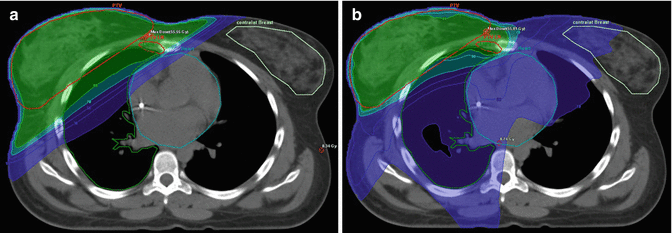
Fig. 17.2
(a, b) Internal mammary node irradiation (IMN-RT): comparison of the dose distribution with 3-D tangential fields (a) versus multiangle IMRT (b); reduced lung dose, increased low-dose exposure to a larger volume of normal tissue and contralateral breast
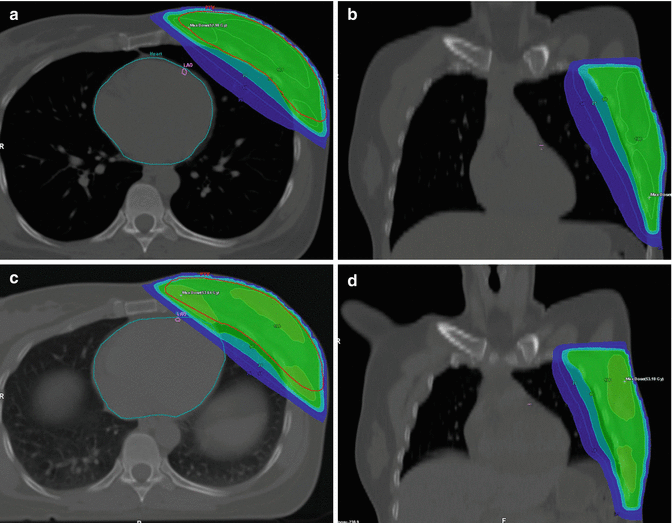
Fig. 17.3
(a–d) Whole breast irradiation (WBI): comparison of the dose distribution to the heart, left anterior descending coronary artery (LAD), and lung with (a, b) and without (c, d) active breathing control (ABC). ABC increases the distance between the heart and LAD to the treatment volume
The doses for target volume and OAR are documented in dose-volume histograms (DVH). For quality control, repeated imaging (mostly by electronic portal imaging or kV-based scans) should be performed to verify and document correct field setup.
17.1.3 Dose and Fractionation for WBI
Conventional fractionation (CF) with a total dose of 50–50.4 Gy in single fractions of 1.8–2 Gy is still regarded as standard in many current guidelines (Belkacémi et al. 2011; NCCN 2014; S3 2012; Sedlmayer et al. 2013a). Divergence exists concerning the appraisal of hypofractionation (HF) as a routinely applicable alternative (NCCN 2014; NICE 2009) or as an option restricted to selected patients (S3 2012; Smith et al. 2011). The ASTRO consensus (Smith et al. 2011) and the German S3 guidelines (S3 2012) additionally recommend to refrain from HF when a homogenous dose is not achievable and in patients who need a boost. In the practical guidelines of the DEGRO, hypofractionated WBI with single doses up to 2.7 Gy in 15–16 fractions to total doses of 40–42 Gy is stated as an option for older women with pT1-2 pN0 tumors who need no chemotherapy. The additional use of a sequential boost is possible (Sedlmayer et al. 2013a).
One critical structure for potential late toxicity of RT is the heart. As late cardiac toxicity of HF is not yet been sufficiently evaluated in long-term follow-up and the latency for clinical manifestation of cardiovascular effects may be 15 years or longer (Harris et al. 2006), HF might be critical in cases of relevant dose exposure to the heart, especially in women with a longer life expectancy.
Body of Evidence for Hypofractionation (HF)
Four randomized clinical trials (Bentzen et al. 2008a, b; Haviland et al. 2013; Owen et al. 2006; Whelan et al. 2010) investigated HF schedules (39–42.9 Gy in single fractions of 2.6–3.3 Gy) for iso-effectiveness compared to conventional fractionation (CF) (50 Gy à 2 Gy), three of those in the last decade. In a Cochrane meta-analysis, published in 2010, these four studies were classified as low to medium quality (James et al. 2010). The authors summarized their findings to provide reassurance that offering HF to carefully selected patients was unlikely to be detrimental in terms of local control, breast appearance, survival, or late radiation breast toxicity for women with small to medium breasts and older than 50 years with small, node-negative tumors.
Longer follow-up was then provided in 2013, when the updated results of the two START trials were published (Haviland et al. 2013). Overall, 4,451 women with invasive breast cancer (pT1–3a, pN0–1, M0) were enrolled. Primary endpoints were locoregional recurrence, late normal tissue effects, and quality of life.
For START A 2,236 women were randomly assigned for CF with 50 Gy in 25 fractions over 5 weeks compared with 41.6 or 39 Gy in 13 fractions over 5 weeks. Median age was 57 years, 85 % had BCS, 51 % had tumors smaller than 2 cm, 29 % had positive lymph nodes, 70 % had grade 1 or 2 disease, 35 % received adjuvant chemotherapy, 14 % received lymphatic radiotherapy, and 61 % had an additional boost (5 × 2 Gy). The 10-year rates of local-regional relapse did not differ significantly between the 41.6 and 50 Gy regimen groups (6.3 % vs. 7.4 %) or the 39 and 50 Gy regimen groups (8.8 % vs. 7.4 %). Distant relapses, disease-free survival, and overall survival were not significantly different between schedules as compared with 50 Gy (Haviland et al. 2013). START B: 2,215 women were recruited, a regimen of 50 Gy in 25 fractions over 5 weeks was compared with 40 Gy in 15 fractions over 3 weeks, and median follow-up was 9.9 years. Median age was 57 years, 92 % had BCS, 64 % had tumors smaller than 2 cm, 23 % had positive lymph nodes, 75 % had grade 1 or 2 disease, 22 % received adjuvant chemotherapy, 7 % received lymphatic radiotherapy, and 43 % patients had an additional boost of 5 × 2 Gy. The proportion of patients with local-regional relapse at 10 years did not differ significantly between the 40 Gy group (4.3 % vs. 5.5 %) and the 50 Gy group. In contrast to START A, the 10-year rate of distant metastases was 12.3 % for HF vs. 16 % for CF (p = 0.01); all-cause mortality was 15.9 % for HF vs. 19.2 % for CF (p = 0.042). A difference of about 3 % for DFS and OAS had already been described after 5 years in the START B group; according to the authors, it is unexpected and not attributable to differences in local control and remains unexplained in the 10-year update (Haviland et al. 2013).
Cosmesis
Cosmesis was evaluated in about half of the START patients. Photographs were taken before RT and at 2 and 5 years; however an unspecified number had no 5-year photographic assessment. After 5 years, a quality of life study was performed in half of the patients of both studies (2,208 women). Patient-reported symptoms were moderate or marked changes in breast appearance in about 40 %, breast shrinkage in one fourth, and moderate or marked pain in the arm and shoulder in one third of the participants. Change in skin appearance was less in the HF arms (28 % vs. 23 % in START B) (Hopwood et al. 2010). These patient-reported results were not updated in the 10-year analysis; the rate of physician-reported breast shrinkage in the 10-year update was 31.2 % for CF vs. 26.2 % for HF (p = 0.015); of breast induration, 17.4 % CF vs. 14.3 % HF (n.s.); and of breast edema, 9.0 % vs. 5.1 % (n.s.) (Haviland et al. 2013).
In the Canadian study (Whelan et al. 2010), 1,234 patients with pT1-2 node-negative tumors, a breast size <25 cm (measured as width at the posterior border of the medial and lateral tangential beams), were randomized for CF (50 Gy in fractions of 2 Gy) vs. HF (42.5 Gy in fractions of 2.6 Gy); in both arms, no boost was used, no lymph node irradiation was performed, and chemotherapy was applied in 10.9 %. The majority (76 %) were >50 years. In the whole group, the 10-year LRR was similar (6.2 vs. 6.7 %) with the exception of poorly differentiated tumors where HF provided an inferior tumor control (10-year LRR 15.6 % for HF vs. 4.7 % with CF, p = 0.01). No difference in survival was observed.
Cosmesis
It was evaluated by experienced nurses, and the 10-year follow-up revealed fair or poor results in one third, good results in 39 %, and excellent results in 30 % for both groups (Whelan et al. 2010).
Comments and Conclusions
HF appears as a safe treatment concerning oncologic outcome and non-inferior regarding cosmesis. So far, data from the randomized studies refer to women mostly >50 years, predominantly with small- to medium-sized breasts and node-negative tumors. The question remains whether these results are assignable to all women with early breast cancer, especially those of younger age, large breasts, and positive nodes and the large group of women receiving chemotherapy with modern combinations. Changes in breast appearance were reported to be no worse than with CF; however, uncertainty remains whether a rate of about one third fair and poor results is an adequate benchmark nowadays.
17.2 Boost
17.2.1 Indications for Boost RT
An additional boost to the tumor bed improves local control and is therefore generally recommended (Belkacémi et al. 2011; NCCN 2014) with the exception of postmenopausal patients with very low-risk tumors (in particular >60 years of age, small tumors, good prognostic features). The absolute benefit of the boost is smaller in this subgroup; therefore its omission may be considered.
Body of Evidence
Two large randomized studies evaluated local tumor control of WBI alone vs. WBI plus boost. The EORTC trial (Bartelink et al. 2007) comprised 5,318 patients, and the 10-year LRR was 10.2 % for the boost group and 6.2 % for patients with WBI alone (hazard ratio (HR) 0.59, 0.46–0.76). LRR was about halved in all age groups though the absolute reduction was smaller in older patients (Antonini et al. 2007). The boost technique (electrons, external beam photons, or brachytherapy) had no significant impact on the oncologic outcome. The reduction of LRR did not translate into improved survival in this trial. Similar results were achieved in the Lyon Trial (Romestaing et al. 1997); however, no update of these 5-year results has been published.
17.2.2 Treatment Planning and Techniques of Boost RT
The PTV encompasses the tumor bed including seroma and a safety margin (Fig. 17.4). The width of the margin varies from 5 to 30 mm (Nielsen et al. 2013; Poortmans et al. 2009; van der Laan et al. 2010).
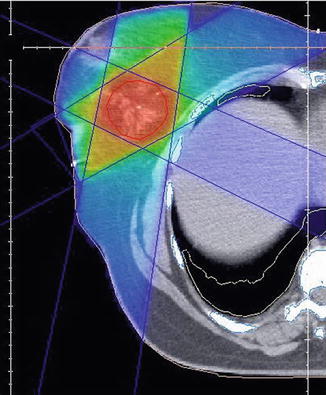
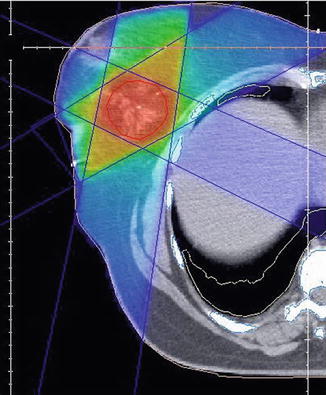
Fig. 17.4
Boost RT: dose distribution 3-D conformal technique, photons. Clip inside the tumor bed for better localization of boost region
The following techniques are used for boost treatment: external beam photons and electrons (Bartelink et al. 2007), interstitial brachytherapy (Polgár and Major 2009), endoluminal brachytherapy (Shah et al. 2010), and intraoperative radiotherapy with electrons (IOERT) (Fastner et al. 2013) or low-energy photons (kV) (Vaidya et al. 2011). Dose distribution is substantially different for these techniques; therefore, outcome analyses of local control rates have to take account for the limited biological comparability of dose specifications, according to the respective method (Jalali et al. 2007; Nairz et al. 2006).
17.2.3 Dose and Fractionation of Boost RT
For an external beam boost, a dose of 10–16 Gy in 5–8 fractions is commonly recommended (Belkacémi et al. 2011; NCCN 2014; New Zealand Guidelines Group (NZGG) 2009; S3 2012) following WBI.
For the simultaneously integrated boost, single fractions of 2.1–2.25 Gy are applicable (Sedlmayer et al. 2013b).
For brachytherapy and IORT, varying doses are applied according to the respective method (see below).
Body of Evidence
In the EORTC boost trial, a dose of 16 Gy was used either in 8 fractions of 2 Gy EBRT or as low-dose-rate brachytherapy using iridium-192 implants with a dose rate of 0.5 Gy per hour. In the French randomized trial, Romestaing et al. (Romestaing et al. 1997) used 10 Gy in fractions of 2 Gy; updated results have not been published. A subgroup analysis of the EORTC boost trial (Poortmans et al. 2009) assessed the outcome of 251 patients with R1 resection who were randomized to receive either a 10 Gy or a 26 Gy boost. There was no statistically significant difference in local control or survival between the lower and the higher boost dose group. Fibrosis occurred significantly more often after 26 Gy. Extrapolating these results on patients with complete resection, it seems justifiable to use a 10 Gy boost, even though data are less valid than for 16 Gy (S3 2012). In a European survey about current practice in breast RT, one third of the institutions used a 10 Gy boost dose (van der Laan et al. 2010).
Recently, several studies evaluated the technique of a simultaneously integrated boost which is administered by daily zonal dose augmentations (Bantema-Joppe et al. 2012; Hurkmans et al. 2006; Van Parijs et al. 2012). Long-term data are not yet available, but radiobiological considerations suggest that single tumor bed doses of 2.1 Gy for low-risk tumors up to 2.25 Gy for constellations with higher risk for local recurrence seem to be within the acceptable range. Outside clinical trials, SIB technique with HF is discouraged (Sedlmayer et al. 2013b).
17.3 Accelerated Partial Breast Irradiation (APBI)
17.3.1 Indications for APBI
Accelerated partial breast irradiation (APBI) refers to RT of a smaller (partial) breast volume over a shorter treatment time, covering the tumor bed with a limited margin of normal tissue. APBI can be delivered intraoperatively in a single fraction or postoperatively over 1–3 weeks by brachytherapy or external beam radiotherapy.
For patients at a lower risk for local recurrence, APBI has been strongly propagated in the last years as it spares treatment time and costs and allegedly provides less toxicity, however possibly on the expense of deteriorated local control (Valachis et al. 2010).
There is no general consensus regarding the indication for using ABPI as a sole treatment for breast cancer. While the ASTRO and GEC-ESTRO permit APBI for selected patients (Polgár et al. 2010; Smith et al. 2009), the German interdisciplinary guidelines do not recommend APBI outside clinical trials (S3 2012), while the DEGRO practical guidelines (Sedlmayer et al. 2013a) regard ABPI with IORT or brachytherapy as an option for patients >70 years with node-negative T1, luminal A tumors.
Body of Evidence
A meta-analysis of these trials revealed an increased risk for both local (odds ratio (OR) 2.15; p 0.001) and regional recurrences (OR 3.43; p < 0.001) for APBI compared to WBI, however, not yet translating in a survival difference (OR 0.91; p 0.55) (Valachis et al. 2010).
TARGIT-A was a randomized, non-inferiority trial (Vaidya et al. 2014). Women aged 45 years and older with invasive ductal carcinoma were enrolled and randomly assigned to receive intraoperative radiotherapy (IORT) (n = 1,721) or WBI with 50 Gy in fractions of 2 Gy.whole breast EBRT (n = 1,730). For IORT, a point source of 50 kV energy x-rays at the center of a spherical applicator is used, delivering a dose of 20 Gy to the surface of the tumor bed that attenuates to 5–7 Gy at 1 cm depth.
Randomization occurred either before lumpectomy (prepathology stratum, i.e., IORT concurrent with lumpectomy) or after lumpectomy (postpathology stratum, i.e., IORT given subsequently by reopening the wound). Of the group randomized for IORT, 15.2 % received supplemental WBRT if unforeseen adverse features were detected on final pathology but counted as APBI according to the intent-to-treat principle. The primary endpoint was the absolute difference in local recurrence in the conserved breast, with a non-inferiority margin of 2.5 % at 5 years. The median follow-up of all patients was 29 months. The 5-year risk for in-breast recurrence was 3.3 % for APBI vs. 1.3 % for EBRT (p = 0.042). Overall, breast cancer mortality was similar between groups (2.6 % for APBI vs. 1.9 % for WBRT; p = 0.56). Toxicity was comparable, and grade 3 or 4 skin complications were less frequent with IORT (0.2 %) vs. EBRT (0.7 %, p = 0.029).
In the ELIOT trial (Veronesi et al. 2013), 1,305 women aged 48–75 years with early breast cancer, a maximum tumor diameter of up to 2.5 cm, were randomly assigned to WBRT (n = 654) with 50 Gy plus boost or intraoperative radiotherapy with electrons (IOERT) with a single dose of 21 Gy to the tumor bed during surgery (n = 651). The primary endpoint was occurrence of ipsilateral breast tumor recurrences (IBTR); overall survival was a secondary endpoint; the main analysis was by intention to treat.
After a medium follow-up of 5.8 years, 35 patients in the intraoperative radiotherapy group and four patients in the external radiotherapy group had had an IBTR. The 5-year event rate for IBRT was 4.4 % in the IOERT group and 0.4 % in the WBRT group (p < 0.0001). The 5-year OAS was identical (96.8 %). The authors state that they observed significantly (p = 0.0002) fewer skin side effects with IOERT. In patients with data available (n = 876), acute skin toxicity was 6.9 % with WBI vs. 1.2 % with IOERT; however, chronic skin toxicity was observed in 5 of 464 patients after WBI (1.1 %) and 6 of 412 patients (1.5 %) with IOERT (Veronesi et al. 2013).
Comments and Conclusion
The available data strongly indicate an increased risk of in-breast recurrence for patients treated with APBI alone, while toxicity is comparable. The question remains whether even a small reduction of local control appears justifiable in patients with early breast cancer, who have an excellent prognosis with standard WBI (Moser and Vrieling 2012; Sautter-Bihl et al. 2010). Of note, long-term results of APBI studies are mandatory for final assessment of its effectiveness as true local recurrences are presumed to occur between 40 and 65 months after primary treatment and out-quadrant relapses even later (Brooks et al. 2005; Freedman et al. 2005; Gujral et al. 2011).
17.3.2 Technique and Dose of APBI
The following techniques are used: external beam RT (Lewin et al. 2012; Vicini et al. 2010), interstitial brachytherapy (Arthur et al. 2008; Ott et al. 2007), endoluminal brachytherapy, and intraoperative radiotherapy with electrons (Veronesi et al. 2010) or low-energy photons (Vaidya et al. 2010). Dose distribution is substantially different for these techniques; therefore, outcome analyses of local control rates have to take account for the limited biological comparability of dose specifications, according to the respective method (Sautter-Bihl et al. 2010).
17.4 Postmastectomy Radiotherapy (PMRT)
17.4.1 Indications for Postmastectomy Radiation (PMRT)
While the indication for PMRT is undisputed for women with T4 tumors and ≥4 affected nodes, it has been an issue of debate for those with 1–3 positive nodes. Recent data confirm the benefit of PMRT for pN1 patients (EBCTCG). Moreover, PMRT should be considered in node-negative patients with T3 tumors in case of additional risk factors such as lymphovascular invasion, blood vessel invasion, positive node ratio >20%, resection margins <3 mm, grading 3, young age/premenopausal, extracapsular invasion, negative hormone receptors, invasive lobular cancer, or a tumor size >2 cm.
Body of Evidence
The latest meta-analysis of the Early Breast Cancer Trialists’ Collaborative Group (EBCTCG) 2014) confirmed the effectiveness of PMRT for women with 1–3 positive nodes as described by the Danish Breast Group and the British Columbia trial (Overgaard et al. 2007; Ragaz et al. 2005). The EBCTCG analyzed data of 8,135 women in 22 randomized trials (treated before 2000), comparing PMRT to the chest wall and regional lymph nodes after mastectomy and axillary surgery versus the same surgery without radiotherapy. Of those, 3,786 women had axillary lymph node dissection (ALND) to at least level II and/or a median number of at least 10 removed nodes. Less extensive surgery (n = 4,065) was classified as axillary sampling (AS). In 183 patients the extent of surgery was not specified. Analyses were stratified by trial, individual follow-up year, age at entry, and pathological nodal status. Overall, 5,821 patients (72 %) were node positive, of those 54 % had ALND and 44 % had AS, whereas in the 1,594 node-negative women, 44 % had ALND and 55 % underwent AS. After complete axillary dissection, node-negative patients did not profit from PMRT, neither in terms of recurrence nor survival. By contrast, for the 870 women with node-negative disease who underwent axillary sampling only, PMRT provided a fivefold reduction of local recurrence (16.3 vs. 3.3 % p < 0.00001); moreover, any recurrence was reduced by 12.1 % (34.2 vs. 22.1 %, p < 0.00001). However, no significant difference in survival was observed (Table 17.2).
Table 17.2
Results of the EBCTCG 2014 comparing postoperative radiotherapy after mastectomy (PMRT) vs. none
– | 1–3 LK | 4+ LK | ||
---|---|---|---|---|
RT | No RT | RT | No RT | |
10-year LRR | 3.8 % | 20.3 % | 13.0 % | 32.1 % |
Absolute gain | 16.5 % | 19.1 % | ||
2p < 0.00001 | 2p < 0.00001 | |||
10-year any recurrence | 34.2 % | 45.7 % | 66.3 % | 75.1 % |
Absolute gain | 11.5 % | 8.8 % | ||
2p 0.00006 | 2p 0.0003 | |||
20-year BC mortality | 42.3 % | 50.2 % | 70.7 % | 80 % |
Absolute gain | 7.9 % | 9.3 % | ||
2p = 0.01 | 2p = 0.04 |
Among the 1,314 women who had axillary dissection and only one to three positive nodes, radiotherapy reduced locoregional recurrence (2p < 0.00001), overall recurrence (RR 0.68, 95 % CI 0.57–0.82, 2p = 0.00006), and breast cancer mortality (RR 0.80, 95 % CI 0.67–0.95, 2p = 0.01).
The absolute gain for irradiated women was comparable between pN1-3 and pN4+ patients: 10-year LC 16.5 % ( pN1-3) and 19.1 % (pN4+) in favor of the irradiated group. In terms of overall recurrence, the absolute benefit was 11.5 % (pN1-3) and 8.8 % (pN4+). PMRT provided an absolute reduction of 20-year breast cancer mortality of 7.9 % (2p = 0.01) for pN1-3 and 9.3 % (2p = 0.04) for pN4+ patients. These effects were similar whether patients received systemic treatment or not.
Comments and Conclusion
The available evidence strongly suggests to offer PMRT to all node-positive patients.
17.4.2 Treatment Planning, Technique, and Dose for PMRT of the Chest Wall
Three-dimensional CT planning is mandatory. The PTV comprises the chest wall including the scar and a safety margin. The cranial and caudal field borders are adapted to the size and position of the contralateral breast with the inferior extension about 1.5 cm below the original submammary fold. For sparing of the OAR, the same technical options apply as described for WBI in Sect. 17.1.2. However, the target volume of PMRT differs substantially from WBI as it is usually more flat shaped and includes the chest wall more generously (Fig. 17.5). IMRT may be helpful, especially for left-sided cancer (Rudat et al. 2011).
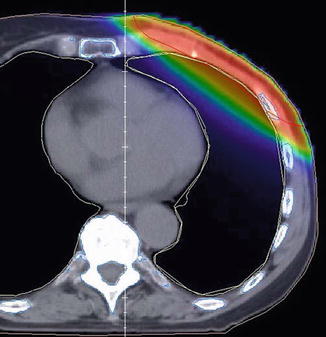
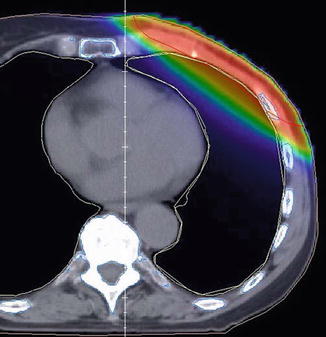
Fig. 17.5
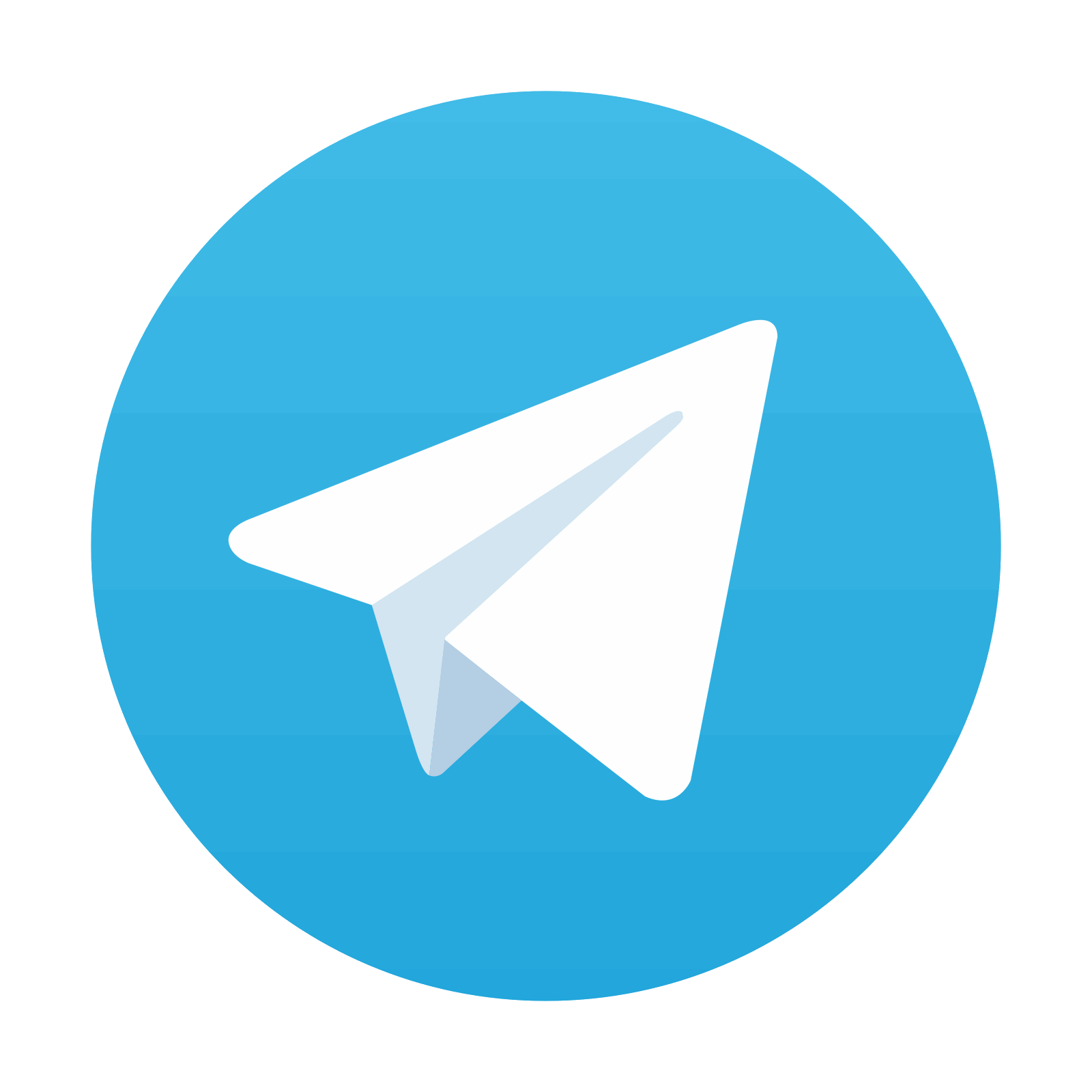
Postmastectomy radiotherapy (PMRT): dose distribution to the heart and lung in deep breath hold
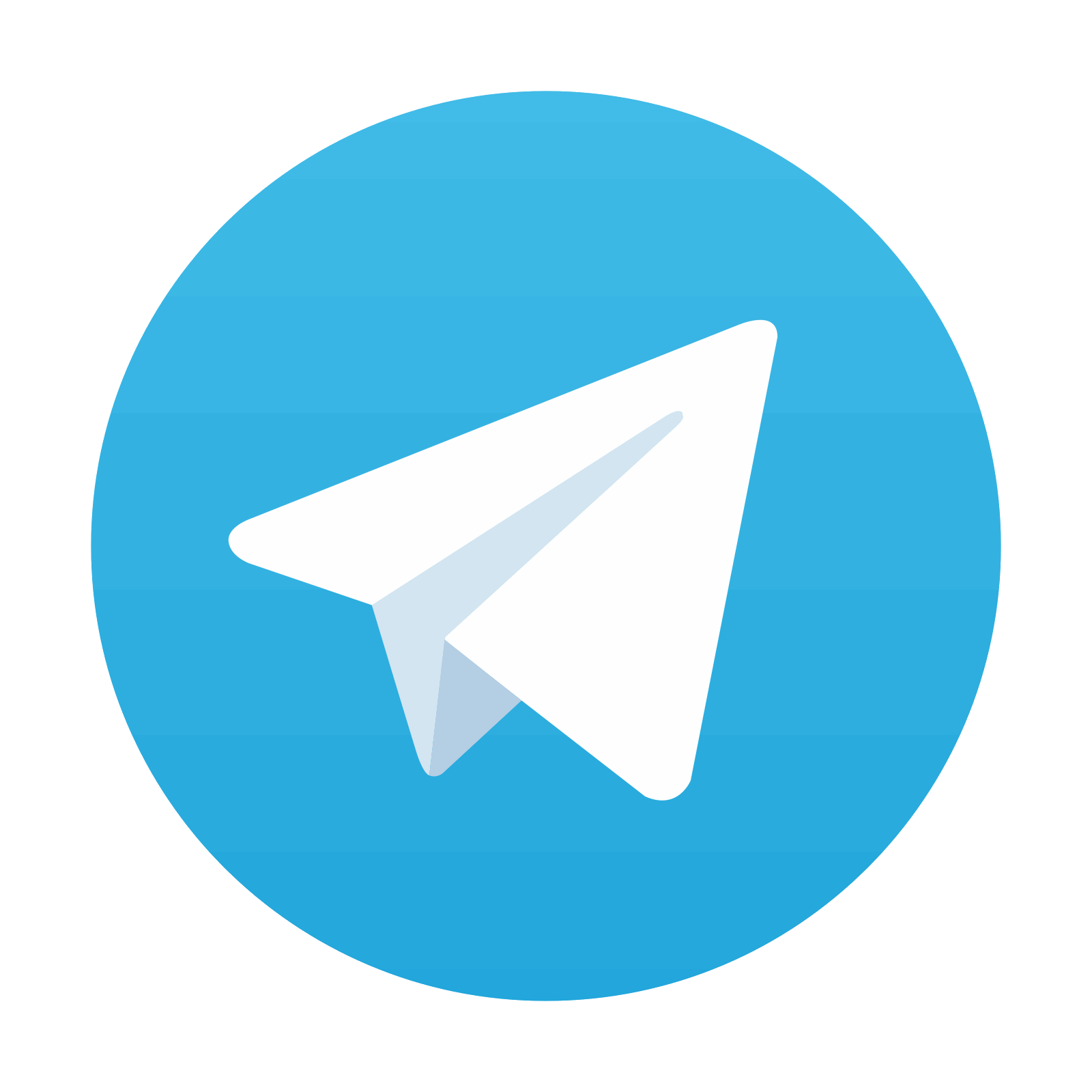
Stay updated, free articles. Join our Telegram channel

Full access? Get Clinical Tree
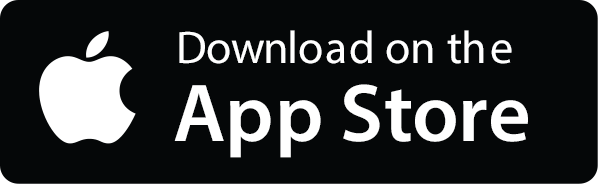
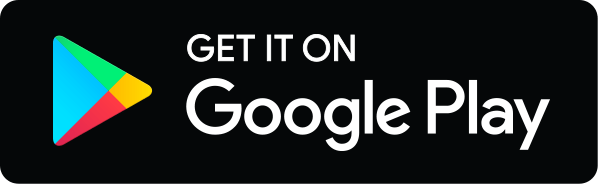
