Celia L. Gregson
Bone and Joint Aging
The musculoskeletal system serves three primary functions: (1) it enables an efficient means of limb movement; (2) it acts as an endoskeleton, providing overall mechanical support and protection to soft tissues; and (3) it serves as a mineral reservoir for calcium homeostasis. In older adults, the first two of these functions frequently become compromised; musculoskeletal problems are a major cause of pain and physical disability in older adults and represent a significant contributor to the global burden of disease.1 Furthermore, fracture incidence rises steeply with age2 (Figure 20-1). Several factors contribute to the age-related decline in musculoskeletal function:
A number of interrelated hypotheses have been proposed to explain the high prevalence of bone, muscle and joint problems in older adults3–6:
Several different mechanisms are involved in musculoskeletal tissue aging, including the following7–10:
• Decline in mesenchymal stem cell (MSC) populations
• Changes in posttranslational modification of structural proteins such as collagen and elastin
• Aberrant epigenetic modification altering cell regulation
• Induction of inflammatory mediators with accumulation of proinflammatory cytokines
• Alterations in the loading patterns of tissue or the tissue’s response to loading
The major tissues pivotal to the integrity of the musculoskeletal system are articular cartilage, skeleton, and soft tissues. Age-related changes in these structures will now be described in more detail.
Articular Cartilage
The structure of a mammalian synovial joint is summarized in Figure 20-2. Much of its function derives from the properties of articular cartilage, which cushions the subchondral bone beneath and provides a low-friction surface necessary for free movement. Articular cartilage contains very few cells, is aneural and avascular, and yet in health its integrity is maintained throughout a lifetime of biomechanical stress. A certain amount of mechanical loading is known to be necessary for cartilage homeostasis, because joint damage develops following immobilization.11 The chief cells in cartilage are chondrocytes; the extracellular matrix is composed principally of type II collagen and aggrecan (aggregating proteoglycans). Collagen molecules consist of a triple helix of three polypeptide chains, cross-linked to form collagen fibrils, which are bound to hyaluronic acid and aggrecan and form a network of collagen fibrils.12,13 Aggrecan has many glycosaminoglycan side chains, which help retain water molecules within the matrix.13 Over two thirds of the articular cartilage weight is water, and this high water content is vital to maintain the tissue’s viscoelastic properties.12 The collagen fibrillar network confers tensile strength to the articular cartilage, whereas aggrecan produces stiffness under compression.12,13
With age, articular cartilage thins and changes color from a glistening white to a dull yellow, and its mechanical properties deteriorate. There is a decrease in tensile stiffness, fatigue resistance, and strength, but no significant change in its compressive properties; these changes are partly caused by a decrease in water content. The morphology and function of the chondrocytes and nature of aggrecan and type II collagen also change with age. Osteoarthritis (OA) is the name given to a number of characteristic pathologic changes occurring in synovial joints and adversely affecting joint function. OA is thought to arise when there is an imbalance between the mechanical forces acting on or within a joint and the ability of the articular cartilage and other joint tissues to withstand these forces. Damage can be caused by abnormal mechanical forces acting on normal joint tissues or by normal forces acting on already damaged or abnormal tissues.14 Although OA is not an inevitable consequence of aging, aging adds to the risk of developing OA because it is associated with a number of joint changes affecting all the different joint tissues (Figure 20-3).

The chondrocyte’s principal function is to maintain cartilage homeostasis. However, with age, chondrocytes develop a senescent phenotype with impaired synthetic activity such that the proteoglycans they produce become small and irregular. The response by chondrocytes to changes in anabolic and catabolic stimuli (e.g., IGF-1, osteogenic protein-1, transforming growth factor-β [TGF-β], interleukins [ILs]) tips the balance toward catabolism, which increases OA susceptibility.7 In OA, excess catabolic activity disrupts cartilage homeostasis, causing cartilage matrix breakdown, principally orchestrated by proinflammatory cytokines and catabolic mediators (e.g., MMPs [matrix metalloproteinases]) and ADAMTS (a disintegrin and metalloproteinase with thrombospondin motifs). Replicative senescence, due to telomere shortening with consequent telomere dysfunction, may contribute to chondrocyte aging. However, slow chondrocyte turnover rates reduce susceptibility. Instead, stress-induced senescence, due to telomere damage from oxidative stress, activated oncogenes, mitochondrial dysfunction, and inflammation, is thought to play a greater role. The senescent chondrocytes produce ILs and MMPs, mediating cartilage matrix damage. Autophagy, a homeostatic mechanism of cell recycling that removes damaged and/or redundant organelles and proteins, becomes deregulated in aging cartilage. Excess activation of the protein kinase mammalian target of rapamycin (mTOR), which suppresses autophagy, has been associated with aging. Interestingly, senescent cells, enlarged from accumulated proteins, can be rescued by rapamycin, an mTOR inhibitor.15 Chondrocyte loss can also occur through increased apoptosis, a normal physiologic process involved in the removal of potential carcinogenic and damaged cells. High-mobility group box protein (HMGB2), whose levels decline with age, has emerged as an important regulator of chondrocyte survival.16
Proteoglycan depletion is one of the earliest signs of articular cartilage loss in OA. Proteoglycans consist of a protein core and two major glycosaminoglycan (GAG) side chains, chondroitin sulfate (CS) and keratin sulfate (KS). CS, the predominant GAG chain in human articular cartilage, is made up of oligosaccharide (sugar) chains containing a basic disaccharide repeat of two sugar molecules (N-acetylgalactosamine and glucuronic acid), which carry a sulfate group on the sixth (C6) or fourth (C4) carbon atom. Changes in the C6/C4 sulfation ratio show marked changes with aging and in OA, potentially making the cartilage more susceptible to cytokine-mediated damage.17,18 The main proteoglycan, aggrecan, binds with hyaluronan to form massive hydrophilic aggregates that expand the collagen framework, providing compressive and tensile strength. With age, proteoglycan aggregation reduces, with the synthesis of smaller proteoglycans with increased KS and reduced CS content and increased aggrecanase production, leading to aggrecan degradation.
Collagen also changes with age, with increases in fiber diameter and cross-linking. Fiber cross-linking may be enzymic or nonenzymic; the former process involves the enzyme lysyl hydroxylase. In young growing bone, collagen turnover is high, and enzymic divalent and trivalent cross-links stabilize the collagen fibers, with almost complete hydroxylation of telopeptide lysines. With age, lysyl hydroxylase activity wanes, causing incomplete hydroxylation of telopeptide lysines. However, further increases in collagen fiber cross-linking occur with age due to nonenzymatic reactions between glucose and lysine, forming glucosyl lysine and related molecules. Subsequent oxidative and nonoxidative reactions produce stable end products, known as advanced glycation end products (AGEs), some of which can act as collagen cross-links and produce fibers too stiff for optimal function, making cartilage more vulnerable to mechanical failure.19,20 In chondrocytes, AGEs can suppress type II collagen production, simulate MMP and ADAMTS expression, and increase inflammation through the production of TNF-α (tumor necrosis factor-α), prostaglandin E2, and nitric oxide.21,22 Hyperglycemia and oxidative stress increase AGE production, and dietary AGE intake may also be an important factor.23,24 Elastin, which conveys extensibility and elastic recoil in some ligaments, is also stabilized by cross-linking, and AGE production can also prompt age-related stiffening.25 Accumulation of reactive oxygen species (ROS) in the chondrocyte with aging, due in part to mitochondrial dysfunction, increases oxidative stress, which has a series of consequences, including DNA damage, telomere shortening, loss of anabolic activity, increased production of inflammatory cytokines and MMPs, chondrocyte senescence, and apoptosis.8
As well as changes to the articular cartilage, aging also adversely affects other tissues of the joint. Below the basal layer of articular cartilage (calcified cartilage) lies subchondral bone; an emerging body of evidence has now suggested that the metabolism of cartilage and bone is tightly coupled within joints and that this is important in the pathogenesis of OA.26 Certainly, distinct bone changes are seen as OA progresses—increased subchondral bone turnover, hypomineralization of the underlying trabecular bone, subchondral sclerosis, and the formation of osteophytes and bone marrow lesions. The latter is predictive of the pain of OA.27 Age-related reductions in estrogen, as seen in postmenopausal women, are associated with increases in bone turnover and cartilage degradation.28 Correspondingly, use of estrogen replacement therapy has been associated with a reduced prevalence of OA.29 Studies linking increased rates of bone turnover to OA progression have suggested a role for increased osteoclast activity in the pathogenesis of OA.30 Thus, there is current interest in targeting bone and cartilage with antiresorptive medications,26 although the efficacy of this approach has yet to be demonstrated in humans.31 Further age-related changes within periarticular soft tissue structures that may also adversely affect joint health are discussed later.
Epigenetics in Aging and Osteoarthritis
The role of epigenetic regulation in aging and the cause of OA has been of growing research interest. Epigenetics may explain some of the so-called missing heritability of OA, a disease that can have a strong familial pattern. Epigenetic mechanisms are stable and inherited determinants of gene expression involving no changes in the underlying DNA sequence. They include DNA methylation; histone; modification; and small, noncoding microRNAs (miRNAs). Generally, methylation levels are reduced with age. Hypomethylation of a number of MMP promoters has been seen in cartilage affected by OA.32 Furthermore, histone methylation has been shown to regulate the nuclear factor of activated T cells (NFAT); transcription factors in articular chondrocytes, as an age-dependent mechanism controlling chondrocyte homeostasis that when perturbed, manifests an OA-like phenotype.33 Already a wide variety of miRNAs has been identified, with a range of roles in cartilage and the development of OA.9 Research into the epigenetic mechanisms underlying aging and OA has been gaining momentum, offering the potential for novel insights into the mechanisms of disease, aging, and future therapies.
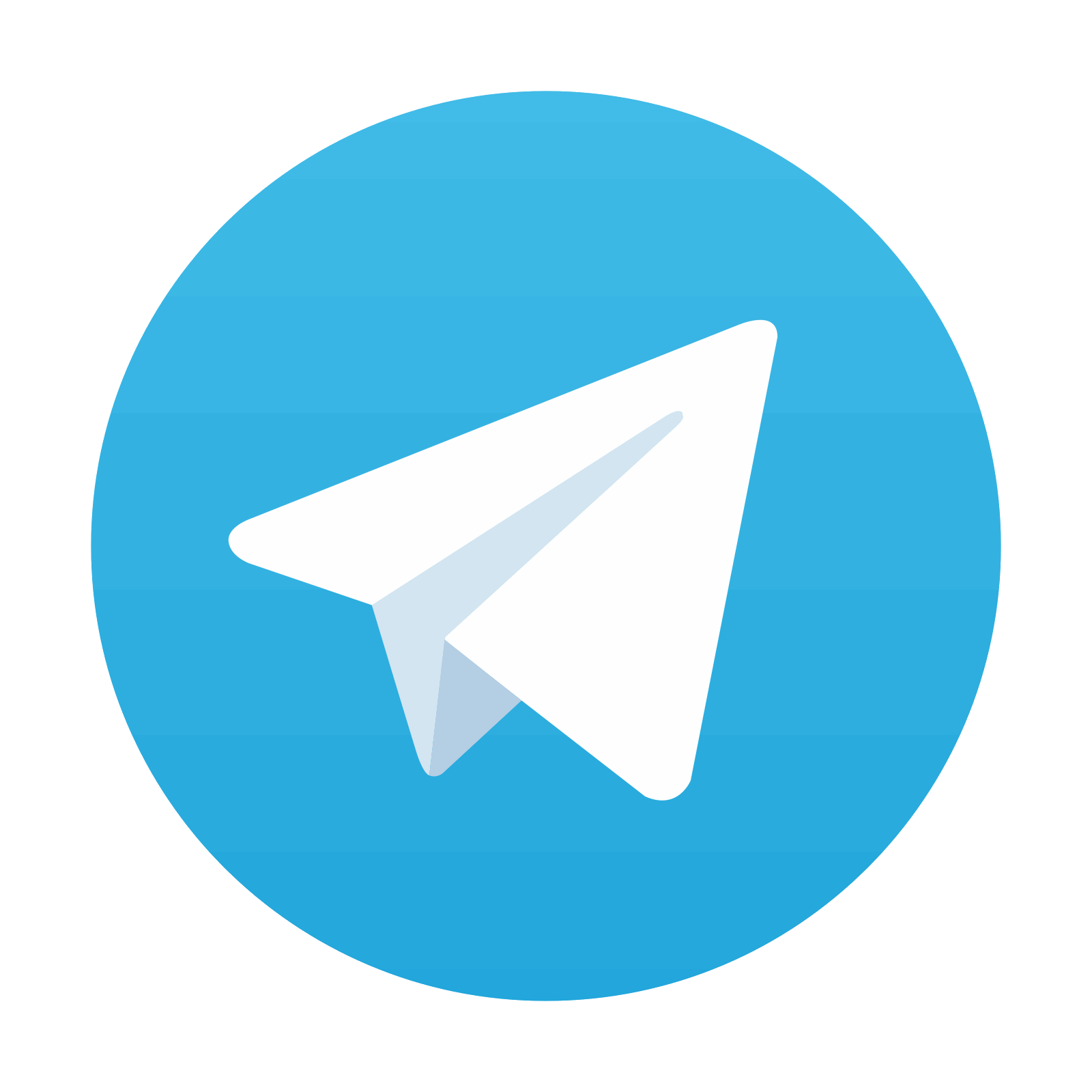
Stay updated, free articles. Join our Telegram channel

Full access? Get Clinical Tree
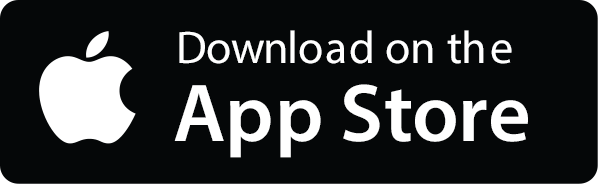
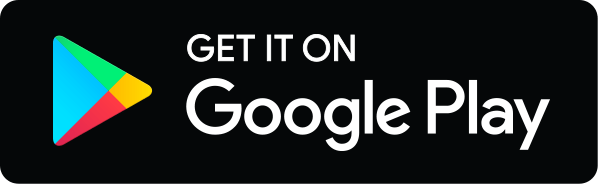