Testicular cancer has been a model for a curable malignancy. The existence of an array of potential new therapies is the result of a prodigious effort in the researching and defining of the molecular components of the cancer phenotype and the subsequent rational design of agents to target candidate pathways. A better understanding of the molecular biology of cancer may also aid in guiding the most appropriate use of existing therapies, such as conventional chemotherapy.
Germ cell tumors are derived from cells belonging to the germ cell lineage. They occur primarily in the gonads, and less than 10% arise in specific extragonadal sites along the midline of the body. This pattern of distribution is thought to reflect the migration route of the primordial germ cells (PGCs) during embryogenesis—from the yolk sac to the genital ridges.
Germ cell tumors of the testis (TGCTs) account for more than 95% of all testicular tumors, and they are the most common solid malignancies to affect young white men. Histologically, TGCTs comprise 2 major subgroups: seminomas and nonseminomatous germ cell tumors (NSGCTs). Seminomas are composed of uniform tumor cells that resemble PGCs/gonocytes. In contrast, NSGCTs may contain one or more histologic subtypes that represent different differentiation lineages and stages of embryonic development—embryonal carcinoma, choriocarcinoma, yolk sac carcinoma, and teratoma. Ten percent of TGCTs comprise elements of both seminomas and NSGCTs.
TGCTs are characterized by extreme sensitivity to chemotherapy. Despite this, approximately 20% of patients with metastatic TGCTs fail to achieve a complete response or relapse from complete remission; this is due to intrinsic cisplatin resistance or acquired resistance after an initial response. Understanding the molecular biology of TGCTs may thus allow the development of new therapies for the small subset of patients with a poor prognosis.
Preinvasive disease
Despite the clinical and histologic differences between seminomas and NSGCTs, all TGCTs are thought to arise from a common precursor lesion, carcinoma in situ, first described by Skakkebaek in 1972. This hypothesis is supported by the frequent observation of carcinoma in situ in the testicular tissue adjacent to TGCTs as well as the development of TGCTs in patients previously diagnosed with carcinoma in situ. Due to the absence of epithelial differentiation in these precursors, the term, intratubular germ cell neoplasia, unclassified (ITGCNU ), is now more commonly used. Spontaneous regression of ITGCNU does not seem to occur and eventually all ITGCNUs progress to TGCTs. The median time to the development of invasive disease is 5 years.
ITGCNUs are thought to be derived from transformation of a PGC or gonocyte during fetal development. Evidence supporting this includes the morphologic similarities and the presence of overlapping developmental immunohistochemical markers (PLAP, KIT, and OCT3/4) between ITGCNUs and PGCs and early gonocytes. In addition, biallelic expression of the imprinted genes, H19 and IGF2 , has been reported in TGCTs, indicating again that these tumors may have arisen from PGCs, where genomic imprinting is temporarily erased. More recently, gene expression profiling studies have revealed a substantial overlap between the expression profile of ITGCNUs and that of embryonic stem cells, providing further evidence of the fetal origin of ITGCNUs.
The precise molecular events underlying the initiation of malignant transformation from gonocyte to ITGCNU remains poorly understood; 2 theories have been put forward. Skakkebaek and colleagues proposed that the origins of ITGCNU are fetal gonocytes that are unable to develop into normal spermatogonia. These arrested germ cells are thought to be susceptible to postnatal or pubertal gonadotrophin stimulation, which may lead to further malignant progression later in development. This model is supported by extensive data suggesting ITGCNU cells are transformed gonocytes. In the second model, Chaganti and Houldsworth postulated that transformation occurs after the onset of spermatogenesis, involving the zygotene-pachytene spermatocyte. These cells contain replicated DNA, express wild-type p53 temporarily, and seem to be associated with a recombination checkpoint. Furthermore, aberrant chromatid exchange events associated with crossing-over during this stage may lead to increased copy number of the short arm of chromosome 12 (12p), consequential cyclin D2 ( CCND2 ) overexpression, and aberrant reinitiation of the cell cycle. This model cannot explain, however, the development of ITGCNU in the gonads of children with sexual differentiation disorders.
Two models have also been proposed to explain the subsequent progression of ITGCNU into seminomas and NSGCTs. In the linear progression model, TGCTs progress along a single pathway, from ITGCNU through seminomas to NSGCTs. Alternatively, the independent progression model postulates that ITGCNU progresses along independent pathways to produce both seminomas and NSGCTs. Considerable evidence exists to support both models and it is likely that both pathways exist.
Genetic changes
TGCTs are characterized by the invariable gain of material from chromosome 12p. In the majority of cases, this is due to an isochromosome of 12p, i(12p), first reported by Atkin and Baker in 1982. This abnormal chromosome comprises 2 fused short arms of chromosome 12 and is common to both seminomas and NSGCTs. The remaining i(12p)-negative TGCTs have also been shown to contain gain of chromosome 12p sequences, either as tandem duplications located in situ or transposed elsewhere in the genome.
In addition to gain of the complete 12p, amplification of subregions of chromosome 12p (12p11.2-p12.1 and 12p12∼13) has also been reported in some TGCTs. The 12p11.2-p12.1 amplification was first identified in a patient with metastatic seminoma and occurs almost exclusively in i(12p)-negative TGCTs. It is associated with a younger age at first presentation compared with seminomas with gain of the complete 12p but does not predict clinical outcome.
Because all TGCTs show gain of chromosome 12p sequences, many investigators have attempted to identify overexpressed genes on 12p, which may be important in the pathogenesis of TGCTs. The growing list of candidate genes can be divided into 2 broad groups: those that confer the tumor cells with a growth advantage (eg, CCND2; K-RAS ; DAD-R, a novel gene associated with decreased apoptosis in TGCTs; and ethanolamine kinase 1 [ EKI1 ], which may protect cells from apoptotic cell death) and those that help to establish or maintain the stem cell phenotype (eg, the stem cell genes NANOG, STELLA , and GDF3 ).
Gain of 12p is also thought to be associated with malignant progression of ITGCNU into invasive TGCTs. Using high-resolution comparative genomic hybridization, Ottesen and colleagues analyzed the microdissected cells from 7 cases of ITGCNU adjacent to TGCTs and from 2 cases of ITGCNU without invasive elements. Gain of 12p was detected in 6 of 7 cases of ITGCNU adjacent to TGCTs but not in the 2 cases of ITGCNU without invasive disease. Nevertheless, gain of 12p is not thought to be the initiating genetic event, because acquisition of i(12p) has been shown to be preceded by polyploidization.
A nonrandom pattern of over-representation and under-representation of other chromosomal regions has also been reported, although at a lower frequency than the gain of 12p. These changes are common to both seminomas and NSGCTs, supporting the hypothesis of a common origin for all histologic subtypes of TGCTs.
Differential chromosomal gains and losses have also been reported between seminomas and NSGCTs. Gain of chromosomes 7, 15, 19, and 22 and loss of chromosome 17 have been associated with seminomas, whereas gain of chromosomes 5q, 6q, 13q, and 17q and loss of chromosomes 10q, 19, and 22 were detected in NSGCTs, suggesting these regions may be important in the differentiation of TGCTs. Several recent studies have attempted to identify differentially expressed genes corresponding to such regions of chromosomal gains and losses. Despite this, few of the identified genes have been shown to contribute to the development or progression of TGCTs.
Genetic changes
TGCTs are characterized by the invariable gain of material from chromosome 12p. In the majority of cases, this is due to an isochromosome of 12p, i(12p), first reported by Atkin and Baker in 1982. This abnormal chromosome comprises 2 fused short arms of chromosome 12 and is common to both seminomas and NSGCTs. The remaining i(12p)-negative TGCTs have also been shown to contain gain of chromosome 12p sequences, either as tandem duplications located in situ or transposed elsewhere in the genome.
In addition to gain of the complete 12p, amplification of subregions of chromosome 12p (12p11.2-p12.1 and 12p12∼13) has also been reported in some TGCTs. The 12p11.2-p12.1 amplification was first identified in a patient with metastatic seminoma and occurs almost exclusively in i(12p)-negative TGCTs. It is associated with a younger age at first presentation compared with seminomas with gain of the complete 12p but does not predict clinical outcome.
Because all TGCTs show gain of chromosome 12p sequences, many investigators have attempted to identify overexpressed genes on 12p, which may be important in the pathogenesis of TGCTs. The growing list of candidate genes can be divided into 2 broad groups: those that confer the tumor cells with a growth advantage (eg, CCND2; K-RAS ; DAD-R, a novel gene associated with decreased apoptosis in TGCTs; and ethanolamine kinase 1 [ EKI1 ], which may protect cells from apoptotic cell death) and those that help to establish or maintain the stem cell phenotype (eg, the stem cell genes NANOG, STELLA , and GDF3 ).
Gain of 12p is also thought to be associated with malignant progression of ITGCNU into invasive TGCTs. Using high-resolution comparative genomic hybridization, Ottesen and colleagues analyzed the microdissected cells from 7 cases of ITGCNU adjacent to TGCTs and from 2 cases of ITGCNU without invasive elements. Gain of 12p was detected in 6 of 7 cases of ITGCNU adjacent to TGCTs but not in the 2 cases of ITGCNU without invasive disease. Nevertheless, gain of 12p is not thought to be the initiating genetic event, because acquisition of i(12p) has been shown to be preceded by polyploidization.
A nonrandom pattern of over-representation and under-representation of other chromosomal regions has also been reported, although at a lower frequency than the gain of 12p. These changes are common to both seminomas and NSGCTs, supporting the hypothesis of a common origin for all histologic subtypes of TGCTs.
Differential chromosomal gains and losses have also been reported between seminomas and NSGCTs. Gain of chromosomes 7, 15, 19, and 22 and loss of chromosome 17 have been associated with seminomas, whereas gain of chromosomes 5q, 6q, 13q, and 17q and loss of chromosomes 10q, 19, and 22 were detected in NSGCTs, suggesting these regions may be important in the differentiation of TGCTs. Several recent studies have attempted to identify differentially expressed genes corresponding to such regions of chromosomal gains and losses. Despite this, few of the identified genes have been shown to contribute to the development or progression of TGCTs.
Epigenetic changes
Epigenetic silencing of tumor suppressor genes is known to play a significant role in carcinogenesis. The epigenetic regulation of gene expression involves DNA methylation, post-translational modifications of the histone proteins, or both. Aberrant DNA methylation in the proximal promoter region of tumor suppressor genes has been reported in virtually every tumor type and is frequently associated with silencing of gene expression, although the genes inactivated by methylation varies with tumor type.
Despite their genetic similarities, seminomas and NSGCTs are characterized by distinct epigenetic phenotypes. Using restriction landmark genomic scanning, Smiraglia and colleagues analyzed 16 TGCT samples and observed virtually no CpG island methylation in the seminomas, whereas CpG island methylation in NSGCTs was similar to that previously identified in other solid malignancies (mean 0.08% vs 1.11%, P = .003). Seminomas were also characterized by a higher level of global hypomethylation compared with NSGCTs. This pattern was also demonstrated in several candidate gene studies: methylation of one or more gene promoters was frequently detected in NSGCTs (eg, MGMT, RASSF1A, APC , and FHIT ) whereas minimal methylation was found in seminomas. Consistent with these findings, Almstrup and colleagues demonstrated upregulation of DNA methyltransferase 3B (DNMT3B) in NSGCTs but not in seminomas compared with ITGCNU. DNMT3B is predominantly involved in establishing de novo methylation patterns.
Furthermore, aberrant promoter methylation was associated with absent or downregulated gene expression in most of the methylated genes, and reactivation of gene expression was frequently observed, in vitro, after treatment with the demethylating agent, 5-aza-2’deoxycytidine.
Recently, the distinct methylation profiles have been correlated with differentiation. Using immunohistochemical staining against 5-methylcytosine to assess global methylation patterns, hypomethylation was observed in ITGCNU and seminomas, whereas the more differentiated nonseminomas (eg, yolk sac tumors, choriocarcinomas, and teratomas) were all consistently hypermethylated. This was also observed using a candidate gene approach. Using microdissected cells, the upstream region of OCT3/4 (a transcription factor involved in the maintenance of pluripotency in embryonic stem cells) was found hypomethylated in seminomas and in the undifferentiated nonseminomatous embryonal carcinoma, whereas this region was hypermethylated in the differentiated nonseminomatous components. Distinguishing methylation changes that reflect normal development from those contributing to TGCT pathogenesis presents an important challenge.
Gene expression profiling
Using gene expression profiling, distinct molecular signatures have also been observed between seminomas and the different histologic components of NSGCTs, providing further insight into germ cell tumorigenesis. Port and colleagues analyzed 11 TGCTs (5 seminomas and 6 NSGCTs) and revealed almost opposing gene expression profiles between seminomas and NSGCTs. The majority of the differentially expressed genes fell into 5 functional groups: cell cycle, intracellular transducer, apoptosis, DNA synthesis and repair, and transcription, irrespective of the histologic subtype. Korkola and colleagues performed gene expression profiling on 84 NSGCTs (42 pure and 42 mixed) and identified differentially expressed genes predictive of each subset of NSGCTs. Using hierarchical clustering, Hofer and colleagues identified a new subgroup of seminomas with an expression profile similar to the embryonal carcinoma component of NSGCTs. The 2 subgroups of seminomas distinguished were otherwise histologically similar. Whether this allows identification of a subgroup of patients with a poorer prognosis remains unknown but is worthy of investigation.
Gene expression profiling studies have also been used to predict clinical outcome in patients with TGCTs. Sugimura and colleagues performed gene expression microarray analysis on 17 metastatic NSGCTs and identified 11 differentially expressed genes that could distinguish early-relapse NSGCTs from late-relapse NSGCTs. More recently, Korkola and colleagues performed gene expression profiling on 108 NSGCT patients treated with cisplatin-based chemotherapy and identified a gene expression signature that could predict overall survival.
Biology of chemosensitivity
The exquisite sensitivity of TGCTs to cisplatin-based chemotherapy is thought to be related to their embryonal origin. Embryonic stem cells retain the potential to produce any cell type in the body, and these cells therefore have a high sensitivity to DNA damage to maintain genomic integrity. The high sensitivity of TGCTs to chemotherapy in vivo is also maintained in vitro with many studies demonstrating that TGCT cells are 2-fold to 4-fold more sensitive to cytotoxic agents or irradiation compared with other types of tumor cells. Thus, TGCT provides an ideal model in which to study the mechanisms of chemotherapy sensitivity.
In general, 4 factors determine the sensitivity of a cell to chemotherapeutic agents: transport of the drug across the cell membrane, drug metabolism, accessibility of the drug to DNA, and the cell’s response to DNA damage. The molecular basis for the extreme chemosensitivity of TGCTs remains poorly understood but is thought to be predominantly related to the DNA damage response pathways.
Expression of p53
It was initially proposed that the absence of p53 mutations and the expression of high levels of wild-type p53 in TGCTs might account for the chemosensitivity of these tumors. More than 30% of TGCTs, however, show no p53 staining using immunohistochemistry. Furthermore, in clinical samples, a high level of p53 expression did not relate to treatment sensitivity of the TGCTs; p53 was detected in 59% of the treatment-sensitive tumors compared with 89% of the treatment-resistant TGCTs. Thus, the expression of wild-type p53 alone is insufficient to account for the chemosensitivity of TGCTs.
Apoptosis
Studies investigating the role of apoptosis in TGCTs have reported conflicting data. An initial study reported relatively high levels of expression of the proapoptotic protein Bax in 3 TGCT cell lines and no expression of the antiapoptotic protein Bcl-2, and this high Bax:Bcl-2 ratio was hypothesized to contribute to the 15-fold higher sensitivity to etoposide-induced apoptosis observed in the TGCT cell lines compared with 3 bladder cancer cell lines. Subsequent transfection of one of these TGCT cell lines (833K) with Bcl-2 resulted in a surprising increase in sensitivity to drug-induced apoptosis, and the observed reciprocal down-regulation of the Bcl-2 homolog, Bcl-X L was proposed as the mechanism by which Bcl-2 transfection resulted in activation of apoptosis. A separate study, however, using 4 different TGCT cell lines observed no correlation between the expression of Bax, Bcl-2, and cisplatin-induced apoptosis. Furthermore, although a high Bax:Bcl-2 ratio was also detected in the invasive components of 46 TGCT samples derived from patients with both chemosensitive and chemotherapy-refractory disease, this was not associated with treatment outcome.
Seladin-1 is a multifunctional protein involved in apoptosis. Recently, an inverse relationship between the expression levels of seladin-1 in TGCT cell lines and sensitivity to cisplatin was demonstrated, and the antiapoptotic effect was mediated through inhibition of caspase-3 activation.
DNA Repair Pathways
In mammalian cells, nucleotide excision repair (NER) is the main mechanism by which cisplatin-induced intrastrand DNA cross-links are removed. In 1988, Bedford and colleagues provided the first evidence that NER was defective in TGCTs: using atomic absorption spectroscopy and alkaline elution, TGCT cell lines demonstrated a reduced ability to remove platinum-DNA adducts from the genome compared with a bladder cancer cell line. The reduced capacity for NER in TGCT cell lines has been attributed to low levels of the core NER proteins, xeroderma pigmentosum group A protein, and the ERCC1-XPF endonuclease. Clinical data correlating low NER protein levels to adverse clinical outcome, however, are currently lacking.
Interstrand DNA cross-links (ICLs) account for less than 5% of all cisplatin lesions and these are repaired by ICL repair. Recently, it has been demonstrated that TGCT cell lines are also deficient in ICL repair, due to low level expression of ERCC1-XPF, and that this accounts, at least in part, to the sensitivity to cisplatin.
High-mobility group domain proteins have also been investigated. These proteins specifically recognize and bind to the main cisplatin-induced intrastrand cross-link, 1,2 d(GpG) adduct and block these lesions from NER. In TGCT cell lines, the human testis-determining factor, SRY, and the murine testis-specific high-mobility group domain protein have been shown to bind to the cisplatin-DNA adducts with high affinity. Again, however, clinical data are lacking.
Biology of chemotherapy resistance
Many mechanisms associated with resistance to chemotherapy have been identified, predominantly from studies using drug-resistant cell lines. Cisplatin resistance has been the most widely studied.
Transport of Cisplatin Across Cell Membrane
One potential mechanism for resistance is to reduce the intracellular concentration of cisplatin that is available to target DNA, either by preventing drug influx or by exporting the drug out of the cell. Transporters involved in the maintenance of copper homeostasis have been found to be important in the transport of platinum agents. Decreased expression of CTR1, the major influx transporter, and overexpression of ATP7A and ATP7B, the efflux transporters, have been associated with cisplatin resistance in vitro. In ovarian and gastric carcinomas that were refractory to cisplatin, overexpression of ATP7B has also been reported. The expression of these transporters in TGCT in vivo, however, has yet to be reported.
Overexpression of the ABC transporters has also been investigated but not been shown to contribute significantly to chemotherapy resistance. No significant difference in the levels of 4 ABC transporters (Pgp, MRP1, MRP2, and BRCP) or the major vault protein, LRP, were detected between TGCT samples taken from patients with responsive and those with resistant disease, although individual samples from chemotherapy-resistant cases showed high expression of MRP2.
Drug Metabolism
Elevated levels of the detoxifying agents, glutathione and metallothionein, can also decrease the intracellular levels of active drug. Conflicting data, however, have been published from different studies, suggesting that drug detoxification does not contribute significantly to cisplatin resistance in TGCTs.
Cell’s Response to DNA Damage
Mismatch repair pathway
The mismatch repair (MMR) pathway contributes to acquired cisplatin resistance. Platinum complexes interfere with normal MMR activity and prevent a complete repair of DNA damage. When the MMR pathway is intact, this leads to the initiation of apoptosis. When MMR is deficient, cells can continue to proliferate in spite of DNA damage caused by cisplatin. Studies in several human tumor cell lines have shown that loss of DNA mismatch repair due to lack of expression of hMSH2 or hMLH1 results in low-level resistance to cisplatin. In addition, in a xenograft model, this low-level resistance (<2-fold) was sufficient to impair clinical response to cisplatin in vivo.
There is increasing evidence that deficiency of MMR also contributes to chemotherapy resistance in TGCTs. Mayer and colleagues detected microsatellite instability, a determinant of deficiency of the MMR pathway, in 5 of 11 refractory TGCTs tested compared with 6 of 100 unselected TGCTs examined (45% vs 6%, P = .001). Furthermore, in a series of 162 TGCTs, Velasco and colleagues demonstrated that decreased immunohistochemical staining for MMR proteins and/or increased frequency of microsatellite instability was associated with a shorter time to tumor recurrence and death. Confirmation of these findings in a prospective study would be useful. Recently, a significantly higher incidence of the BRAF V600E mutation was detected in chemotherapy-resistant TGCTs compared with control TGCTs, and this was highly correlated with microsatellite instability. Because multikinase inhibitors targeting BRAF are available (eg, sorafenib), it will be important to evaluate these agents in patients with chemotherapy-resistant TGCTs.
Apoptosis
p53 Mutations were initially thought to contribute to cisplatin resistance. Houldsworth and colleagues detected p53 mutations in 4 of 23 patients with either primary resistant or chemotherapy-relapsed TGCTs. All the mutations, however, were identified in TGCTs with a teratomatous histology, which are known to be intrinsically resistant to chemotherapy. A subsequent study identified p53 mutations in only 1 of 17 treatment-sensitive TGCT and in 0 of 18 treatment-resistant TGCTs, and expression of p53 was not lower in the refractory tumors compared with the responsive group. This second study contained a lower number of mature teratoma samples. Thus, p53 mutations are unlikely to account for cisplatin resistance in most patients with TGCTs.
Preclinical data have also shown that p53 mutations do not contribute significantly to cisplatin resistance: the susceptibility of 6 TGCT cell lines to induction of apoptosis was not associated with their p53 status. Furthermore, inactivation of wild-type p53 function by transfecting cells with the HPV16 E6 gene did not affect cisplatin-induced or gamma irradiation–induced apoptosis. Thus, wild-type p53 expression is not required for the efficient induction of apoptosis in TGCT cell lines. Cisplatin has been shown to mediate p53-independent apoptosis via activation of the Fas/CD95 or the MEK-ERK signaling pathways, and inactivation of these pathways has been associated with resistance to cisplatin-induced apoptosis in vitro.
The caspase family of proteases also has been investigated. Inhibition of caspase-9 in TGCT cell lines almost completely blocked apoptosis and induced cisplatin resistance. This effect was independent of the expression of p53, Fas receptor, Fas ligand, or the Bcl-2 family proteins.
NER
Although reduced NER has been shown to contribute significantly to the chemosensitivity of TGCTs, enhanced NER has not emerged as a major contributor to cisplatin resistance. No difference in the DNA repair capacity was detected in 2 sublines with acquired cisplatin resistance, compared with the cisplatin-sensitive parental cell lines.
Genome-wide Studies
Genome-wide studies have also been conducted to identify the genetic mechanisms underlying cisplatin resistance. Using comparative genomic hybridization, high-level amplification in 8 regions were observed in 5 of 17 chemotherapy-resistant TGCTs: 1q31–32, 2p23–24, 7q21, 7q31, 9q22, 9q32–34, 15q23–24, and 20q11.2–12. Further studies are needed, however, to identify potential candidate genes residing in these regions. Wilson and colleagues performed comparative expressed sequence hybridization, a technique for gene expression profiling along chromosomes, on 3 pairs of TGCT cell lines, and revealed a striking overexpression of 16q in all 3 cisplatin-resistant cell lines compared with their cisplatin-sensitive parental cell lines. This suggests that a gene or group of genes from 16q plays an important role in cisplatin resistance in TGCTs, although, again, the relevant genes remain to be identified. More recently, gene expression profiling has been performed on these 3 pairs of TGCT cell lines and revealed only a few differentially expressed genes when comparing the parental cells with the resistant cells. The most significantly differentially expressed gene was found to be CCND1 , and in a series of 25 clinical samples (13 resistant and 12 sensitive), significantly higher levels of CCND1 expression were detected in the resistant cases compared with the sensitive samples. Furthermore, CCND1 overexpression was detected in most of the resistant samples, suggesting that CCND1 overexpression may be a common mechanism of cisplatin resistance in TGCTs.
Epigenetics and Cisplatin Resistance
Two recent studies have demonstrated in vitro that chemical demethylation using 5-azacytidine or 5-aza-2’-deoxycytidine can resensitize cisplatin-resistant cells to cisplatin-mediated toxicity. Further in vitro and in vivo studies are needed to assess if these agents may provide an additional therapeutic modality for patients with resistant TGCTs.
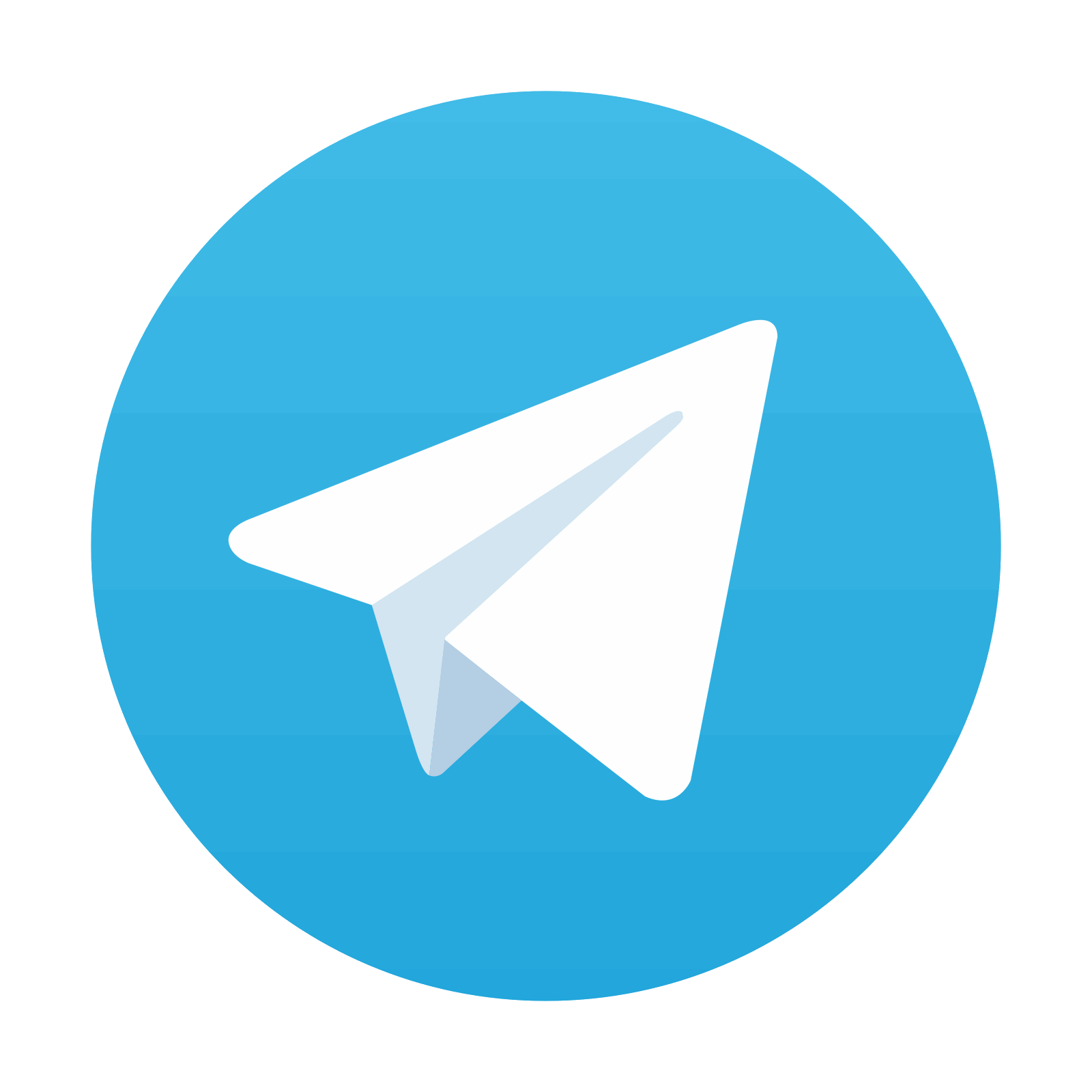
Stay updated, free articles. Join our Telegram channel

Full access? Get Clinical Tree
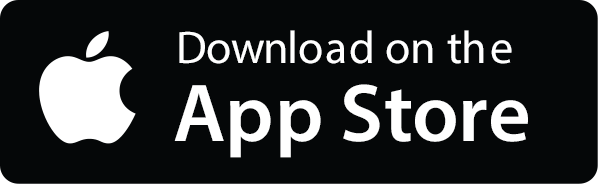
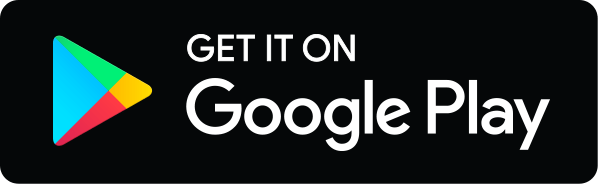