Fig. 1.1
Histopathology of bone metastasis (BMT) from prostate cancer (PC). In the same patient, BMTs are heterogeneous, with predominantly blastic (1) and predominantly lytic metastases (2). Furthermore, as shown in the histopathological sections in the same specimen of a single metastasis, there is an alternation of predominantly lytic and blastic area (mixed pattern) (3): 2–20 % Bone volume: predominantly lytic area; 50–70 % bone volume: predominantly blastic area (Modified from Roudier et al. [12]). Green bone, red osteoid, grey/pink tumour stroma
Bone metastases in castration-resistant PC patients were characterised according to expression levels of steroidogenic enzyme and androgen receptor splice variants. It was found that increased tumour expression of steroidogenic enzymes in individual patients is associated with advanced tumour stage. Interestingly, there are distinct subgroups of CRPC patients with BMTs expressing high levels of AKR1C3 (that convert circulating dehydroepiandrosterone and androstenedione (synthesised in the adrenals) into 5-androstenediol and testosterone) or expressing high levels of ligand binding domain (LBD)-truncated, constitutively active androgen receptor splice variants (AR-Vs). The possible clinical relevance of this is that patients with high AKR1C3 expression and low AR-V expression may show a good response to treatment with abiraterone acetate (Cyp17 inhibition) and/or would benefit from drugs targeting AKR1C3, whereas patients with a high expression of constitutively active AR-Vs will probably not respond to abiraterone acetate or to any therapy targeting androgen synthesis or the LBD of the AR [14].
1.3 Pathophysiology of Bone Metastasis
1.3.1 Pathophysiological Heterogeneity
The osteoblastic lesion is a very complex multistep process that is not fully understood in detail. It is the result of releasing osteoblast-promoting factors such as bone morphogenetic protein (BMP), Wnt family ligand, endothelin-1 and PDGF from PC cells and of a closed interaction with bone matrix, stroma cells and bone cells. Another characteristic of BMT from PC is the biological and pathophysiological heterogeneity. The high level of heterogeneity of the BMTs in PC from the pathological point of view reflects the great complexity of the biology and molecular regulation that underlie their pathophysiology. Lytic and blastic metastases share many molecular mechanisms that give an account of similar therapeutic outcome treating them with bone-modifying agents such as zoledronic acid and denosumab. The complexity of the bone response in PC invasion is underscored by the variety of soluble factors, signalling pathways and transcriptional regulators involved. The abnormal bone response is further promoted by the potential for osteomimicry of the tumour cells signalling in a paracrine fashion within the bone environment and an autocrine signalling cascade of the bone cells themselves. These interactions between the PC cells and bone cells often yield a predominantly osteoblastic response. However, the formation of osteoblastic bone is also often associated with a significant osteolytic component, leading to a mixed, woven bone response in the same patient at different metastatic sites.
Bone remodelling proteins and transcripts in human specimens of PC BMTs were analysed in detail [15]. The main bone remodelling proteins that were recognised were assessed in lytic and blastic BMTs: BMP-2, BMP-7, dickkopf-related protein 1 (DKK-1), receptor tyrosine-protein kinase erbB-3 (ErbB3), endothelin-1 (ET-1), NEL-like protein 1 (NELL-1), tumour necrosis factor receptor superfamily 11B (OPG), phosphoglycerate kinase 1 (PGK1), sclerostin, substance P, a putative osteoblastic factor EMI domain-containing protein 1 (Emu1) and two putative osteolytic factors, matrix metalloproteinase-12 (MMP-12) and secreted frizzled-related protein 1 (SFRP1). Interestingly, many of these proteins and transcripts were equally expressed in lytic and blastic BMTs, such as BMP-2, BMP-7, DKK-1 and sclerostin. Instead, expression of some of these, such as OPG, Emu1, PGK1 and substance P, was higher in prevalent blastic lesions than in lytic lesions, but not the transcripts. OPG, PGK1 and substance P have been proven to inhibit osteoclastogenesis and induce osteoblastic differentiation. Emu1 has been shown to be prevalent in the epithelium during embryonic development and it has been hypothesised that Emu1 in PC aids adhesion The single proteins are probably not the unique drivers for conditioning the evolution towards a blastic phenotype of the metastasis, and a possible explanation for the characteristic “predominantly osteoblastic phenotype” is that PC expresses a disproportionate number of pro-osteoblastic and pro-osteolytic factors and the relative prevalence of the former will determine the pathological aspect of the lesion [15, 16].
1.3.2 The Role of Osteoclasts in Blastic and Mixed Bone Metastases
Independently from the phenotype of the lesion, osteoclasts, mainly in the first phases of BMT development, are principally responsible for the initiation, development and clinical consequences such as pain, fracture and hypercalcemia of the evident bone lesion (Fig. 1.2).


Fig. 1.2
Physiopathology of blastic bone metastasis (BMT). Osteoclasts (yellow cells) reabsorbing bone facilitate the expansion of PC metastasis and make available in the bone microenvironment factors promoting penetration and growth of metastasis (TGF beta, osteopontin, FGF, PDGF, VEGF, IGF-1 and IGF-1 are described in detail in the text). In turn, PC cells express cytokines (RANKL, DKK-1 and hormone such as PTHrP) that maintain osteoclast activity and cytokine and factors such as uPA and ET-1, inducing osteoblast bone formation. In the micro-environment of a BMT site, the high bone turnover is characterised by the alternation of osteoclast (lytic) areas and osteoblastic (woven bone) areas, resulting in a disorganised and frail bone structure
Osteoclasts have two pivotal functions in the development of bone lesions: they reabsorb the bone, creating the necessary space for the penetration and development of metastasis into the bone, and they enrich, as a direct consequence of the bone matrix breakdown, the bone micro-environment of a plethora of growth factors and tumour-seeking factors that sustain the proliferation of the cancer cells, which is essential during the first phases of metastasis. These mechanisms are the basis of the “seed and soil” concept, where the bone micro-environment factors represent the fertile ground (the soil) and the “seed” represents cancer cell growth.
Physiologically, bone resorption and bone formation in skeletal remodelling are almost always tightly coupled. The bone resorption by osteoclasts is regulated by the RANK/RANKL/OPG axis, where osteoblasts expressing RANKL induce recruitment, differentiation and activation of osteoclasts, binding and activating of RANK, and conversely expressing OPG, the RANKL decoy receptor, and osteoblasts inhibit the excess osteoclastogenesis. The ratio RANKL/OPG in bone micro-environment drives the equilibrium between bone formation and resorption.
Expression of RANKL by stromal cells/osteoblasts and osteocytes is regulated by cytokines and paracrine hormones that stimulate bone resorption [17] such as interleukin-1 (IL-1), IL-6, IL-11, IL-17, prostaglandin E2 (PGE2), parathyroid hormone (PTH) and parathyroid hormone-related peptide (PTHrP), which stimulate osteoblasts or their progenitors to express RANKL and/or to downregulate the expression of OPG [18]. Recently, the role of osteocytes through the Wnt/DKK-1 and sclerostin pathway has been elucidated (Fig. 1.2) [19].
The tumour cells co-opting the normal process that regulates bone resorption interfere with the balance of the RANKL/RANK/OPG axis. The tumour/bone interface is replete with factors that stimulate bone resorption directly produced by tumour cells themselves, by macrophages and T cells associated with metastasis or by stromal cells influenced by metastasising cells. PTHrP, IL-8 and PGE2 have been shown to increase expression of RANKL and downregulate OPG expression either in vitro in the osteoblast/tumour cell coculture or in vivo using the BMT model [17–20].
Parathyroid hormone-related peptide is not physiologically present in the circulation, but it has been found to be widely distributed in most fetal and adult tissues [21], suggesting that it might act in an autocrine/paracrine manner. This peptide plays an important role in regulating many tissues including cancer tissue [22]. PTHrP is expressed by many types of cancer cells, such as breast cancer and PC, and has been proposed as an antigen for cancer immunotherapy [23–26]. PTHrP, as PTH in physiology, stimulates osteoblasts expressing PTHR1 receptor to express RANKL, which activates osteoclasts [27]. Interestingly, it has been found that T cells also express PTHR1 and are activated by PTH and PTHrP [28, 29], contributing to osteoclast activation via RANKL. It has been demonstrated that in mice bone resorption may be prevented by the immunosuppressor abatacept, a CTLA4-Ig preventing T-cell activation [30].
In addition to PTHrP, IL-8 plays an important role in the activation of osteoclasts. IL-8 is the human homologue to murine MIP-2 belonging to the family of chemokine CXC and is constitutively produced by osteoblasts [31]. IL-8 is overexpressed in the breast cancer cell line [32], and it is believed that it acts before PTHrP in the early stages of breast cancer metastasis stimulating osteoclasts via RANKL [32, 33] and then initiating the vicious cycle that maintains osteolysis in cancer metastasis. It has been suggested that IL-8 might also directly stimulate osteoclasts [33], increase angiogenesis and suppress osteoblast activity [34, 35].
Cancer cells in BMT produce many factors that activate T cells, as discussed above. T cells of patients with breast, prostate and lung cancer support osteoclastogenesis by secreting TNF alpha and expressing RANKL. In addition, T cells suppress the osteoprotegerin action secreting TRAIL (TNF-related apoptosis-inducing ligand), therefore inhibiting the anti-osteoclastogenic effect of osteoprotegerin [36]. In turn, cancer cells produce many factors such as PTHrP, IL-7 and IL-,8 which could recruit or activate T cells with the consequence of further stimulating osteoclastic bone resorption. These mechanisms contribute to the imbalance towards the osteolytic phenotype of the bone lesion.
Studies using RANKL inhibitors have shown the almost complete dependence of tumour-mediated osteoclastogenesis on RANKL. Treatment of mice with OPG-Fc prevented the progression of osteolysis induced by the breast cancer cell line MDA-MB-231 [37]. RANKL inhibition has been shown to prevent the implantation and development of osteolytic lesions in the PC3 cell line in animals [38, 39]. The efficacy of RANKL inhibition was also demonstrated in mixed BMTs in animals, where OPG-Fc blocked the establishment and progression of bone lesions [40, 41]. Recent data indicate that cathepsin G activity at the tumour–bone interface plays an important role in tumour-induced osteolysis and suggest that cathepsin G might be a potentially novel therapeutic target in the treatment of BMT. In a mouse model that mimics osteolytic changes associated with breast cancer-induced BMTs, it has recently been demonstrated that cathepsin G, cooperating with MMP9 and MMP13, is able to cut the extracellular domain of RANKL, generating active soluble RANKL, which is critical for widespread differentiation and activation of osteoclast precursors [42].
Furthermore, some RANKL-independent ways for osteoclast activation in BMT have been found. Some cancer cells, such as PC and breast cancer, may express RANKL and directly activate osteoclasts [43, 44]. Breast cancer cells, myeloma cells and other cancer cells could directly activate osteoclasts in the early stages of BMT via IL-8 production and via MIP-1, a member of the CXC chemokine family that is naturally secreted by osteoblasts and is primarily associated with cell adhesion and migration. It is chemotactic for monocytes and monocyte-like cells, including osteoclast precursors. It directly stimulates osteoclast formation and differentiation in a dose-dependent manner, through the receptors CCR1 and CCR5 expressed by osteoclasts. Moreover, neutralising antibody against MIP-1 blocks MIP-1-induced osteoclast activation [45, 46].
1.3.3 The Role of Osteoblasts in Blastic and Mixed Bone Metastases
In blastic metastases the number and activity of osteoblasts are amplified. Osteoblast differentiates from bone marrow mesenchymal stem cells. A variety of factors contribute to osteoblast formation, including insulin-like growth factor, endothelin-1, BMPs and sclerostin and Wnt proteins (Fig. 1.2) [47, 48].
1.3.4 Endothelin-1
Production of endothelin-1 (ET-1) from PC cells has proven to induce a blastic metastasis promoting osteoblast differentiation and activity. ET-1 is a small vasoconstrictive peptide that plays a key role in vascular homeostasis. ET-1 promotes osteoblast function by binding to ET receptor subtype A (ETA). The activation of receptor ETA stimulates phosphate transport and is important for the initiation of bone matrix calcification. ET-1 also increases osteoblast proliferation and inhibits osteoclast formation and motility, and recently it has been suggested that these actions might be indirect and mediated through the Wnt/DKK-1 pathway, inhibiting DKK-1 [49–51]. ET-1 can also enhance the mitogenic effect of other growth factors, such as insulin-like growth factor 1 (IGF-1), platelet-derived growth factor (PDGF) and epidermal growth factor (EGF) [52]. Furthermore, ET-1 has been found to be elevated in androgen-resistant advanced PC. However, there are some doubts with regard to the pivotal role of ET-1 in osteoblastic lesions from PC, because a clinical trial with atrasentan, a selective ET receptor antagonist, produced a modest effect on metastatic PC [53].
1.3.5 Bone Morphogenetic Proteins
The expression of several BMPs has been detected in BMTs from PC. BMPs seem to have a crucial role in contributing to osteoblastic phenotype of BMT in PC. BMPs are members of TGF-beta family and are known to be involved in cancer cell migration. In PC tissues, the expression of BMP-7 was higher in metastatic bone than in normal tissue.
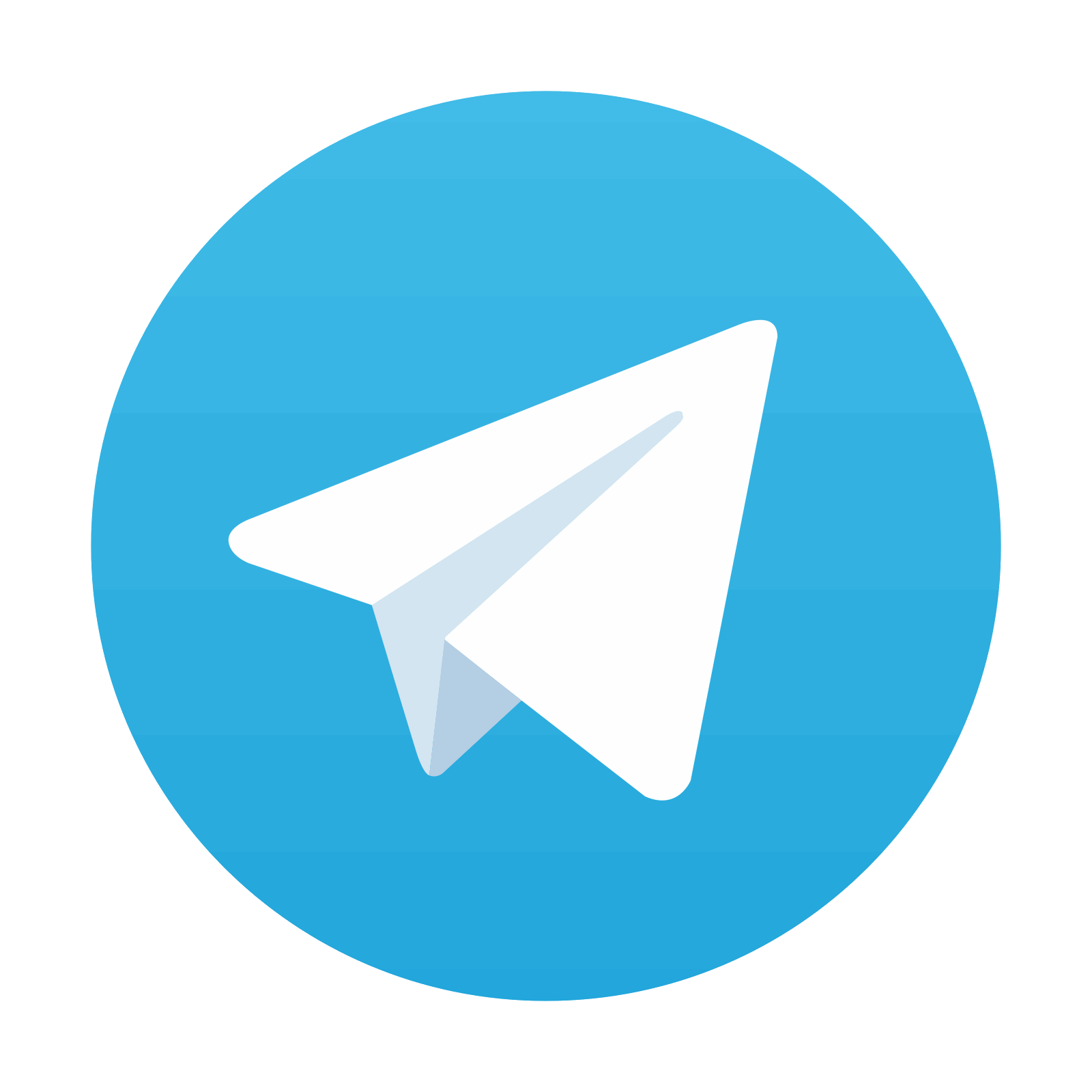
Stay updated, free articles. Join our Telegram channel

Full access? Get Clinical Tree
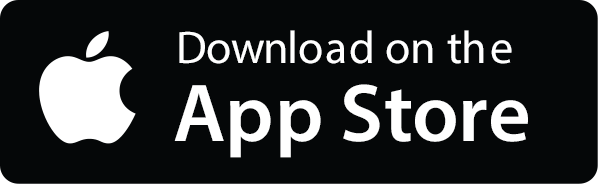
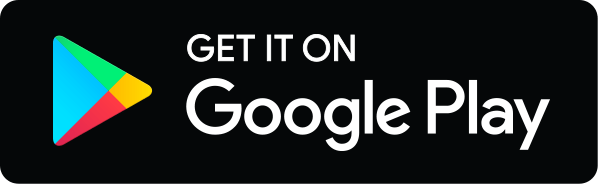