© Springer International Publishing Switzerland 2016
Gilles Freyer (ed.)Ovarian Cancer in Elderly Patients10.1007/978-3-319-23588-2_99. Biological Research: Current Directions
(1)
Geriatrics Unit, CarMEN Laboratory, Lyon Sud University Hospital, Lyon University, Pierre-Bénite, France
(2)
HCL Cancer Institute, Medical Oncology, and Université de Lyon, Lyon, France
(3)
Institute for Research on Cancer and Aging Nice (IRCAN), Université de Nice-Sophia Antipolis, UMR 7284 CNRS, U1081 INSERM, Nice, France
(4)
Department of Medical Genetics, Archet 2 Hospital, Centre Hospalier Universitaire de Nice, Nice, France
Introduction
Ovarian cancer treatment has progressed considerably over the past 10 years. Substantial changes in treatment guidelines are attributable to the advent of targeted therapies. This revolution was accompanied by a serious effort to understand the biological drivers of tumour development and progression. Of these, senescence and ageing pathways are good candidates for exploration using age-related tumor models. In this respect, ovarian physiopathology affords a model system that may allow us to better understand senescence pathways and the impact thereof both loss of fecundity and cancer development. As ovarian cancer demographics peak at older ages, it is tempting to speculate that a relationship exists between senescence and cancer.
Cancer treatment in the elderly is always associated with the question of how to assess geriatric populations and how to use such assessments to plan treatment. Again, modern biology may provide objective tools facilitating such work: biomarkers of aging must be defined.
This review will seek to answer two principal questions:
How do ovarian ageing and ovarian carcinogenesis illuminate the theories of ageing?
Are biomarkers of aging clinically relevant in the context of ovarian cancer?
Ovarian Biology and the Theories of Ageing
Various theories on aging have been formulated, commencing in the early 1950s. Modern biology has provided some support for all theories; illustrative examples and proofs-of- concept have been described. In the field of ovarian biology, in particular, it is tempting to speculate that a relationship exists between senescence and cancer development. Ovarian cancers increase in number after menopause and peak at 70–80 years of age. From a biological viewpoint, menopause is a consequence of ovarian germ cell senescence, and a complex relationship is known to exist between senescence and cancer; senescence is both a barrier to cancer and tumor-promoting in nature. This section will describe the various theories of aging, again developed from the 1950s, and provide current illustrations thereof in the context of tumor biology and, in particular, ovarian cancer.
From Historical Theories of Aging to Modern Biology
Ovarian Ageing: Different Cell Lines – Different Points of View
Ovarian ageing has been extensively studied because of the triple impact on fertility, menopause, and associated cardiovascular risks, and ovarian cancer. Briefly, the ovary comprises various cellular components, including germ, granulosa, stromal, and ovarian surface epithelial (OSE) cells [1]. Premature ovarian failure (POF) is considered as a model of premature ageing and is caused by premature senescence of germ cells, induced by senescence signaling in a particular micro-environment (stromal cells). It is believed that exposure of oocytes to an aged ovarian microenvironment triggers a female age-dependent process termed “reproductive aging” or “maternal aging” [2]. OSE cells regulate the secretion and transport of 17β-hydroxysteroid dehydrogenase and were recently shown to be the major source of ovarian cancer; other cancers originate from fallopian tube epithelium [3] and granulosa cells [4].
The Mutation Accumulation Theory Supports Premature Ovarian Failure and Ovarian Cancer Pathogenesis
The mutation accumulation theory of Medawar, a Nobel laureate, maintains that aging is caused by accumulation of mutations, and that natural selection cannot eliminate mutations that exert no detrimental effects until late in life. This view was prominent in the 1950–60s, creating affiliated theories such as the free radical theory of Harmann and the oxidative stress theory. According to the latter theory, aging promotes cancer development, and mutations affecting aging may thus exert such effects.
Infertility and premature menopause induced by cytotoxic treatments in premenopausal women [5], by cigarette smoke exposure [6] and, (more generally) by oxidative stress [7], constitute models of accelerated ovarian ageing, and amply illustrate the mutation accumulation theory.
Oxidative stress triggered by ovulation induces repeated cycles of DNA damage in OSE cells [8] as do chemical agents such as methoxychlor [9]. Such stress is hypothesized to induce pre-malignancy. Thus, it is not surprising that inhibition of the DNA repair genes BRCA1 and BRCA2 (termed “BRCAness”) via mutation, gene deletion, or gene silencing, affects cancer development. This is an illustration of the mutation accumulation theory in play. Mutations in tumor suppressor genes are not (or are poorly [10]) counter-selected because such mutations exert no detrimental effects on fertility.
The Antagonist Pleiotropy Theory
A theory developed by George C. William suggests that certain genes may be positively selected because they promote reproduction and genomic stability, but that such gene expression may be detrimental later in life. This theory is usually exemplified by the dual role played by p16 INK4A or p53; the gene is responsible for genomic stability by triggering cell-cycle arrest during DNA repair and, eventually, apoptosis and senescence. However, such activities may cause tissue exhaustion and clonality restrictions later in life, promoting aging in tissues with high-level renewal turnover.
In the ovary, early senescence of stromal cells triggers germ cell senescence; this is a perfect illustration of antagonist pleiotropy. Early cessation of germline meiosis is indeed supposed to ensure the quality of the transmitted genome. Maintenance of high-level proofreading mechanisms in germ cell lines creates high risks of infertility and premature ovarian failure after exposure to high-level oxidative stress (please see below).
The Disposable Soma Theory
August Weissman was the first to conceptualize what was later described as the disposable soma theory by Thomas Kirkwood; this features the co-existence of different proofreading devices in somatic and germline cells. In the former cells, low-accuracy mechanisms allow energy saving, leading to accumulation of mutations and, eventually, cell deterioration or death, whereas high-quality proofreading is necessary in germ lines, to ensure maintenance of the species. Ovarian physiology fits well with such a “wear and tear” theory of aging because, in ovarian germline cells, early detection of a mutation triggers premature meiotic arrest and apoptosis. In line with this view, most ovarian cancers are derived from somatic cells and, in particular, OSE cells. Indeed, during fertile life, somatic OSE cells are subjected to constant high-level DNA damage [8].
One of the more prominent illustrations of the disposable theory is the discovery of the telomerase gene by E. Blackburn. Telomeres are complex nucleoprotein structures located at chromosomal extremities and dedicated to the protection thereof from the mutagenic risk associated with linear DNA (that may be recognized by the nuclear machinery as containing DNA breaks). Each round of chromosomal replication is associated with systematic telomere loss at the 5′ extremity, leading to progressive telomeric attrition, which is compensated, to different extents depending on the cell type, by telomerase-dependant elongation of the truncated telomeres. In germline cells, telomerase is expressed at high levels, allowing long telomeres to be maintained in gametic cells. In somatic cells, however, low-level or no telomerase expression is associated with a high rate of telomere loss, triggering DNA damage that ultimately causes senescence or apoptosis. If this response fails, a second level of genomic protection is available for even shorter telomeres triggering cell crisis and death.
Mice programmed to synthesize abnormally short telomeres are compromised in terms of both fertility and embryonic development [11]. Moreover, the telomere reverse transcriptase is more highly expressed in oocytes from young females, and telomere length in oocytes declines with age [12, 13]. As the oocytes of adult females are believed to be principally post-mitotic, any significance of the observed reductions in telomerase expression and telomere length remains unclear. It is possible that increased oxidative stress during aging contributes to telomere shortening and dysfunction. Another outcome of reduced telomerase expression may be extratelomeric in nature resulting in genome-wide transcriptional changes [14]. Therefore, it is important to better understand how the functions of oocyte telomeres are controlled. In this context, it is interesting that the death-associated protein DAXX, overexpressed in ovarian cancer cells, contributes to maintenance of genomic stability, prevention of cellular senescence, and ovarian oncogenesis, via an interaction with the Promyelocytic Leukemia Protein (PML) [15, 16]. As embryonic stem (ES) cells require DAXX and PML to ensure telomeric integrity [17], it may be speculated that these two proteins are also involved in telomeric maintenance in OSE cells, to protect such cells from senescence.
The Complex Relationship Between Senescence and Cancer
Senescence as an Anticancer Process
A protective role of senescence in the context of cancer is suggested by the fact that oncogene expression is sufficient to trigger senescence in primary human cells. The possible oncosuppressive effect of senescence is supported by a series of papers showing that pre-neoplastic lesions contain high levels of senescent cells [18–20]. Moreover, senescence may be a cytolytic process whereby cancer cells are eliminated by the immune system [21, 22]. From the perspective of ovarian cancer, recent data have confirmed that senescence protects against cancer because, as in most tumors, the senescence pathway is disabled in ovarian cancer cells, which are therefore immortal [23]. Recent data suggest that a synthetic progesterone receptor (PR) agonist exerts an oncostatic effect that depends on induction of senescence in such cells; the protein upregulates expression of p21 and the Forkhead-box transcription factor FOXO1 [23]. These data may explain how high levels of circulating progesterone and progestin-containing oral contraceptives protect against cancer. Moreover, PR and FOXO1 are frequently lost by ovarian cancer cells, further suggesting that these proteins play oncosuppressive roles.
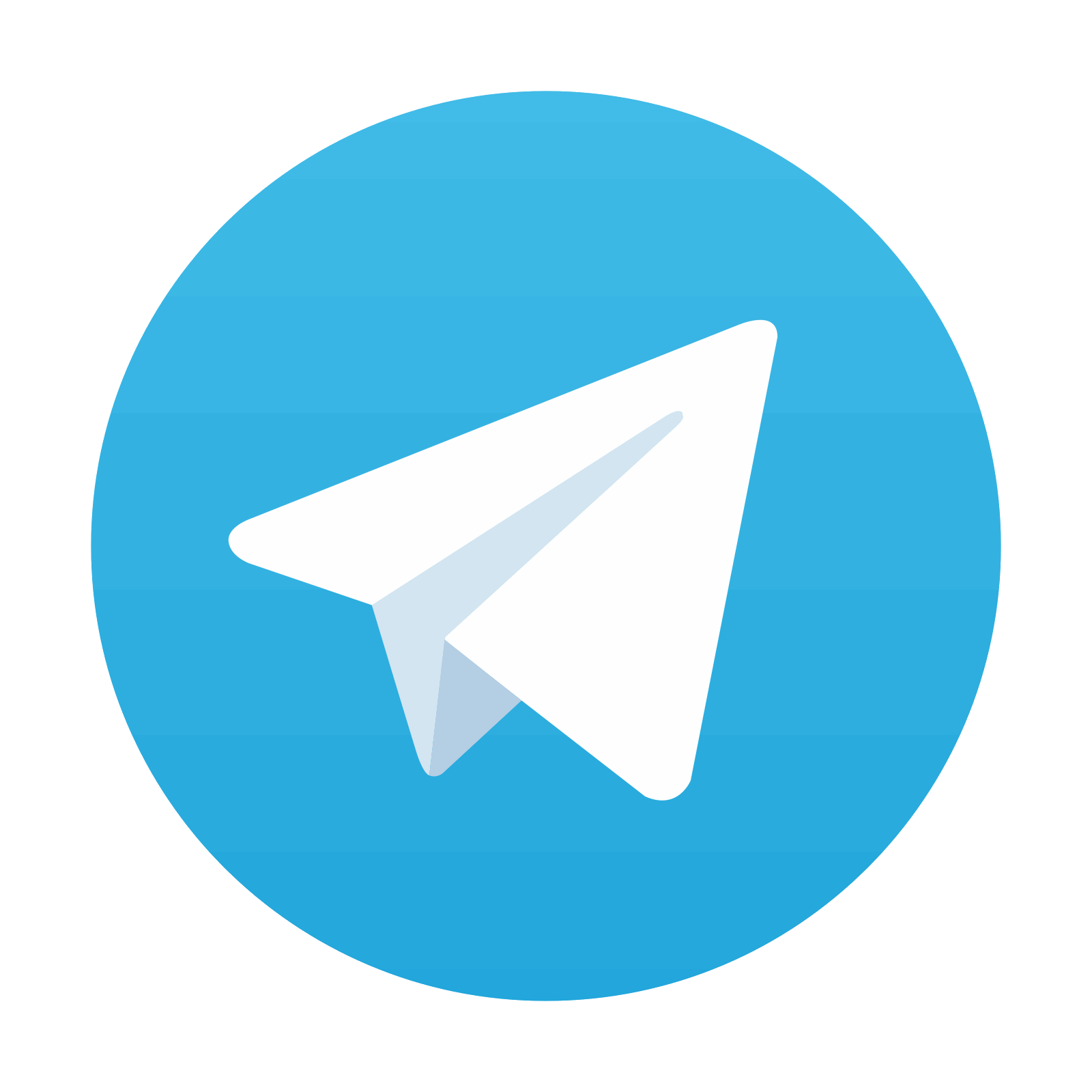
Stay updated, free articles. Join our Telegram channel

Full access? Get Clinical Tree
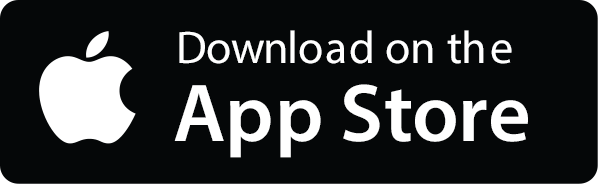
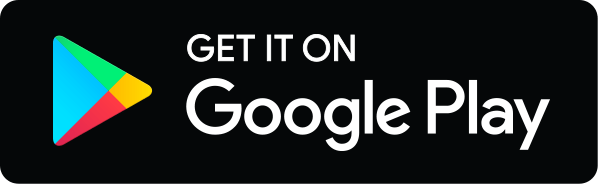