Keywords
Cancer gene therapy, molecular imaging, systemic gene delivery, tumor targeting, vascular targeting, bacteriophage, adeno-associated virus
Introduction
Gene therapy has attracted considerable attention in the past few decades. One of its most promising applications is in the field of cancer treatment. However, several obstacles need to be overcome before this potentially powerful technique can be clinically implemented. It is essential to identify a systemically administered vector that can safely and effectively deliver therapeutic genes to precisely targeted cells anywhere in the body . Experimental investigation of gene therapy for cancer thus involves three key issues:
- 1.
The choice of the target
- 2.
The choice of vector
- 3.
An effective way to measure how well transgenes are functioning
A number of recent investigations have explored tissue-targeted gene delivery. In addition to discriminating target cells with a high degree of precision, this minimizes adverse side effects such as cytotoxicity and immune response by reducing the required vector load. Endothelial cells associated with tumor vasculature are particular suitable for targeting. They are easily accessible to intravenously administered agents, which is particularly important in the case of vectors because they are poor at leaving the circulation. In addition, endothelial cells possess a number of surface markers that are absent or barely detectable in normal blood vessels, including growth factor receptors, cell adhesion molecules, proteoglycans, and proteases .
Regarding the question of the optimal vector, animal viruses have been shown to have significant potential for ligand-targeted gene delivery. However, to avoid toxic side effects, it is necessary to eliminate their native tropism and retarget them to different receptors, which substantially reduced the efficacy . One solution to this drawback is the use of bacteriophage vectors. Unlike animal viruses, bacteriophages do not exhibit tropism, do not bind to receptors on mammalian cells, and are nontoxic to humans. They specifically and exclusively infect bacteria. This makes them ideal candidates for delivering therapeutic genes .
One promising development in this regard is the use of virus–bacteriophage hybrid. In 2006, Hajitou et al. generated a chimera between adeno-associated virus (AAV) and M13 filamentous bacteriophage. This hybrid, which was designated an AAV/phage (AAVP), incorporated a mammalian gene cassette flanked by inverted terminal repeats (ITRs) from AAV2 into an intergenic region of the bacteriophage genome. Thus, the well-characterized gene delivery capability of AAV was packaged within a bacteriophage . The use of this as a vector for ligand-directed internalization, transfer, and expression of transgenes was demonstrated both in vitro and in vivo , with a much higher mammalian transduction efficiency than conventional phage-based vectors .
The optimization of the target and vector used in gene therapy requires an effective way to measure the success of the gene transfer involved. In cancer gene therapy, the level of transgene expression is normally assessed using conventional molecular and histopathological methods. However, these require the sacrifice of experimental animals to obtain the necessary tissue samples and provide information only about the size and accessibility of the tumor. They do not permit an assessment of the temporal and spatial distribution of the applied vectors . Alternative noninvasive techniques capable of providing repeated quantitative in vivo data are obviously desirable. Recent developments in imaging technology have made such monitoring possible, and a number of studies on animal models have been undertaken. These have successfully used positron emission tomography (PET), single-photon emission computed tomography (SPECT), and optical imaging (e.g., fluorescence and bioluminescence imaging) to investigate tumor growth kinetics, to evaluate the effect on cancer progression of genetic modifications, and to assess tumor response to therapies on the molecular level .
This chapter reviews all three issues mentioned previously in more detail in the context of vascular-targeted systemic gene therapy and molecular imaging by RGD4C AAVP.
Targeting with Animal Viruses
The efficacy of therapeutic agents is determined by the difference between targeted and nontargeted cytotoxic effect. This difference is often disappointingly low for current delivery systems, and adverse immune reactions are also problematic . The use of specific tissue-targeting vectors can offer a solution to these concerns. Such vectors can be broadly classified as either transcriptional or ligand targeting. The former involves the use of tissue-specific promoters (TSPs), which cause selective expression of transgenes in target cells . A major disadvantage of this targeting technique is that pathological change in target tissue, such as degeneration or malignant transformation, can ectopically activate TSPs . It also fails to address the problem of toxic side effects due to vector particles localizing in the wrong place .
An alternative targeting strategy involves the incorporation of ligands that mediate selective binding to target cells into the outer capsid proteins of an animal virus . Two kinds of ligand have been used: bispecific conjugates that include anti-vector antibodies and monospecific peptides . The latter have the advantages of ease of handling, better stability, small size, and avoidance of additional immunogenicity. A key property required of such ligands is the ability to induce receptor-mediated endocytosis and subsequent internalization within target cells. In 1996, Pasqualini and Ruoslahti identified a number of suitable ligands by bacteriophage display technology, and this technique is now extensively employed .
However, the integration of ligands into viral vectors does not always result in the selective gene delivery that is sought. The effect of the ligand on the function of the backbone capsid or envelope protein is sometimes unpredictable . Many cell-binding peptides identified by phage display lose the desired activity when displayed in the different protein structures of a viral capsid protein. Only a minority of selected peptide ligands function in targeted phage particles equally well as they do in modified vector capsid such as adenovirus or adeno-associated virus . It has been suggested that the different structural context of capsid protein affects the binding and/or internalization properties of ligands or disrupts viral assembly and function . In order to circumvent this ligand compatibility problem, several groups have developed random peptide-display libraries based on the vector capsid for AAVs and for retroviruses . This approach allows the selection of peptide ligands binding specifically to a certain cell type within the context of the specific viral capsid protein . In another approach to overcome this context problem, Gosh and Barry generated a “context-specific” filamentous phage library in which random peptides were displayed between the H and I sheets in the normal position of the HI loop from the Ad5 fiber protein . They demonstrated the selection and functional translation of a skeletal muscle cell-binding peptide back into Ad5 vectors to increase transduction of these cells.
Targeting the Tumor Vasculature
Specialization of Tumor Vasculature
It is widely accepted that the distinctive nature of tumor vasculature makes it an attractive target for cancer therapies. Generally, solid tumors cannot grow beyond a few millimeters in a diameter without the support of blood vessels to provide oxygen and other essential nutrients as well as to facilitate the elimination of waste products of metabolism . For malignant growth, tumors must stimulate the formation of new capillary blood vessels (angiogenesis). Tumor angiogenesis is highly specialized and biochemically and structurally distinct from homeostatic angiogenesis. It includes irregular vascular structure, increased permeability, enlargement of the vessel diameter, altered endothelial cell–pericyte interactions, an increased number of endothelial cells, a decreased number of pericytes, delayed maturation, and abnormal blood flow . This tumor-associated phenomenon not only promotes the growth and survival of the tumor but also plays a crucial role in its spread (metastasis). The endothelial cells, which line the interior surface of blood vessels and form an interface and barrier between the circulating blood in the lumen and the malignant cells within a tumor, are genetically more stable than cancer cells. This genomic stability makes them very suitable for anti-angiogenic therapy because they are less likely to accumulate mutations that render them resistant to therapy . The processes of angiogenesis induced by the specific tumor environment give rise to unique epitopes that are expressed in the associated endothelial cells, allowing the tumor vasculature to be distinguished from the normal vasculature . Such tissue-specific endothelial cell surface markers, often designated as vascular addresses or “zip codes,” could serve as receptors for tissue-specific binding of soluble circulating ligands or for homing of cells such as leukocytes . In addition, these epitopes are readily accessible to the lumen of blood vessels, which mediate efficient accumulation of targeting agents, whereas most cancer-associated epitopes are expressed on malignant cells inside the tumor compartment, which is not readily available to intravenous agents. For all these reasons, the endothelial cells are considered to be an ideal target for therapeutic intervention.
Phage Display and Vascular-Targeted Peptides
High-throughput technologies for the identification of novel tissue-specific vascular markers have been developed. One of these is the screening of phage display peptide libraries. This has resulted in the wide use of phage as a platform for peptide, protein, or antibody display, enabling the rapid selection of a bacteriophage expressing the appropriate molecule with a high affinity for any substrate, including organic, inorganic, and biological materials. In most cases, the technique involves in vitro selection by immobilizing the target molecule onto plastic or selection on cell surfaces such as biopanning on cultured cells . One possibility is to isolate relevant cells from the target tissues or organs to generate antibodies or other ligands. Ligand-targeting cell surface receptors can be selected on known tissue-specific receptors presented as recombinant proteins or receptors overexpressed on cultured cells. These can be subsequently evaluated in vivo as tissue homing ligands . Unfortunately, when removed from their microenvironment, isolated and cultured cells appear to lose much of their tissue-specific nature upon culture . In the case of cultured endothelial cells, some useful markers might not be produced but expressed instead by other cells in the vasculature and transported to the endothelium. Thus, the phenotype of endothelial cells is likely to change . This strategy is also restricted due in part to the difficulty in obtaining a pure population of endothelial cells from the heterogeneous tissue environment . As a result, this approach is not able to address the accessibility of the target molecule in the lumen of vessel. This drawback can be overcome by using in vivo phage selection. This involves the intravenous injection of the phage library into an animal and isolating phages that home to the appropriate organs . This allows the recovery of displayed peptides or antibodies homing preferentially to the target tissue or organ due to their binding to tissue-specific receptors. Such in vivo selection can be performed without the need to identify a tissue-specific receptor beforehand. The strategy is therefore unbiased and functionally controlled because the selection is based on the accessibility of the receptors to the circulating ligands. The use of such in vivo phage display technology has significantly contributed to the identification of targets and their corresponding ligands in a broad range of tissues, including the prostate, kidney, skin, pancreas, retina, intestine, uterus, and adrenal gland in mice . The identified peptides can later be chemically or genetically fused to synthetic or viral vectors. In this method, phage libraries are intravenously administered to mice through the tail vein for a predetermined circulation period, during which time suitable ligands home to vascular receptors, followed by removal and washing of the target tissue, elution, and amplification of recovered phage, which is then re-injected into mice for further rounds of selection. In order to identify the amino acid sequence conferring binding to vascular addresses, gene sequencing and homology analysis in protein sequence databases can be performed, and receptors can be deduced accordingly . However, tissue-specific protein expression patterns, ligand–receptor pairing, and accessibility issues might be different in human and mice. Thus, the ligands identified as specific for an animal might not bind to the human homologs . To identify molecules for human vasculature, two approaches have been proposed. The best method would be to perform selection in humans. However, such procedures are limited by ethical and practical considerations. The other approach is to establish human xenografts in immunodeficient animals. This technique has been widely employed in cancer research, in which human-derived tumors are grown in nude, SCID, or SCID/NOD mice. The growing tumor induces the formation of new blood vessels. Note that these blood vessels will be murine, so many of the identified targets will also be murine . In vivo phage selection has been successfully used in human tumor xenografts and has identified peptide ligands that appear to recognize both human and murine tumor vasculature . These include the double-cyclic arginine–glycine–aspartate ligand termed RGD4C, which recognizes and interacts with α v integrin receptors. The α v β 3 and α v β 5 integrins are overexpressed on the surface of activated endothelial cells during angiogenesis compared with quiescent established blood vessels .
Various classes of receptor expressed on angiogenic tumor-associated vascular cells are recognized by different peptide ligands identified by in vivo phage selection. Some of the targets appear to be protease enzymes such as aminopeptidase N/CD13 and aminopeptidase A, identified as angiogenic receptors for the NGR and CPRECESIC motifs , respectively. Furthermore, matrix metalloproteinases MMP-2/MMP-9 were identified as proteins specifically expressed in angiogenic endothelial cells and pericytes of both human and murine tissue origin. Targets such as the proteoglycan (NG2) and heat shock protein 90 kDa (HSP90) have also been identified by phage display technology . In addition, some surface proteins can serve in the tissue-specific homing of circulating ligand responsible for either normal development or pathological conditions, including vascular endothelial growth factor receptors and platelet-derived growth factor receptors . Some receptors can also function in the tissue-specific homing of leukocytes; for example, interleukin-11 receptor (IL-11Rα) was identified by the in vivo phage display approach in humans and found to be a potential target for intervention in human prostate cancer .
Ligand-Directed Vectors for Vascular-Targeted Gene Delivery
Phage-Based Vectors
Recently, bacteriophages have become more than just a simple means of identifying targeting ligands; they have become gene delivery carriers in their own right. The prokaryotic virus bacteriophage has been proposed as a safe vector for targeted systemic delivery of transgenes . To date, most gene therapy strategies have used eukaryotic viral vectors such as retroviruses, adenoviruses, adeno-associated viruses, and herpes simplex viruses as the vehicles of choice. They offer relatively high efficacy of gene transfer; however, several restrictions and risks associated with viruses have been found, including unfavorable immunogenicity and cytotoxicity, complexity of production, and limitation in size of the inserted gene, all of which limit gene therapy applications. Although progress has been made in improving these viral vectors, it is essential to investigate alternatives such as prokaryotic viruses (i.e., bacteriophage) and nonviral vectors . This discussion focuses on the application of filamentous bacteriophage to mediate gene delivery.
M13 filamentous bacteriophage, containing a core composed of circular single-stranded DNA encapsulated in approximately 2700 copies of the major coat protein and minor coat proteins capped at the tips of the virion, have been engineered to display a targeting ligand on the capsid and deliver genes to mammalian cells. By displaying foreign cell-specific ligands, such as fibroblast growth factor (FGF2), anti-ErbB2 scFv F5 antibody, or integrin-binding peptides, receptor-mediated internalization of phage vectors can be achieved in mammalian cells . In theory, the use of bacteriophage in this way has several advantages compared to the use of animal viruses. First, phages do not normally infect mammalian cells because there are no natural receptors for phage on mammalian cells. This allows phages to be genetically targeted without the elimination of native tropism. The genetic modification of phage vectors to display specific ligands results in selective binding to mammalian cells and subsequent receptor-mediated internalization . Second, bacteriophages have long been therapeutically administered intranasally, topically, orally, subcutaneously, and intravenously to humans to treat bacterial infections, and only minor side effects have been observed in more than 12 years of use . A formal safety study conducted by Harold Brussow at the Nestle Research Centre, Switzerland, demonstrated no safety concerns when bacteriophages were administered to human volunteers . Certain phage preparations have been approved by the U.S. Food and Drug Administration as antibacterial food additives under generally recognized as safe (GRAS) regulations based on experience in Europe . This acceptance is based on the presence of high levels of bacteriophages in the digestive tract of humans and throughout the natural environment. In addition, their high resistance to nonenzymatic degradation in comparison with other vehicles allows for longer and less costly storage . The production of phage vectors is simple and cost-effective because they are easily produced with high titers in the supernatant of Escherichia coli host cell cultures and can be purified on a large scale. The yield of phage particles secreted is approximately 300 mg/l (20 mg/l for the engineered phage) . In terms of their packaging capacity, bacteriophages do not seem to have a defined packaging limit because they are not limited in size by a preformed capsid but instead form their protein capsid as they are extruded from bacteria . Finally, unlike mammalian viruses, phages do not require further context modification of their capsid because the targeting peptides are actually selected and isolated directly after screening of a phage display peptide library.
Hybrid AAV/Phage
Despite these potential advantages, the efficiency of phage-mediated gene delivery has been considered poor compared to that of typical animal viral vectors because bacteriophage species have evolved to infect bacteria exclusively and have no mechanism for delivering transgenes to mammalian cells. In order to obtain the ideal gene delivery vector system, several attempts have been made to combine the attributes of two or more different types of vectors. In 2006, Hajitou and colleagues combined the advantages of two gene delivery vehicles, namely M13-derived fUSE5 filamentous bacteriophage and recombinant rAAV genome. The former serves as a vehicle displaying targeting peptides on the phage capsid in the structural context on which they were selected, and the latter has the potential for episomal amplification and chromosomal integration. They have thus produced a novel prokaryotic–eukaryotic hybrid vector termed AAV/phage or AAVP, which incorporates key elements of single-stranded bacteriophage and cis elements from AAV2 as described later. In this vector, there is no AAV capsid; instead, the bacteriophage particle serves as a shuttle to deliver the rAAV genome. As for most phage-mediated gene delivery, AAVP vectors have been used with targeting ligand on pIII because its flexible structure is well suited for displaying a variety of biologically active peptides and proteins while retaining the structural integrity of the phage particle . In the reported studies, the efficacy of the vector was assessed by using the cyclic RGD4C displayed on the phage capsid to target α v β 3 integrins overexpressed in the angiogenic blood vessels of animal tumors. After intravenous administration, the targeted RGD4C AAVP vector specifically and efficiently delivered imaging and therapeutic genes to the tumor vessels in mice, rats, and dogs while sparing the normal organs . This vector showed superior gene delivery compared to conventional phage-based vectors with long-term transgene expression in vivo . AAVP can thus be regarded as a new generation of phage vectors that has a multitude of potential applications.
Construction of the Targeted RGD4C AAVP
The use of phage-derived peptides for targeted gene delivery in the structural protein context in which they have been selected can be achieved by directly using the targeted phage particle to generate the AAVP vector without further capsid modifications. As mentioned previously, filamentous bacteriophages have been used for transduction of eukaryotic cells. However, broader application of phage vectors in vivo was prevented due to inherent limitations of the phage particle. A new hybrid AAVP vector was therefore generated to improve gene delivery. In summary, the ligand-directed bacteriophage selected and identified by the in vivo phage display screenings can serve as a platform to construct a tissue-targeted AAVP vector. The targeted bacteriophage is used to produce a phage multiple cloning site (MCS)-based M13 phage by inserting an MCS into an intergenic region of its genome followed by incorporating a recombinant rAAV genome into the MCS of the phage-MCS to generate the targeted AAVP vector. Phage particles are generated and purified from culture supernatants as reported previously . Next, vector particles in suspension are sterile-filtered through 0.45-μm filters. The number of infectious phage particles is expressed as transducing units, which can be determined by infecting K91kan E. coli , followed by selection for bacteria resistant to tetracycline.
In the targeted AAVP prototype vector, a mammalian DNA cassette driven by a cytomegalovirus promoter and flanked by ITRs from AAV was incorporated into the intergenic region of a bacteriophage vector displaying the double-cyclic RGD4C ligand on the minor pIII coat protein as schematically shown in Figure 33.1 . The incorporation of ITRs from AAV into bacteriophage is associated with improved fate of delivered transgenes, maintenance of the entire mammalian transgene cassette, better persistence of episomal DNA, and formation of concatamers of the transgene cassette, resulting in increased transduction efficiency compared to that of conventional phage-based vectors .

Vascular-Targeted Systemic Gene Therapy by RGD4C AAVP
Therapeutic efficiency of vascular-targeted RGD4C AAVP vector was first assessed using vector carrying a commonly used suicide gene, the herpes simplex virus type-1 thymidine kinase ( HSVtk ), and subsequent treatment with ganciclovir (GCV). The HSVtk -expressing cells phosphorylate GCV to its cytotoxic triphosphate derivative, which interferes with DNA replication and induces cell death. This suicide gene therapy has the advantage that in addition to affecting transduced cells expressing the suicide gene, adjacent nontransduced cancer cells are killed by the transfer of the converted cytotoxic drug and/or toxic metabolites between cells. This phenomenon, known as the “bystander effect,” may overcome the low tumor transduction and the need for all cells to be transduced in order to achieve therapy. Recently, it was reported that nontransduced tumor cells can be killed by transduced endothelial cells expressing HSVtk after systemic delivery of HSVtk to tumor endothelial cells through the targeted RGD4C AAVP. This phenomenon leads to suppression of tumor growth due to a heterotypic endothelial cell-mediated bystander effect through gap junctional intercellular communication between endothelial and tumor cells .
A number of preclinical experiments have been performed on models of cancer in immunocompromised animals representing a variety of species and histological origins. Targeted RGD4C AAVP vector carrying HSVtk was assessed in immunodeficient mice bearing human Karposi’s sarcoma (KS1767) cell-derived tumors in combination with the prodrug GCV. As a result, tumor growth was remarkably suppressed by a single intravenous administration of targeted RGD4C AAVP compared to nontargeted AAVP . Similarly, the suppression of tumor growth was observed in immunocompetent BALB/c mice bearing subcutaneous EF43 -FGF4 mammary tumors. As another test, the efficacy of RGD4C AAVP- HSVtk vector was evaluated in UC3-derived bladder carcinomas and DU145-derived prostate carcinomas in nude mice. Significant suppressive effects were observed in these models, regardless of the size of the xenograft being tested . RGD4C AAVP- HSVtk vector was also validated in a preclinical experimental model of human soft-tissue sarcoma xenografts in athymic rats (large rodents) and resulted in tumor growth inhibition . Histopathologic analysis showed extensive destruction of the central area of the tumor associated with disruption and apoptosis of tumor vasculature after 7 days post-administration of the RGD4C AAVP- HSVtk .
An additional experiment using the cancer-targeting properties of RGD4C AAVP to deliver the gene for the antitumor agent tumor necrosis factor-α (TNF-α) to the angiogenic vasculature of a human melanoma tumor model in nude mice was conducted by researchers at the National Cancer Institute (NCI) . The localization of RGD4C AAVP-TNF-α and the expression of TNF-α were observed in tumor endothelium after systemic administration. As a result, mice bearing melanoma xenografts had significant reductions in tumor volume without any evidence of systemic toxicity to control organs . Moreover, a recent study carried out under the direction of the NCI elegantly demonstrated the potential of this technology. Targeted RGD4C AAVP-TNF-α was used to deliver TNF-α to naturally occurring cancers diagnosed in dogs . In this study, single and repeated dosing using RGD4C AAVP-TNF-α proved safe in treated animals. Remarkably, repeated therapy resulted in complete tumor eradication in a few dogs with aggressive cancers such as soft-tissue sarcoma.
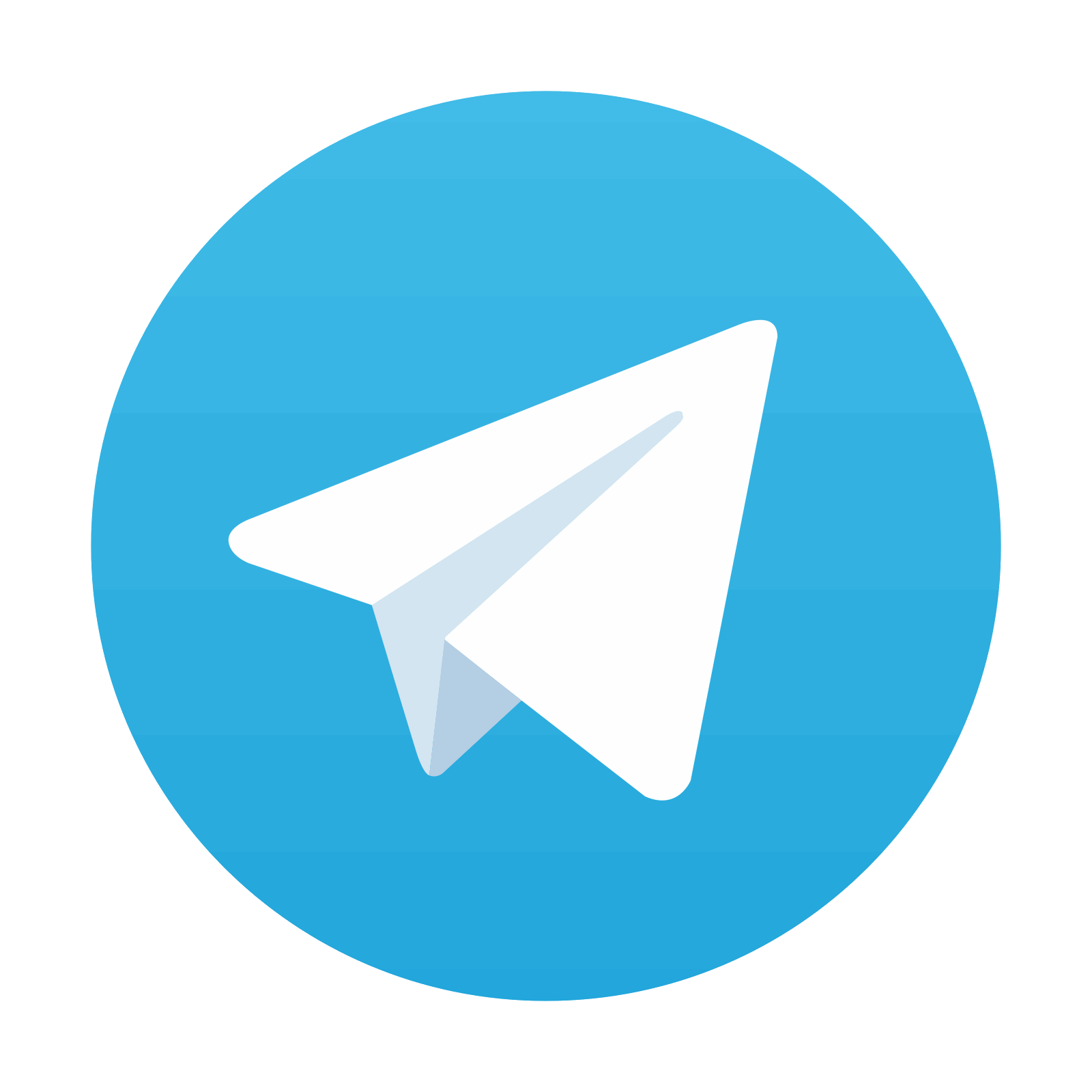
Stay updated, free articles. Join our Telegram channel

Full access? Get Clinical Tree
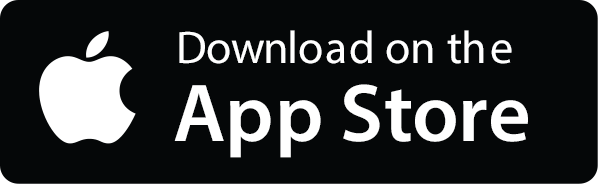
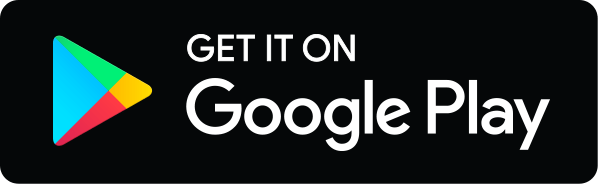