Chapter Outline
PRIMARY AUTOIMMUNE HEMOLYTIC ANEMIA
SECONDARY AUTOIMMUNE HEMOLYTIC ANEMIA
Autoimmune hemolytic anemia (AIHA) is an immunologic disorder in which antibodies are produced that target red blood cells (RBCs). AIHAs can be classified into four major types of disease: warm-reacting AIHA, cold agglutinin disease (CAD), paroxysmal cold hemoglobinuria (PCH), and mixed-type AIHA ( Box 13-1 ). Patients with no underlying associated diseases are classified as having primary or idiopathic AIHA. AIHA in patients with associated autoimmune diseases, immunodeficiencies, or infectious diseases is classified as secondary. Drug-induced and alloantibody-mediated immune hemolytic anemias are other important causes of antibody-mediated RBC destruction.
Autoimmune Hemolytic Anemias
Warm-reacting AIHA
Idiopathic
Secondary (systemic lupus erythematosus, immunodeficiency)
Cold agglutinin disease
Idiopathic
Secondary (infection, malignancy)
Paroxysmal cold hemoglobinuria
Idiopathic
Secondary (infection)
Mixed-type AIHA
Drug-Induced Immune Hemolytic Anemia
Drug-induced AIHA
Drug-related antibody
Alloantibody-Induced Immune Hemolytic Anemia
Hemolytic disease of the fetus and newborn
Hemolytic transfusion reaction
AIHA, Autoimmune hemolytic anemias.
Antibodies produced are of two major classes: immunoglobulin G (IgG) and immunoglobulin M (IgM). IgG-coated RBCs are recognized by macrophages with specific receptors on their surface that are efficient in binding and stimulating the ingestion of the IgG-coated cells. In addition to the clearance of IgG-coated RBCs by macrophages, patients with AIHA can also have IgM antibodies or, rarely, immunoglobulin A (IgA) antibodies directed at the RBC. The macrophage Fcγ receptors primarily responsible for the clearance of IgG-coated cells are FcγRI, FcγRIIIA, and FcγRIIA. IgM antibodies bound to the RBC surface can initiate activation of classic complement pathway, resulting in the deposition of complement components—usually C3b. C3b-coated RBCs are recognized by macrophage C3b receptors, which then internalize complement-coated RBCs. IgG antibodies, when present on the RBC surface at a sufficient antigen density, can also induce complement activation.
Finally, both IgG and IgM antibodies can induce the clearance of the antibody/complement-coated RBCs by tissue macrophages. The tissue macrophages responsible for the clearance of IgG-coated RBCs are present in the spleen. Hepatic macrophages can also bind C3b-coated RBCs and initiate their clearance. Rarely, RBCs coated with other antibody subclasses such as IgA can be present and induce the accelerated clearance of these RBCs, which occurs in a rare subset of patients with AIHA.
Primary AIHA
Incidence
Primary AIHA is relatively uncommon, with an annual incidence of about 1 per 75,000 to 80,000 persons. Within the pediatric population, AIHA is primarily a disease of young children, with a median age of diagnosis of 3.8 years in a recent national observational study. Primary AIHA also affects infants and toddlers, typically after a recent infection, whereas adolescents with AIHA are more likely to have an associated underlying systemic illness and to have secondary disease. Gender preference is unclear; some studies show an equal male to female ratio, whereas other authors report a slightly higher prevalence in young boys but equal prevalence between the sexes during adolescence. No evidence exists that AIHA affects persons of any particular racial background.
Warm-reactive antibodies are primarily of the IgG class and account for the majority of AIHA cases in children. IgM-mediated CAD is uncommon in children and mostly affects middle-aged adults and elderly persons. In contrast, PCH primarily presents in children, with the median age at diagnosis being 5 years.
Natural History
Young children with IgG warm-reactive AIHA can have an acute illness only but tend to have a chronic clinical course characterized by intermittent remissions and relapses. In contrast, patients with cold-reactive autoantibodies, a condition most commonly associated with PCH, may have severe but acute self-limited illness requiring short-term supportive care with transfusion.
Overall mortality rates of 11% to 18% were reported in older series of pediatric AIHA cases, but in a recent study of 265 pediatric patients with AIHA, only 4% of children died. The improved mortality rate is likely a result of improvement in supportive care and new immunosuppressive treatment options, such as rituximab. Pediatric patients who have primary AIHA associated with an infectious etiology have the most favorable outcome, with very low mortality and a high complete remission rate. In this largest cohort of 265 pediatric patients with AIHA, the median evaluable period was 3 years, and it was longer than 1 year for 198 children. More than one third (37%) were diagnosed with Evans syndrome. At the last follow-up, 4% had died, 6% had no response or were in partial remission, and 90% were in complete remission while either receiving or not receiving therapy. Because AIHA is a rare disease, data describing the incidence of acute versus chronic disease are lacking.
Several studies have attempted to define prognostic factors for pediatric AIHA. A positive mixed IgG/C3 direct antiglobulin test (DAT) is more likely associated with chronic disease as an independent prognostic risk factor. In earlier retrospective studies it was observed that younger children with an abrupt onset of symptoms have a better prognosis. In the context of current therapies, sex, age, and the triggering infection were not associated with continuous complete remission.
Clinical Manifestations
Patients with AIHA typically present with symptoms of anemia such as pallor or fatigue, and they sometimes present with jaundice and scleral icterus as a result of rapid erythrocyte destruction and increased bilirubin turnover. Dark urine is reported in up to 80% of cases. Hemoglobinuria is attributed to intravascular hemolysis of erythrocytes and therefore may occur in cases of cold AIHA as a result of IgM-mediated complement activation, but it is uncommon in persons with IgG-associated warm autoantibodies. The onset of symptoms can be rapid but the child is usually well compensated from a cardiovascular standpoint, and symptoms of congestive heart failure or circulatory collapse are rare. Occasionally fever or abdominal pain is reported by the patient.
Children with AIHA often have a benign medical history, but questions should be asked to determine whether a previous similar episode has occurred or if the child has had a recent viral illness. A history of infections, exposure to drugs or vaccination, or signs of immune disease may suggest secondary AIHA. The review of systems should include questions that address a possible underlying autoimmune disease, primary immunodeficiency, inflammatory disorder, or malignancy. The family history is often not revealing, but familial cases of AIHA have been reported and likely represent a familial predisposition to an autoimmune disorder such as systemic lupus erythematosus (SLE).
The physical examination can be normal in patients with mild anemia, but children with moderate to severe AIHA are usually pale and icteric. Jaundice, which may be particularly prominent when ongoing hemolysis is severe, is caused by the breakdown of bilirubin from the destroyed erythrocytes. However, it should be emphasized that complement-coated RBCs are cleared extravascularly and that RBCs are difficult to lyse after complement activation. With less brisk hemolysis, jaundice may be best appreciated in the eyes or in the palms or soles. A heart examination will show that most children are tachycardic and, depending on the degree of anemia, an early systolic flow murmur may be appreciated as a result of the high output anemic state. A gallop may be heard in severely anemic patients and is a sign of impending cardiac failure. Mild to moderate tender splenomegaly is common in patients with severe hemolytic anemia, and occasionally hepatomegaly may occur as well.
Intravascular hemolysis results in hemoglobinemia and hemoglobinuria when hemoglobin is released into the blood and immediately bound by haptoglobin for clearance in the liver. The rate of hemolysis may exceed haptoglobin production, and extra hemoglobin circulates freely, causing hemoglobinemia. Free hemoglobin is typically filtered in the kidneys and reabsorbed in the form of iron. However, if the hemolysis exceeds the reabsorptive capacity of the renal tubules, hemoglobinuria occurs and urinary hemosiderin is detected. Patients with intravascular hemolysis may experience acute abdominal pain, costovertebral angle tenderness, and fever. Extravascular hemolysis occurs via antibody-coated RBC clearance by splenic and liver macrophages. Patients often present with tender splenomegaly, and although hyperbilirubinemia and bilirubinuria are common, hemoglobinemia and hemoglobinuria are not characteristic.
Routine Laboratory Evaluation
By definition, patients with AIHA present with anemia that ranges from mild to life-threatening. Children with primary AIHA often present with marked anemia, with a hemoglobin concentration of 4 to 7 gm/dL and no apparent cardiovascular compromise. Most patients have a normal platelet count, mild leukocytosis, and neutrophilia, but occasionally patients have leukopenia, neutropenia, or thrombocytopenia. If the thrombocytopenia is significant, a diagnosis of Evans syndrome is possible and antiplatelet antibodies should be assayed. However, microangiopathic hemolytic anemias including thrombotic thrombocytopenic purpura (TTP) or hemolytic uremic syndrome (HUS) must also be considered with concurrent thrombocytopenia. The reticulocyte count is usually elevated to compensate for the anemia, but more than one third of patients have a transient reticulocytopenia despite having normal or hyperplastic erythroid marrow. The mechanism for reticulocytopenia is not well understood, but it has been suggested that a distinct autoantibody directed at erythroid progenitor cells leads to their selective destruction. Bone marrow examination is not typically indicated but shows erythroid hyperplasia in the majority of patients.
Evaluation of the peripheral blood smear can reveal important diagnostic hallmarks of AIHA. Spherocytes are usually present in IgG-mediated warm-reactive AIHA ( Fig. 13-1 ), which suggests an immune hemolytic process in the absence of hereditary spherocytosis. Spherocytes result from spleen-induced damage to antibody-coated erythrocytes and are most commonly seen with warm, IgG-mediated AIHA. Spherocytes are uncommon in cold-reactive AIHA, but complement-mediated immune spherocytosis can also result from surface microfragmentation of erythrocytes, and occasional to rare spherocytes are noted on the blood smear. Polychromasia can be appreciated and usually correlates to the degree of reticulocytosis. Other morphologic abnormalities include fragmented and nucleated RBCs. Erythrophagocytosis is a rare finding on the peripheral blood smear but demonstrates monocytes engulfing erythrocytes. Agglutination of RBCs is frequently seen in IgM-mediated cold-reactive AIHA when the binding affinity of the antibody reaches room temperature.

Serum chemistries may be abnormal as a result of erythrocyte hemolysis but are not essential to the diagnosis of AIHA. Elevated levels of lactate dehydrogenase and aspartate aminotransferase reflect erythrocyte destruction and release of cellular enzymes. In contrast, the serum level of hepatic enzymes alanine aminotransferase and gamma glutamyl transferase should not be elevated in persons with AIHA. The total serum bilirubin level is elevated in most patients with AIHA and is primarily unconjugated as a result of accelerated erythrocyte destruction rather than intrinsic hepatic disease. A decreased haptoglobin level is indicative of rapid intravascular hemolysis when the rate of hemolysis exceeds haptoglobin production by the liver. However, haptoglobin levels will be low in newborn infants who do not synthesize it well or high with concurrent infection or inflammation as an acute-phase reactant. Urinalysis may be unremarkable, but when hemoglobinuria is present, urine dipstick analysis shows the presence of blood, although microscopic examination reveals few if any erythrocytes. Urine hemosiderin is detected in patients with hemoglobinuria as a result of intravascular hemolysis and can be used to distinguish hemoglobinuria from hematuria and bilirubinuria.
Serologic Laboratory Evaluation
The DAT (formerly named the Coombs test) is a simple but important test to establish a suspected diagnosis of AIHA. The DAT detects immunoglobulin and/or complement on the surface of RBCs and aids in the classification of hemolysis as immune or non–immune mediated. The test was first reported in the early 1900s but its use became widespread after it was described in 1945 by Coombs and colleagues. Initially this test was used to demonstrate antibody in the serum, but it was later adapted to demonstrate the in vivo coating of RBCs with antibody or complement components.
IgM autoantibodies are pentameric and can bridge and agglutinate adjacent RBCs ( Fig. 13-2 ). In contrast, IgG autoantibodies are smaller and unable to bridge adjacent RBCs because of the surface repulsion between cells, known as the zeta potential. Antihuman globulin (AHG; Coombs reagent) binds to IgG autoantibodies on the RBCs, bridges the zeta potential, and causes agglutination.

The main principle of the DAT is that AHG agglutinates antibody-coated cells, as evidenced by erythrocyte clumping. Ethylenediaminetetraacetic acid (EDTA)–anticoagulated blood samples are preferred because EDTA prevents in vitro fixation of complement. The DAT is optimally performed with use of freshly washed RBCs; washing is necessary to remove proteins and complement that are present in the surrounding plasma and could neutralize the AHG reagent, causing a false-negative result. Typically a polyspecific AHG containing both anti-IgG and anticomplement is used first. A positive DAT result with polyspecific AHG leads to testing with monospecific IgG and complement. Commercial reagents of anti-IgG recognize the gamma heavy chain portion of IgG, and monospecific anticomplement reagents usually contain anti-C3d, which is indicative of bound IgM. The DAT is typically performed at room temperature (25°C) but can be performed at temperatures between 0°C and 37°C to determine thermal reactivity of the antibody. The DAT may be performed with the conventional test tube method, a gel microcolumn technique, or solid-phase methods ( Fig. 13-3 ).

The DAT results describe the pattern of agglutination with the polyspecific AHG reagent and, if positive, the results of subsequent testing with the specific IgG and C3 reagents. The DAT is positive when agglutination is observed either after immediate centrifugation or after centrifugation following a room-temperature incubation. IgG-coated RBCs usually agglutinate immediately, whereas complement-coated RBCs may be more easily demonstrable after incubation. The DAT may also be performed at different temperatures including 4°C, 10°C, 25°C (room temperature) and/or 37°C to distinguish warm and cold-reactive autoantibodies and to characterize their thermal reactivity and amplitude. DAT agglutination results are scored on a scale of 1 to 4.
The presence of IgG on the erythrocyte in a patient with suspected AIHA who has not undergone transfusion is good evidence for an IgG autoantibody. Warm-mediated AIHA is the most common form of AIHA in children and is usually caused by IgG autoantibodies that react at room temperature or 37°C. The DAT is positive for IgG, and simultaneous detection of complement indicates that the antibody can fix complement as well. Cold AIHA is typically caused by IgM autoantibodies that bind to RBCs at temperatures between 0°C and 37°C. A DAT that indicates the presence of complement alone suggests a cold-reactive antibody that fixes complement at lower temperatures but binds poorly to the erythrocyte at 37°C. Further serologic evaluation for the presence of an IgM autoantibody or the IgG Donath-Landsteiner autoantibody would be indicated (described in detail later in this chapter).
The DAT results may be negative despite good clinical evidence of autoimmune hemolysis. DAT-negative AIHA represents 5% to 10% of all AIHAs. One explanation is that the quantity of RBC-bound IgG is below the threshold of the DAT with standard reagents, which currently detects as few as 150 IgG molecules per erythrocyte. More sensitive assays to detect RBC-bound IgG may be helpful and include enzyme-linked immunosorbent assays, gel card analysis, and flow cytometry. Autoantibodies of the IgA or IgM subtypes may cause hemolysis with a negative DAT because the test is designed to detect IgG and C3d. Reagents that react with IgA or IgM are commercially available but are performed in reference immunohematology laboratories only. Clinically relevant low-affinity antibodies may be removed by washing during the DAT procedure. Washing with low ionic strength solution or with ice-cold saline solution rather than room temperature saline solution may help retain the antibody on the RBCs. Other technical issues may lead to false-negative DAT results. If clinical suspicion for AIHA is strong despite negative DAT results, specialized immunohematology reference laboratories such as those at the American Red Cross offer a DAT-negative AIHA evaluation that tests for IgA, IgM, and low-affinity antibodies.
Importantly, positive DAT results are not necessarily diagnostic. The interpretation and significance of positive DAT results must be correlated with the patient’s history, including recent drug or transfusion exposure. Positive DAT results are found in 1 in 1000 to 14,000 healthy donors without hemolysis and in 7% to 8% of hospitalized patients.
Differential Diagnosis
The differential diagnosis of warm AIHA includes other causes of spherocytic anemias; for example, hereditary spherocytosis (HS; see Chapter 16 ) has many common clinical features. HS is a hemolytic anemia caused by deficient attachments of the lipid bilayer to the underlying membrane skeleton that is most often due to partial deficiency of ankyrin or band 3 or beta spectrin and is less often due to deficiency of alpha spectrin or protein band 4.2. Most diagnoses of HS are made in childhood on the basis of a positive family history or as a result of a similar clinical presentation to AIHA of anemia, jaundice, and splenomegaly. DAT results are always negative in persons with HS. A flow cytometry assay directly targeting the HS molecular defect (the eosin-5′-maleimide binding test) distinguishes spherocytosis due to HS versus other causes, including AIHA. Clostridial sepsis, ABO hemolytic disease in neonates ( Chapter 3 ), severe burns or RBC thermal injuries, severe hypophosphatemia, and sometimes the hemolytic anemia associated with Wilson disease ( Chapter 11 ) or congenital dyserythropoietic anemia type 2 are other diseases characterized by spherocytic anemias.
In patients with hemolytic anemia and positive DAT results, several diagnoses should be considered ( Box 13-1 ). Serologic characterization of an autoantibody will help distinguish warm-reacting AIHA, cold-reactive autoantibody syndromes such as CAD and PCH, and mixed-type AIHA. In a patient who has undergone a recent transfusion, positive DAT results may reflect a newly formed alloantibody to donor erythrocytes, indicative of a delayed hemolytic transfusion reaction. Further characterization of the antibody can distinguish whether it is directed at donor or autologous RBCs.
Drugs can cause hemolytic anemia through an immune or nonimmune mechanism. Drug-induced immune hemolytic anemia (DIIHA) is rare, but more than 100 drugs have been implicated. Common drugs causing hemolytic anemia are piperacillin, cefotetan, and ceftriaxone, and they can mimic AIHA or delayed hemolytic transfusion reactions in some patients. Drug-induced hemolysis requires laboratory demonstration of (1) the patient antibody binding only to RBCs coated with the drug in vitro or (2) the patient antibody supporting deposition of complement on RBCs only when the drug, patient serum containing the antibody, and a source of fresh complement are simultaneously present with RBCs. Hemolysis ceases within days of discontinuation of the drug. Serologic evaluation for DIIHA can be performed by immunohematology reference laboratories; the Blood Center of Wisconsin and the American Red Cross (Southern California Blood Services Region) have particular expertise in this type of evaluation.
Spherocytes are typically found on blood smears in patients with warm AIHA, occasionally in patients with PCH, and rarely in patients with CAD. In other forms of acquired hemolytic anemia, spherocytes are rare on the peripheral blood smear, the DAT results are negative, and these disorders will be distinguished through the history and appropriate laboratory studies. Dark urine or hemoglobinuria may be a feature of paroxysmal nocturnal hemoglobinuria ( Chapter 14 ), which is distinguished by decreased levels of CD55 and CD59 on blood cells as detected by flow cytometry. Peripheral blood erythrocytes derived from the mutant clone lack the capacity to restrict cell-surface activation of the alternative pathway of complement and to block formation of the cytolytic membrane attack complex because of a deficiency of CD55 and CD59, respectively. Microangiopathic hemolytic anemias such as TTP and HUS are characterized by schistocytes and minimal spherocytosis and thus are easily distinguished on a peripheral blood smear. Moreover, thrombocytopenia is a common feature of TTP and HUS, whereas it is uncommon in persons with AIHA unless it is associated with Evans syndrome.
Characteristics of Erythrocyte Autoantibodies
Erythrocyte autoantibodies are generally categorized as “warm” or “cold” based on their thermal reactivity or amplitude ( Table 13-1 ). Warm AIHA is usually caused by IgG autoantibodies that are optimally reactive at 37°C, and CAD is typified by IgM autoantibodies that are optimally reactive at 4°C but react at higher temperatures of 30°C to 37°C as well. Some patients have mixed-type AIHA with both IgG warm and IgM cold autoantibodies. PCH is a form of cold-reacting AIHA caused by IgG autoantibodies; conversely, rare cases of warm AIHA caused by IgM autoantibodies have been described.
Characteristic | Warm Reactive | Paroxysmal Cold Hemoglobinuria | Cold Agglutinin Disease |
---|---|---|---|
Immunoglobulin | IgG | IgG | IgM |
Thermal reactivity | 37°C | 4°C | 4°C |
Fixes complement | ± | Yes | Yes |
DAT | |||
4°C | Not performed | IgG, C3 | C3 |
37°C | IgG ± C3 | C3 | C3 |
Plasma titer | Low/absent | Moderate | High |
Hemolysin | No | Yes | Variable |
Antigenic specificity | Rh and others | P | I/i |
Site of RBC destruction | Spleen | Intravascular | Liver, intravascular |
Warm-Reactive Autoantibodies
Warm-reactive autoantibodies are maximally reactive at 37°C and typically react with a patient’s own RBCs, as well as with all donor RBCs against which they are tested. In some cases a relative or absolute specificity is identified, of which Rh blood group system antigens are the most common. Warm-reactive autoantibodies are usually polyclonal IgG antibodies. The strength of the antiglobulin reaction and the rate of RBC destruction is variable but appears to be influenced by IgG subtype. Four subtypes of IgG antibodies exist and are designated IgG 1 , IgG 2 , IgG 3 , and IgG 4 . IgG 1 is the most commonly encountered subclass, either alone or in combination with other IgG subtypes. IgG 1 and IgG 3 autoantibodies activate C1 more readily, bind strongly to FcγRI, FcγRII, and FcγRIII, and increase RBC destruction in comparison with IgG 2 and IgG 4 subtypes.
DAT results in persons with warm reactive AIHA may be positive for IgG plus complement (67%); IgG only (20%); or complement only (13%). If the autoantibody has been adsorbed by the patient’s RBCs in vivo, the serum may not contain detectable free antibody, resulting in a negative indirect antiglobulin test. However, the presence of an IgG autoantibody bound to the erythrocytes may be confirmed by elution of antibodies from the patient’s RBCs. Either autoantibodies eluted from the patient’s RBCs or those present in serum typically bind to all common RBCs in blood bank test panels. This condition is described as a “panagglutinin” or having no antigenic specificity. The eluate will usually have no serologic reactivity if the only protein coating the RBCs is complement.
In many cases, warm autoantibodies demonstrate a relative specificity for a blood group antigen, most commonly a member of the Rh blood group system. The patient’s serum or eluate often has broad specificity in the Rh system but may be weaker or nonreactive if tests are performed with cells of rare Rh phenotypes, such as Rh null RBCs that lack the entire Rh complex. Apparent or clear specificity for one of the common Rh antigens (anti-e, anti-E, or anti-c) is occasionally seen, or more commonly a “relative” specificity is demonstrated in which reactivity is stronger with certain Rh phenotypes. Warm autoantibodies in the Kell, LW, Kidd, Duffy, and Diego systems are rare but have also been reported. Autoantibodies against non–blood group antigens are extremely rare and include protein 4.1 and band 3. When a relative specificity is identified, transfusion of RBCs lacking the antigen may be useful.
RBC autoantibodies of the IgA class are found in up to 14% of patients with warm AIHA. In cases of DAT-negative AIHA, an isolated IgA autoantibody on the RBCs can occur. However, autoimmune hemolysis due to IgA antibodies alone is rare and more commonly acts synergistically with other immunoglobulins (usually IgG) and complement. AIHA due to warm IgM autoantibodies is rare but is associated with severe hemolysis that often fails to respond to standard therapies in both adults and children and may be fatal. These cases are difficult to diagnose because the routine DAT does not detect IgM antibodies, and thus misdiagnosis with CAD or PCH may occur if the DAT is positive for C3 only. Serologically, these IgM antibodies appear to have high affinity, resisting RBC dissociation and causing irreversible autoagglutination at 37°C. RBC autoagglutination of the sample should raise the suspicion of IgM autoantibodies, which do not disaggregate despite washing with warm (37°C) saline solution. Dithiothreitol (a reducing agent that inactivates IgM antibodies) does not affect IgG, and treatment of RBCs can be used to define the immunoglobulin type. Reference laboratories can distinguish IgM warm autoantibodies by routine tube methods with anti-IgM reagents or by flow cytometry.
Of three cases of pediatric AIHA attributed to warm IgM autoantibodies in contemporary literature, two were fatal despite treatment with corticosteroids, intravenous immunoglobulin (IVIG), RBC transfusions, whole blood exchange transfusions, rituximab anti-CD20 antibody, cyclophosphamide, mycophenolate mofetil (MMF), and cyclosporin A. Successful treatment of severe warm-reactive IgM-mediated AIHA with rituximab in a child with common variable immunodeficiency (CVID) has been reported. In patients who have warm IgM-mediated AIHA that does not respond to standard therapies, resulting in severe AIHA, the anti-C5-9 monoclonal antibody eculizumab is now available; however, results of its use in persons with AIHA have not yet been published. Notably, Salama and Mueller-Eckhardt reported 12 cases of warm AIHA associated with non–complement binding IgM autoantibodies that were referred to their reference immunohematology laboratory for serologic evaluation as a result of observed hemolysis that could not be explained by an underlying RBC abnormality; standard DAT results were negative. These patients were treated with blood transfusions and prednisone only, and definitive improvement or remission of hemolysis occurred in 11 of 12 cases; none was fatal.
Cold-Reactive Autoantibodies
Cold-reactive autoantibodies are primarily associated with CAD and PCH. Most commonly, CAD affects adults and PCH affects young children. PCH is the most common form of cold-reactive AIHA in children, but postinfectious CAD also occurs in childhood and adolescence, albeit rarely. Both are caused by autoantibodies that bind RBCs optimally at temperatures below 37°C and usually below 31°C. The associated hemolysis occurs through fixation of complement. In general, CAD and PCH may be idiopathic or secondary to an underlying systemic illness such as neoplasm or infection. Cold agglutinins are IgM autoantibodies that primarily cause extravascular hemolysis but can mediate intravascular hemolysis as well, whereas PCH is IgG mediated and causes intravascular hemolysis ( Table 13-1 ).
The pathogenesis of a cold-reactive autoantibody is dependent on its ability to bind host RBCs and activate complement. Although in vitro agglutination may occur maximally at 4°C in vitro, complement fixation by these antibodies occurs at 20°C to 25°C or higher. Complement fixation may cause RBC destruction via opsonization by macrophages in the reticuloendothelial system or rarely by direct lysis. With direct lysis, the Fc portion of the immunoglobulin interacts with C1q, the first component of the complement cascade. One IgM molecule is sufficient to bind C1q and activate the propagation from C1 to C9 sequence on the RBC membrane. Such direct lysis results in intravascular hemolysis, leading to hemoglobinemia and hemoglobinuria. Extravascular hemolysis occurs when the complement cascade leaves opsonic fragments of C3 and C4 on the cell surface, which is then ingested by macrophages in the liver or spleen. The ingested RBC may be engulfed entirely or released back into the circulation as a spherocyte after losing some of its membrane, providing an explanation for the observation of occasional spherocytes with IgM-mediated AIHA.
Cold Agglutinin Disease.
Cold agglutinins were first described by Landsteiner in 1903, but CAD was not distinguished as a distinct hemolytic anemia until the 1950s. CAD is uncommon in children, but when it does occur it is acute and often a result of Mycoplasma pneumoniae infection. Postinfectious cold agglutinins can also be seen with Epstein-Barr virus (EBV), varicella, and adenovirus. Postinfectious CAD is caused by polyclonal IgM antibodies, in contrast to monoclonal IgM that is seen classically in older adults with chronic CAD that commonly occurs as a result of an underlying lymphoproliferative disorder. CAD typically occurs in the second or third week of illness, and a rapid onset of hemolysis causes the patient to experience jaundice and pallor. Hemoglobinuria and acrocyanosis are not characteristically present in persons with postinfectious CAD. The hemolysis is self-limiting, and the degree of anemia is typically mild to moderate. The cold autoantibody is an IgM with a characteristic anti-I specificity associated with M. pneumoniae and anti-i specificity associated with EBV. Patients with postinfectious CAD will demonstrate high (≥256) cold agglutinin titers (although not nearly as high as in the chronic, adult form of CAD, with titers ≥1000), and they will have negative Donath-Landsteiner test results. Treatment of postinfectious CAD is supportive and includes keeping the patient warm and using a blood warmer if the degree of anemia warrants RBC transfusion.
In contrast, the chronic form of CAD is seen in elderly patients and is associated with lymphoma, chronic lymphocytic anemia, or Waldenström macroglobulinemia. The autoantibody is of the IgM subtype, is often monoclonal, and is maximally reactive in the cold but has reactivity up to at least 30°C. Patients with the chronic form experience hemoglobinuria from intravascular hemolysis and acrocyanosis of the ears, nose, fingers, and toes from autoagglutination of RBCs in the skin capillaries, particularly in cold weather. Autoagglutination of anticoagulated blood that occurs as the sample of blood cools to room temperature is characteristic and is frequently the first observation made to suggest the diagnosis ( Fig. 13-1, C ). Autoagglutination can be enhanced by cooling the blood to 4°C and is reversed by warming to 37°C.
Cold-reactive IgM binds to RBCs at lower temperature in the peripheral circulation and causes complement fixation to the RBCs. However, as the blood circulates to warmer regions of body, the IgM dissociates but the complement remains. Thus complement is the only protein detected on the erythrocytes in almost all cases of CAD (postinfectious or chronic). If the RBCs have been collected properly, maintained strictly at 37°C until the serum and red cells are separated, and washed at 37°C, there should be no immunoglobulin detected on the RBCs and no reactivity in the eluate. The majority of IgM cold-reactive autoagglutinins associated with immune hemolysis should have reactivity up to at least 30°C in saline solution or albumin and have a cold agglutinin titer of greater than or equal to 256 when tested at 4°C. Cold agglutinin IgM molecules are typically directed against the I/i RBC antigens. Importantly, the observation of a cold agglutinin alone is not sufficient to diagnose CAD.
Paroxysmal Cold Hemoglobinuria.
Historically, PCH was associated with syphilis and was named for paroxysms of constitutional symptoms and hemoglobinuria precipitated by cold temperatures. PCH now occurs primarily in children as an acute transient condition following an upper respiratory or viral infection and is detected by the Donath-Landsteiner test. PCH is the second most common form of AIHA in children, with a median age of 5 years. The precise incidence and prevalence of PCH in children is unknown. The transient nature of the Donath-Landsteiner antibody, the availability of the Donath-Landsteiner test, and the level of awareness among physicians all affect the recognition and correct diagnosis of PCH.
In children, PCH classically presents within several weeks after a viral infection with the sudden onset of hemoglobinuria, accompanied by fever, pallor, and jaundice. Symptoms of PCH in children are not precipitated by cold exposure, in contrast to the chronic, syphilitic PCH in adults. A specific etiologic agent is not usually identified, but an upper respiratory tract infection precedes the hemolysis in the majority of cases. The intravascular hemolysis and a rapidly progressing anemia may also cause headache, abdominal pain, nausea, vomiting, or diarrhea. Hepatomegaly and splenomegaly were reported in up to 25% of cases. The degree of anemia is variable in children but can be severe. Approximately one third of patients have hemoglobin levels of 5 gm/dL or lower at presentation, which may further decrease during their clinical course. Reticulocytosis is characteristic, but reticulocytopenia can be observed early in the course of disease and may result from viral suppression of the bone marrow or destruction by the autoantibody. The peripheral blood smear demonstrates RBC agglutination, polychromasia, nucleated RBCs, and occasional spherocytes. The DAT is usually positive for complement only but can be negative for both C3d and IgG. Other laboratory findings include increased levels of lactate dehydrogenase and unconjugated bilirubin, decreased or absent haptoglobin, and increased levels of blood urea nitrogen and serum creatinine.
The Donath-Landsteiner antibody is an IgG biphasic hemolysin that binds to RBCs and fixes complement at lower temperatures but does not cause hemolysis until the complement-coated RBCs are warmed to near 37°C. Between these temperatures, the autoantibody may dissociate. As a result, the DAT is positive with anti-C3 but is usually negative with anti-IgG unless performed at colder temperatures. Donath-Landsteiner antibodies do not interfere in routine pretransfusion and compatibility testing because the autoantibody rarely causes RBC agglutination above 20°C. For the same reason, the antibody screen is usually negative. The autoantibody may agglutinate normal red cells at 4°C, but in contrast to CAD, rarely to titers greater than 64. The Donath-Landsteiner antibody usually has specificity for the P blood group antigen, but other antibody specificities have been reported. Many patients with unexplained hemolytic anemia, often with negative DAT results, probably have undiagnosed PCH. The biphasic antibody may be transient and detectable only during the phase of acute hemolysis, and thus in suspected cases the Donath-Landsteiner test should be carried out as early as possible.
To confirm the diagnosis of PCH, the Donath-Landsteiner test can be performed by the hospital blood bank or reference immunohematology laboratory. A fresh sample of blood from the patient must be collected and maintained at 37°C until the serum is separated from the RBCs. Three sets of test tubes, labeled A1-A2-A3, B1-B2-B3, and C1-C2-C3, are used to mix serum with washed group O RBCs that express the P antigen. Tubes 1 and 2 of each set contain the patient’s serum, and tubes 2 and 3 contain fresh normal serum that has normal complement levels. One volume of 50% suspension of washed P + RBCs is added to each tube, and all the tubes are mixed. After mixing, the three “A” tubes are placed in a bath of melting ice for 30 minutes and then at 37°C for 1 hour. The three “B” tubes are placed in a bath of melting ice for 30 minutes and kept in melting ice for 90 minutes. The three “C” tubes are placed and kept at 37°C for 90 minutes. After the 90 minutes, the tubes are centrifuged and the supernatant fluids are examined for hemolysis. The test is considered positive when the patient’s serum, with or without added complement from normal serum, causes hemolysis in the tubes that were first incubated in the melting ice and then at 37°C (tubes A1 and/or A2) and there is no hemolysis in any of the tubes maintained at 37°C (tubes C1 and C2) or in melting ice (tubes B1 and B2) ( Table 13-2 ). Tubes A3, B3, and C3 serve as controls with normal serum and should not demonstrate hemolysis.
Tube 1 Patient Serum | Tube 2 Patient + Normal Serum | Tube 3 Normal Serum | |
---|---|---|---|
Tube A Ice bath and then 37°C | + | + | 0 |
Tube B Ice bath only | 0 | 0 | 0 |
Tube C 37°C only | 0 | 0 | 0 |
* The patient’s sample should be kept at 37°C until the serum and red cells are separated to avoid the loss of autoantibody by cold autoadsorption prior to testing.
PCH usually resolves spontaneously within several weeks and does not recur. Treatment is supportive with transfusion if the anemia is severe. Although the specificity of the Donath-Lansteiner antibody is almost always directed at the P antigen, RBCs lacking the P antigen are rare and not available for urgent transfusion. Most patients requiring transfusion achieve an adequate posttransfusion hemoglobin and hematocrit with P + RBCs despite the presumed or documented incompatibility. Although children with PCH do not typically have cold-induced hemolytic symptoms, the patient should be kept warm and the use of a blood warmer for RBC transfusions is prudent despite the lack of data to support this practice. Steroids are often initiated empirically for treatment of AIHA, but because no evidence exists to support their benefit, they can be discontinued once the diagnosis of PCH is confirmed.
Immune Clearance of Sensitized Erythrocytes.
The role of antibodies, complement, monocytes, and the reticuloendothelial system in the immune-mediated clearance of RBCs was elucidated more than four decades ago. In the 1970s, a guinea pig model for AIHA was developed that allowed the study of RBC clearance as a result of antibody or complement. IgG-coated erythrocytes were removed predominantly by the spleen, and the amount of surface IgG correlated with the rate of RBC clearance. In contrast, IgM-coated erythrocytes were sequestered and cleared by the liver. Again, the amount of bound IgM correlated with the rate of RBC clearance. These studies established that clearance of IgM-bound erythrocytes was dependent on the interaction of erythrocyte-bound complement fragments with specific receptors on hepatic macrophages. Human monocyte monolayer assays have been developed to correlate in vivo hemolysis with RBC autoantibodies, but these tests have shown limited utility. Flow cytometry can also be used to measure the amount of RBC-bound IgG but are also unable to define a clear quantitative threshold to correlate in vivo hemolysis.
Therapy
AIHA is a potentially fatal condition, and the decision regarding treatment depends on the severity and rapidity with which the anemia develops. Because of the relative rarity of the disease, evidence-based treatment of AIHA is limited, particularly evidence regarding pediatric management. No randomized studies and only a few prospective phase 2 trials have been conducted in adults or children. Most reports describe retrospective studies or small series of patients or are single case reports. Comparison of different treatment modalities is challenged by the fact that no formal consensus exists with regard to the definition of complete or partial hematologic response, remission, or refractoriness. Thus most treatment decisions, including whether to provide treatment, are made on the basis of experience and on an individual patient basis. The two major determinants for treatment are the type of antibody involved (IgG vs. IgM mediated) and whether the AIHA is primary or secondary. Hospitalization is almost always required.
Treatment of Acute AIHA
Transfusion Therapy.
In the patient with acute, newly diagnosed, or recurrent AIHA, the clinician must first decide whether the patient needs a transfusion immediately. A child who has relatively mild anemia with a hemoglobin level of 9 to 12 gm/dL may require observation alone. If the patient has a moderate to severe degree of anemia with a hemoglobin level of 6 to 9 gm/dL or is symptomatic with lethargy or shortness of breath, or if the rate of decline is precipitous, transfusion therapy is indicated. For patients who have very severe anemia with hemoglobin levels lower than 6 gm/dL, RBC transfusion should be instituted as soon as possible. In cases of warm, idiopathic AIHA, the antibody is typically directed against blood group antigens that are present on most RBCs. Therefore no truly matched RBC units are possible, but transfusion can be safely performed if underlying alloantibodies are excluded. In children and adolescents, clinically significant alloimmunization should only have occurred if they previously underwent transfusion, were pregnant, or received an organ transplant. A patient who has not previously undergone transfusion should have no risk of underlying alloantibodies and thus that lack of risk should be weighed against the risk of withholding transfusion for a time-consuming blood bank alloantibody evaluation in a severely ill child.
In a patient who has previously undergone a transfusion or been pregnant, the presence of an underlying alloantibody must be assessed to provide appropriate RBCs. Patients with AIHA that is associated with warm autoantibodies have an alloimmunization rate between 15% and 45%, but these studies focused primarily on adult patients. If IgG autoantibodies are demonstrable in the serum, they will typically interfere with routine pretransfusion antibody screening and compatibility testing. IgG antibodies in persons with PCH do not interfere with routine compatibility testing because the autoantibodies do not cause agglutination above 20°C. Autoadsorption or alloadsorption procedures are performed to remove the patient’s autoantibody from their serum sample by adsorption with autologous or allogeneic RBCs, thereby allowing identification of any remaining alloantibody in the adsorbed serum. Autoadsorption is generally preferred because removal of antibody activity by adsorption with autologous RBCs confirms that the antibodies removed are autoantibodies rather than alloantibodies. Autologous adsorption is not recommended for patients who have undergone a transfusion within the past 3 months because the blood sample may contain some transfused RBCs that could adsorb alloantibody, even if only a small amount of transfused RBCs are remaining. The patient’s RBCs are typically warmed to dissociate some of the bound autoantibodies and then treated with a mixture of enzymes to enhance their capacity to adsorb autoantibody from the serum sample in vitro.
Alloadsorption techniques are indicated when insufficient autologous RBCs are available from a very anemic patient or if the patient has undergone a transfusion in the past 3 months. Allogeneic RBCs used for autoantibody adsorption must lack the antigen(s) against which the alloantibodies react. Because the underlying antibody specificity is usually unknown, a panel of RBCs with different phenotypes must be used to adsorb several aliquots of the patient’s serum. RBC selection is based on antigens for which alloantibodies of clinical significance are likely to be present. These antigens include the common Rh antigens (D, C, E, c, and e), K, Fy a and Fy b , Jk a and Jk b , and S and s. Adsorption techniques are time-consuming and require a minimum of 2 to 3 hours to exclude the presence of alloantibodies but could take up to 4 to 6 hours because each aliquot of serum may need to be adsorbed two to three times to remove all of the autoantibody. Moreover, many hospital blood banks do not perform adsorption techniques and rely on reference laboratories, particularly for allogeneic adsorptions that require a supply of fully phenotyped RBC reagents.
Because adsorption procedures are labor-intensive, some transfusion services choose to prophylactically provide RBCs that are phenotypically matched with the patient’s Rh, Kell, and Kidd system antigens rather than searching for new alloantibodies. However, although the most clinically significant antigens may be matched, the potential exists to miss newly developed alloantibodies against other blood group antigens. Shirey and colleagues reported that prophylactic antigen-matched donor RBCs for patients with warm AIHA can obviate the need for adsorption studies in some but not all cases and that provision of extended antigen-matched RBCs is feasible and safe. In 12 of 20 adult patients with autoantibodies (including six with existing alloantibodies), a serologic phenotype was successfully obtained, a total of 149 prophylactic antigen-matched RBC units were transfused, and adsorption studies were avoided on 51 pretransfusion samples. Autoantibodies in the remaining eight patients interfered with determination of a complete RBC phenotype, and adsorption studies were required before transfusion. In both groups, Shirey and colleagues reported expected rises in hemoglobin and hematocrit values and no clinical signs of hemolysis as a result of transfusion, but these signs are probably difficult to assess in patients for whom RBC survival is already expected to be shortened. With increasing availability of molecular methods to type RBCs, which have the potential for a short turnaround period, prophylactic extended antigen-matched transfusions using RBC blood group genotyping is a reasonable option for patients with autoantibodies.
Once the blood bank has determined the desired RBC phenotype for a particular patient, the patient’s serum will be used to perform crossmatches with the RBC units. If the antigenic specificity is panreactive, the crossmatch will likely identify no units of blood that are fully compatible. Units that are “least incompatible” with the patient’s serum are typically provided for transfusion. Thus to prevent a hemolytic transfusion reaction, the most important consideration is to exclude potentially clinically significant alloantibodies before selecting RBCs for the patient. In an urgent situation, the clinician must balance the risk of delaying transfusion to complete an alloantibody evaluation against the benefit of an RBC transfusion in a severely anemic patient.
Corticosteroid Therapy.
Corticosteroids are the mainstay of treatment for persons with newly diagnosed primary idiopathic warm AIHA ( Table 13-3 ). Corticosteroids improve hemolysis in patients with warm AIHA via several mechanisms. Patients who respond to corticosteroids show a decrease in serum antibody concentration, which rises if the dose of prednisone is insufficient. The guinea pig model of AIHA demonstrated that corticosteroids increase the survival of IgG-sensitized RBCs by decreasing sequestration in the spleen. The ability of corticosteroids to suppress sequestration of RBCs by splenic macrophages may be the result of the reduced number of Fcγ receptors observed on peripheral blood monocytes of patients with AIHA during steroid treatment.
Acute First Line | Second Line | Third Line | Refractory Cases | |
---|---|---|---|---|
Primary warm AIHA | Corticosteroids | Rituximab IVIG Splenectomy | Cyclosporin Azathioprine Mycophenolate mofetil Cyclophosphamide Danazol | Campath-1H High-dose cyclophosphamide |
Paroxysmal cold hemoglobinuria | Supportive care | Corticosteroids | Rituximab | |
Cold agglutinin disease | Avoidance of cold Plasma exchange | Rituximab | Eculizumab Bortezomib |
In a patient with acute warm AIHA or PCH, intravenous methylprednisolone at a dose of 1 to 2 mg/kg is typically given every 6 hours for 1 to 3 days. In an adolescent, adult guidelines for warm AIHA recommend 1 mg/kg/day of oral prednisone or intravenous methylprednisolone, because higher doses result in hypertension, hyperglycemia, acute irritability, or psychoses. Once the patient’s hemoglobin level begins to rise and is clinically stable, oral prednisone should be used at a dose of 2 mg/kg/day for children and 1 mg/kg/day for adolescents. High doses of steroids are usually required for 2 to 4 weeks, and then the amount of corticosteroids must be reduced gradually to prevent relapses. For example, once the patient is taking 1 to 2 mg/kg/day of prednisone, a 10% to 20% taper with each dose change is reasonable. Some physicians first switch to an every other day schedule without reducing the total dose by administering a higher dose on even days and a lower dose on odd days. They then taper slowly on the alternate day schedule. Tapering of the corticosteroids is guided by the patient’s hemoglobin concentration and reticulocyte count and, to a lesser degree, the DAT results. Most importantly, the steroid taper must be performed slowly over a long period to avoid relapse. When a patient relapses, a very high dose of prednisone is usually required to achieve remission again. Ultimately, the goal is to maintain a stable hemoglobin count with a relatively low dose of corticosteroids and, preferably, an every-other-day regimen. An overall clinical response is achieved in approximately 80% of patients with warm AIHA. The total duration of corticosteroid therapy can vary from 3 to 12 months after remission is achieved.
Adverse effects of prolonged corticosteroid use should be considered. These adverse effects include irritability or even psychoses, sleep disturbances, hypertension, hyperglycemia that may require insulin therapy, weight gain, impaired longitudinal growth, and osteoporosis. Patients who are treated with prolonged courses of glucocorticoids should also take calcium and vitamin D supplements and be screened for osteopenia or osteoporosis if their diet and lifestyle place them at particular risk for these conditions.
Intravenous Immunoglobulin.
Although IVIG provides potent blockade of the reticuloendothelial system in persons with immune thrombocytopenic purpura (ITP), an inconsistent benefit has been shown for patients with AIHA. The largest cohort of patients studied included 37 cases treated with IVIG in pilot studies from three institutions, combined with a review of 36 cases in the literature, both adult and pediatric. Overall, all but four patients had received or were receiving prednisone therapy at the time of IVIG treatment, and nearly two thirds had received some previous therapy for AIHA. Patients received a range of IVIG doses, including 0.5 gm/kg/day for 5 days to 1 gm/kg/day for 5 to 7 days in the pilot studies, and a range of 0.4 to 2 gm/kg/day for 2 to 5 days in published cases. Overall, 40% had a response as measured by a rise in the hemoglobin level of greater than 2 gm/dL within 10 days of IVIG initiation. Six of 11 pediatric patients (55%) responded to IVIG, but whether they were receiving combined corticosteroid therapy is not reported. Although based on a small number of cases, high-dose IVIG (a 5 gm/kg total dose over 5 days) has been shown to have limited efficacy for the treatment of AIHA. In general, IVIG is not considered first-line treatment for AIHA in children, but it may be considered in a patient who is not responding to steroids.
Therapeutic Plasma Exchange.
The role of therapeutic plasma exchange (TPE) in patients with warm AIHA is considered a category III intervention by the American Society of Apheresis, meaning it may be beneficial but insufficient evidence exists to establish efficacy. Individual cases have been reported in which plasmapheresis has been efficacious. Whether TPE increases the survival of transfused RBCs in patients with severe hemolysis is controversial. Because IgG is mostly extravascular and is absorbed onto RBCs at body temperature, TPE may not efficiently remove the RBC autoantibodies. In contrast, IgM is mostly intravascular and binds poorly to RBCs at body temperature, and thus in persons with CAD, TPE can significantly reduce antibody titers, which are higher, particularly in adults. Whether TPE increases the efficacy of RBC transfusions in patients with severe hemolysis is controversial. However, plasmapheresis may be considered as a temporizing measure in a severely ill child who has a suboptimal response to RBC transfusion and may not have had time to respond to corticosteroid therapy.
Treatment of Chronic or Refractory AIHA
For a child with chronic or refractory AIHA, more aggressive therapy is often required to maintain a stable hemoglobin and quality of life. Long-term use of corticosteroids is not desirable given the numerous adverse effects. Several additional therapeutic options should be considered and should be individualized for each patient based on his or her hematologic response and the adverse effects. Splenectomy and rituximab are the only second-line treatments with proven short-term efficacy.
Rituximab.
Rituximab is a chimeric human IgG1/K monoclonal antibody specific for the CD20 antigen, which is expressed on B lymphocytes. It induces rapid in vivo depletion of both normal B lymphocytes and lymphoma B cells. Its mechanism of action includes complement-mediated cytotoxicity, inhibition of B-cell proliferation, and induction of apoptosis. Because of its clinical efficacy in B-cell lymphoma and its limited toxicity profile, its use has expanded to many autoimmune diseases, as well as benign hematologic diseases. Three cohort studies have described the use of rituximab for refractory AIHA in 25 children, all of whom failed to respond to conventional therapies. The standard dose of rituximab (375 mg/m 2 ) was administered weekly for 1 to 6 weeks (a median of four doses). More than 90% of patients had a complete response that lasted 7 to 28 months (a median of 16 months), and only three responders experienced a relapse. All three children who experienced a relapse were treated with another course of rituximab and achieved remission. Numerous single case reports and case series regarding the use of rituximab for AIHA in pediatric patients have been published, and nearly all reported a complete response. In the largest study by Zecca and colleagues, it was reported that 13 of 15 children (87%) with refractory AIHA responded to treatment with at least a 1.5 gm/dL increase in hemoglobin level observed at a median of 12 days from the time the first dose of rituximab was administered (range, 5 to 72 days). In the responders, a decrease in the absolute reticulocyte count of more than 50% occurred at a median of 21 days (range, 5 to 82 days). Most patients responded within 2 to 4 weeks from the time of the initial dose. Other therapies should be considered if no hematologic response occurs within 2 months. Given the high degree of efficacy of rituximab in treating refractory AIHA in pediatric patients, early use should be considered, and its use should also be considered for patients who respond to steroids but have significant adverse effects. Patients taking corticosteroids before the initiation of rituximab should continue to take steroids until a response to rituximab is clearly established. Although published data that compare rituximab or splenectomy as a second-line therapy are lacking, the trend has been to use rituximab first.
From a safety perspective, rare complications of rituximab include severe infusion reactions, infection, progressive multifocal leukoencephalopathy, and reactivation of hepatitis B and fulminant hepatitis. Untreated hepatitis B infection is a contraindication to rituximab therapy. Because treatment with rituximab causes marked B-cell depletion, with levels remaining low for 2 to 6 months and returning to pretreatment levels after 6 to 12 months, immunizations should be delayed until the B-cell levels return to baseline. After rituximab treatment younger children appear to have a higher risk of transient hypogammaglobulinemia, which can be treated with prophylactic administration of IVIG.
Splenectomy.
Splenectomy results in short-term complete and partial response in approximately two thirds of patients. No long-term follow-up data are available for patients with AIHA who were treated with splenectomy, but approximately 50% of patients will remain in remission for years. In 53 patients with primary and secondary AIHA who underwent splenectomy, 63% had a hematologic remission defined by a hematocrit of greater than 30% without concurrent corticosteroid therapy after a mean follow-up of 33 months. The perioperative risk of splenectomy is relatively low, because most cases can be performed laparoscopically. In a large national study, the mortality and morbidity of laparoscopic splenectomy for hematologic indications was 0.6% and 18%, respectively. Complications included fever (7%), pleural effusion (4%), and actual or suspected hemorrhage (4%).
Because of the risk of postsplenectomy sepsis with encapsulated bacterial organisms (see Chapter 16 ), prior to surgery children should be immunized with the polyvalent polysaccharide vaccines against Streptococcus pneumoniae and Neisseria meningitides in addition to the routine infant immunizations against S. pneumoniae and Haemophilus influenzae . After undergoing a splenectomy, children should also receive penicillin prophylaxis twice a day for at least several years after surgery. Penicillin V potassium, 250 mg twice daily, is recommended for children older than 3 to 5 years, and a dose of 125 mg twice daily is recommended for younger patients. Some clinicians recommend lifelong penicillin prophylaxis because sepsis can occur years after a splenectomy. Patients and families should be educated to seek prompt medical evaluation for fever because of the risk of bacteremia or sepsis in a person who has undergone a splenectomy. Relatively recent evidence suggests that splenectomy in patients with AIHA may increase the long-term risk of thromboembolism and pulmonary hypertension (also see Chapter 16 ), and these possibilities should be considered and discussed with the patient and family. The timing of a splenectomy is controversial, because other approaches such as rituximab have become available and are relatively safe.
Alternative Immunotherapeutic Agents.
Immunosuppressive and cytotoxic drugs are indicated in patients who do not respond to corticosteroids, rituximab, and splenectomy or for patients who have contraindications to those therapies. Cyclophosphamide, cyclosporine, azathioprine (6-mercaptopurine), and Campath-1H have been used to treat refractory AIHA, but published data on their efficacy are limited to case reports. A response to many of these agents may take months, and thus treatment should be continued for up to 6 months before it is considered to have failed. High-dose cyclophosphamide without stem cell rescue for refractory AIHA has induced complete and partial remissions. Moyo and colleagues treated nine adult patients who had severe refractory AIHA with cyclophosphamide, 50 mg/kg/day for 4 days. Six patients achieved complete remission with normal hemoglobin levels for their age and sex, and three patients had partial remissions, measured as a hemoglobin level of at least 10 gm/dL without transfusion support. Patients became RBC transfusion–independent after a median of 19 days (range, 15-31 days) after treatment. High-dose cyclophosphamide was well tolerated, but common adverse effects included nausea, vomiting, and transient myelosuppression.
Cyclosporine, 6-mercaptopurine, and MMF (mycophenolate mofetil, Cell-Cept) are additional immunosuppressive agents that have induced clinical remission in some patients with refractory AIHA. By affecting T-lymphocyte function, these agents interfere with autoantibody synthesis. Although cyclosporine has improved the course of AIHA in a few patients with steroid-refractory AIHA, it is not routinely used because of the adverse effects of nephrotoxicity, hypertension, and hirsutism. Single-agent therapy with 6-mercaptopurine in pediatric patients with AIHA was reported as part of a case series of autoimmune cytopenias. All seven pediatric patients with refractory AIHA (five cases of primary AIHA and two cases of secondary AIHA) responded, with response defined as an increase in the hemoglobin level of at least 1.5 gm/dL and to a level of 10 gm/dL or greater. The time required to achieve a hematologic response was not reported. The adverse effects of 6-mercaptopurine include leukopenia, thrombocytopenia, and elevated transaminase levels. MMF also inhibits lymphocyte proliferation and was assessed prospectively in a single-center study for adult refractory autoimmune cytopenias. The study included three patients with AIHA that was resistant to high-dose steroids; two of these patients had also responded poorly to IVIG, and one had responded poorly to high-dose cyclophosphamide. All three patients with refractory AIHA responded and achieved a hemoglobin level greater than 10 gm/dL; they were transfusion independent after 4 to 6 months, and the drug was well tolerated. After this response was achieved, MMF was discontinued in one patient and tapered for the other two patients. None had relapsed at a follow-up of 49, 40, and 13 months. Lastly, Campath-1H monoclonal antibodies may improve refractory disease but do not appear to induce a prolonged remission, and the adverse effects include significant immunosuppression.
Danazol.
Danazol, a semisynthetic androgen, has been efficacious for some adults with AIHA, but it has not been studied in children. Synergistic effects of danazol and corticosteroids have been suggested in two studies. A rise in the hematocrit level is usually evident within 1 to 3 weeks. The mechanism of action for danazol is not clear, but decreased titers of cell-bound IgG and complement have been reported in patients who had a response. Because of its potential androgenic adverse effects, it is not typically recommended for young females, and the potential liver toxicity makes its long-term use in young children difficult.
Hematopoietic Stem Cell Transplantation.
Complete lymphoid and myeloablation with hematopoietic stem cell transplantation (HSCT) is a potential option. HSCT has been explored to treat a variety of autoimmune diseases. Its use in patients with AIHA has been reported with limited success. In a study of 36 patients with severe refractory autoimmune cytopenia, two patients underwent allogeneic HSCT and five patients underwent autologous HSCT. The two patients who underwent allogeneic HSCT achieved continuous remission, and of the five patients who underwent autologous HSCT, one died of treatment-related causes, one died of progressive disease, two had no response, and one had a transient response. A single case report describes successful autologous HSCT in a child with refractory AIHA. Despite significant advances in the management of high-dose chemotherapy and HSCT, this treatment option should be reserved for patients with severe life-threatening disease for whom all other therapies have failed.
Treatment Considerations for Cold-Reactive AIHA.
In children, CAD typically occurs after an infection, is associated with M. pneumoniae or viral infections, and usually only requires supportive care with RBC transfusion ( Table 13-3 ). Importantly, the patient should be kept warm and instructed to avoid cold stimuli. Patients may have a mild, compensated anemia that may not require treatment or transfusions. For persons with moderate to severe anemia, transfusion is necessary. In contrast to the serologic problems encountered in persons with warm AIHA, cold IgM-mediated antibodies do not interfere with pretransfusion testing, and it is usually easy to find compatible donor erythrocytes. If transfusion is indicated, a blood warmer must be used to warm the RBCs as they are infused. In severe cases of cold, IgM-mediated AIHA, plasmapheresis can efficiently remove autoantibodies and improve the clinical course. Exchange procedures should also be performed with an in-line blood warmer for infusion of the plasma replacement.
The treatment of PCH is also supportive with transfusion, because the anemia is often moderate to severe at presentation. Although the specificity of the Donath-Lansteiner antibody is directed at the P antigen, P − donor RBCs are rare, and most patients achieve an adequate posttransfusion hematocrit level with P + RBCs. Although cold-induced hemolytic symptoms are not characteristic, the patient should be kept warm and the use of a blood warmer for RBC transfusions is prudent despite the lack of data to support this practice. Steroids are often initiated empirically for treatment of AIHA, but because no evidence exists to support their benefit, they can be discontinued once the diagnosis of PCH is confirmed with the Donath-Landsteiner assay.
Pathogenesis of Autoantibody Formation
The pathogenesis of AIHA is far from clear, and many mechanisms underlying autoantibody production have been proposed. These mechanisms involve autoantigens, the lack of effective presentation of autoantigens, and functional abnormalities of B and T cells. Most evidence suggests that the basis of disease is due to a breakdown in self-tolerance mechanisms toward RBC antigens.
The most common targets of RBC autoantibodies are the Rh blood group proteins, but other blood group antigen specificities, including antibodies directed at glycophorins, band 3, and the RBC anion channel protein have been documented. Cross-reactivity between environmental antigens and autoantigens, termed molecular mimicry , has been proposed as the mechanism to explain such autoantibodies. However, although deletion of the dominant autoantigen in New Zealand Black mice with AIHA demonstrated a defect in self-tolerance directed at the RBC, the autoantibodies were not specific to one RBC antigen. In New Zealand Black mice with AIHA, this T-helper cell cross-reactivity between the helper-dominant epitope and an exogenous antigen illustrates how specific therapy may be needed to induce immune tolerance.
Self versus nonself discrimination is dependent on induction of tolerance in the thymus and bone marrow by deleting, receptor-editing, or anergizing self-reactive T- and B-cell clones. Antigen-presenting cells such as dendritic cells and macrophages present self-antigens to induce T-cell tolerance. One explanation for the lack of effective presentation of autoantigens is that many self-epitopes may be inefficiently processed and presented by antigen-presenting cells. The T cells stay naïve and remain part of the T-cell repertoire rather than being eliminated by the thymus. A genetic predisposition for AIHA has also been proposed involving human leukocyte antigen (HLA) genes that encode the cell surface antigen–presenting proteins. Although no large study has examined the role of HLA genetic polymorphisms and AIHA, an association between the development of AIHA and HLA-B8 and HLA-B27 has been reported.
RBC autoantibodies in patients with AIHA are polyclonal with multiple IgG isotypes and, sometimes, IgA antibodies. Sequence analysis of the immunoglobulin gene rearrangements used by B-cell clones from patients with cold agglutinins suggest that typical in vivo polyclonal antigen-driven selection and mutation occurs. Of note, Stahl and colleagues analyzed the self-reactive IgG and IgM antibody repertoires of patients with warm AIHA using quantitative immunoblotting on a panel of whole-tissue extracts representing sources of self-antigens. They demonstrated that the self-reactive IgG repertoires purified from plasma and from RBC eluates did not differ between healthy donors and patients with warm AIHA, whereas the IgM repertoires from patients showed broadly altered patterns of reactivity compared with those of control subjects. The observation that the IgG autoantibodies of patients with warm AIHA are similar to natural-occurring anti-RBC autoantibodies of healthy donors suggests that dysregulation of IgG autoreactivity may be an underlying mechanism for AIHA.
The role of CD4+ regulatory T cells in AIHA has also been studied, because T regulatory cells have been shown to play a key role in immunologic tolerance and in autoimmune diseases. In patients, autoantibodies and activated autoreactive CD4+ T-helper cells that secrete interferon-γ are specific for Rh proteins on the RBC. It has also been demonstrated that T regulatory cells specific for epitopes on the Rh proteins are present in the peripheral blood and spleens of patients with AIHA and that these cells can inhibit T-helper cells by secretion of the suppressive cytokine interleukin (IL)-10. This role for regulatory T cells has been corroborated in murine models of AIHA in which C57/Bl6 mice depleted of CD4+CD25+ regulatory T cells developed AIHA at a very high incidence after repeated immunization with rat RBCs. Adoptive transfer of purified CD4+CD25+ regulatory T cells but not CD4+CD25− T cells from immunized mice into naïve recipients prevented the induction of AIHA.
The roles of complement activation and cytokine production in the pathophysiology of AIHA have also been investigated. One study has reported that patients with primary or secondary AIHA may have a deficiency of CD59 expression on their RBCs, which may stimulate abnormal complement activation. Patients with AIHA have also been shown to have high serum levels of IL-1α, IL-2, IL-4, IL-2R, IL-6, IL-10, and IL-13. One proposed mechanism is that an imbalance between IL-10 and IL-12 production leads to autoantibody production. Taken together, the mechanism of autoantibody production in persons with AIHA is likely complex and involves polyclonal autoreactive B cells that may be present in healthy persons but expand in genetically susceptible persons or in persons with dysregulation of T regulatory cells or antigen presentation, with subsequent loss of self-tolerance mechanisms.
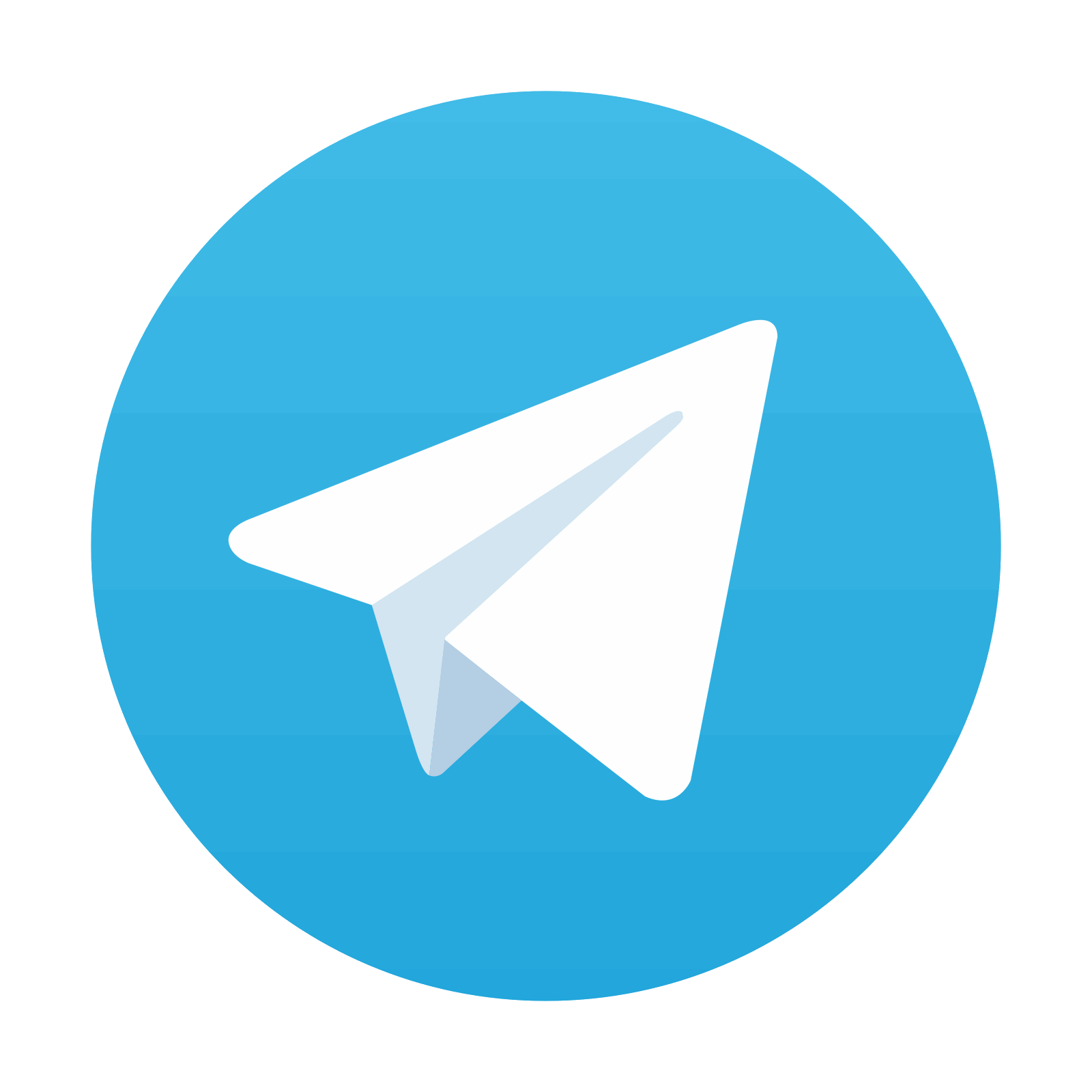
Stay updated, free articles. Join our Telegram channel

Full access? Get Clinical Tree
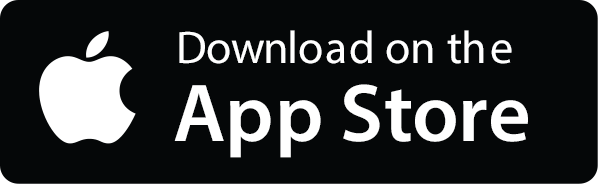
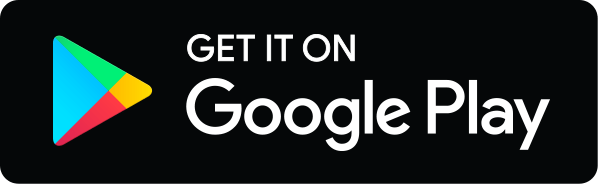