Fig. 12.1
Effect of dietary proteins on metabolism. The digestion, absorption, and metabolism of proteins cause high-energy expenditure in the form of thermogenesis and ATP utilization, either for the synthesis of new proteins or for the degradation of AA via the urea cycle. Proteins also help maintain a high basal metabolic rate by supporting muscle mass while increasing the loss of fat storage under negative energy balance. Finally, the signaling of protein intake through regulatory gut hormones controls food intake and the sensation of satiety. FFA fat free mass
Protein and particularly IAA deficiency is not compatible with survival and protein intake is tightly controlled. Protein consumption in animals and humans appears to be more tightly controlled than the intake of carbohydrates and fats (Griffioen-Roose et al. 2012). Spontaneously, animals select diets having significantly high amount of protein with a proportionate IAA profile and when given a choice among the three macronutrients they often prefer a diet with relatively high-protein content (40 % energy as protein) (Tomé 2004; Gietzen and Rogers 2006). In contrast, animals avoid single IAA-deficient diets (Koehnle et al. 2003), diets with a very low protein (<6 % of energy as protein) to avoid adverse effects from IAA imbalance, although they tend to ingest more of diets that are reasonably below the requirement (5–8 % of energy from protein) compared to regular diets with 10–15 % protein as energy. Higher intake from moderately low-protein diets appears to compensate for the supply of required nitrogen and IAAs and cover protein needs, and food choice is more likely involved in the capacity to reach protein sufficiency (Griffioen-Roose et al. 2014). This could however partly explain the need to consume less of diets with higher protein content as in this case protein control is no more involved in the control of food intake that is only driven by the sensing of energy sufficiency.
12.3 Peripheral Oral and Post-Oral AA Sensing
Foods contain a certain amount of proteins, peptides, and AAs that interact with taste receptors on the tongue. The same GPCRs are found in the alimentary canal (Finger and Kinnamon 2011). The taste of most l-AAs is either sweet (DAAs) or bitter (IAAs), with the exception of glutamic acid and aspartic acid that are sour in the acidic or umami in the ionic form at lower pH (Bassoli et al. 2014; Kawai et al. 2012). Although single AAs are not the most abundant food constituents and vary according to food preparation, they provide complex taste qualities that can modify the flavor of foods. Fermentation, aging, or maturation of foods increases the digestibility of proteins by releasing protein-bound AAs. Soups are also rich in single AAs coming from the extraction of AAs of meats and vegetables (Ninomiya et al. 2010). Humans have an innate attraction for sweet and savory tastes, but flavor preference, which also involves aroma and texture together with taste, is learned as a Pavlovian conditioning response from the post-oral nutritional consequence of food (Sclafani and Ackroff 2012; Teff 2011). A stronger AA taste stimulus may reinforce the satiating feeling of proteins. However, the sensory traits for protein intake regulation are not well understood yet. It has been hypothesized that savory or umami taste, the taste for l-glutamate, acts as a signal for AA ingestion (Griffioen-Roose et al. 2014; Sclafani and Ackroff 2012). The nutritional status (long-term protein intake) may influence the development of specific appetites. A recent study (Griffioen-Roose et al. 2014) found that individuals with a low-protein nutritional status had a higher liking for savory foods than those with a high-protein status. Thus, taste preference and brain reward responses may change with protein status to ensure proper ingestion and restore the body nutritional condition.
Oral and post-oral detection of umami taste involves the GPCR taste receptor type 1 (the heterodimer T1R1/T1R3) (Finger and Kinnamon 2011). l-glutamate is the main agonist for the human T1R1/T1R3, whereas 5′-ribonucleotides such as inosine-5′-monophosphate (IMP) and guanosine-5′-monophosphate (GMP) that enhance umami taste sensitivity function as allosteric modulators (Zhang et al. 2008). This mechanism is known as umami taste synergism. Other receptors also suggested as candidates for umami taste are the splice variants of metabotropic glutamate receptors mGluR4 and mGluR1 (Bachmanov et al. 2014; San Gabriel et al. 2009a) (Table 12.1). Additional GPCRs that are part of the AA signaling pathway are the extracellular calcium-sensing receptor (CaSR), the GPCR family C subtype 6A (GPRC6A), and the G-protein-coupled receptor 92 (GPR92) (Depoortere 2014; Haid et al. 2013; San Gabriel et al. 2009b). GPR92 binds to protein hydrolysates (peptone) and it is suggested to participate in umami taste perception as well (Haid et al. 2013).
Table 12.1
Distribution of AA taste receptors in the oral cavity and the stomach with their predominant functions
Tissue | Cell type | AA receptor | Stimulant | Function |
---|---|---|---|---|
GPCRs | ||||
Taste buds | ||||
Oral cavity | TRCs | T1R1/T1R3 | l-Glutamate | Umami |
l-Aspartate | ||||
IMP, GMP | ||||
CaSR | Glutathione | Taste modifier | ||
Gamma-glutamyl | ||||
peptides | ||||
GPRC6A | l-Alanine | Unknown | ||
l-Arginine, Glycine | ||||
l-Lysine, l-Serine | ||||
l-Methionine | ||||
T1R2/T1R3 | Glycine | Sweet | ||
T2Rs | l-Tryptophan | Bitter | ||
l-Phenylalanine | ||||
mGluRs | l-Glutamate | Umami | ||
GPR92 | Peptides | Umami (?) | ||
Stomach | Parietal | CaSR | l-histidine | ↑Acid |
l-Phenylalanine | Secretion | |||
l-Tryptophan | Pepsinogen activation | |||
mGluRs | l-Glutamate | |||
Chief cells | mGluR1 | l-Glutamate | ↑ Pepsinogen protein digestion | |
EC | ? (indirectly) | l-Glutamate | 5-HT release | |
G cells | CasR | As parietal cells | ↑Gastrin | |
GPR6A | As in taste | |||
GPR92 | Peptone | |||
P/D1 cells | T1R3 | l-Glutamate (?) | ↓Ghrelin | |
D cells | CaSR | As parietal cells | Somatostatin release | |
GPRC6A | As taste cells | |||
mGluRs | l-Glutamate |
In the stomach where protein digestion starts, single AAs can regulate gastric secretion by modulating gastrin and somatostatin release (Haid et al. 2011). l-glutamate also potentiates gastric secretion via the vagus nerve after binding to glutamate receptors (T1R1/T1R3 and mGluRs) located in gastric glands (Khropycheva et al. 2011; Nakamura et al. 2011; San Gabriel et al. 2007; Uneyama et al. 2006). As a result, single AAs from the diet not only add flavor but may also increase protein digestion efficiency. Proteins are also able to suppress the release of ghrelin, the only GI hormone known to increase appetite (Foster-Schubert et al. 2008). Ghrelin-producing cells from murine stomach have no contact with the liminal content, but show T1R3 immunoreactivity (Hass et al. 2010). Circulatory AAs may suppress ghrelin release through T1R3 after absorption. To our knowledge, this has not been confirmed in humans.
These AA and oligopeptide receptors of the alimentary canal initiate the signals for the satiation mechanism.
12.4 Intestinal AA Sensing Signals Satiety in the Brain
The sensing of AAs in the intestine takes place in enteroendocrine cells (Fig. 12.2). The binding of single AAs or oligopeptides with their corresponding receptor triggers the release of regulatory gut hormones such as gastrin, somatostatin, serotonin (5-hydroxytryptamine, 5-HT) (enterochromaffin cells, EC), and ghrelin from stomach (Table 12.1), cholecystokinin (CCK) from duodenum (I-cells), and glucagon-like peptide 1 (GLP-1) and peptide tyrosine-tyrosine (PYY) from ileum and colon (L-cells) (Moran and Dailey 2011) (Table 12.2). These regulatory peptides act mainly locally on receptors at the end of nearby vagal afferents where they are found in higher concentration, but gut peptides can also function in an endocrine way through systemic circulation. Proteins are particularly effective in inducing CCK release and GLP-1 in humans. Protein hydrolysates appear to stimulate enteroendocrine function more potently than single AAs (Rasoamanana et al. 2012). Moreover, the umami seasoning monosodium l-glutamate (MSG) may be involved in the release of GLP-2 (Bauchart-Thevret et al. 2013; Wang et al. 2011). It is proposed that MSG enhances protein satiety acting as a signal for the satiating properties of protein (Masic and Yeomans 2014a, b; Ventura et al. 2012). In a protein-rich context MSG may enhance the effects of proteins.
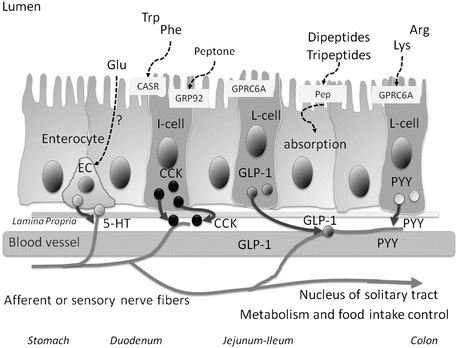
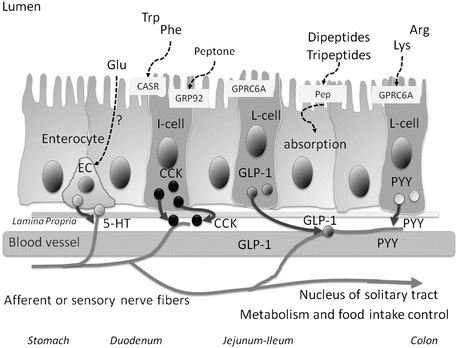
Fig. 12.2
Graphic overview of a section of the intestine with the expression of receptors that can sense the presence of AAs and oligopeptides in the luminal content. I- and L-cells represent the various types of endocrine cells that respond to luminal AAs with the release of gut hormones. EC Enterochromaffin cells, Pep peptide transporter, CaSR calcium-sensing receptor, GPRC6A G-protein-coupled receptor family C group 6 member A, CCK cholecystokinin, GLP1 glucagon-like peptide-1, PYY peptide YY
Table 12.2
Distribution of AA taste receptors in the intestine and their digestive functions
Intestine | Cell type | AA receptor | Stimulant | Function |
---|---|---|---|---|
GPCRs | ||||
Duodenum | I cell | CaSR | Aromatic AAs | CCK release (satiation motility) |
T1R1/T1R3 | l-Glutamate | |||
IMP, GMP | ||||
GPR92 | Peptone | |||
Jejunum Ileum Colon | L cell | GRPC6A | Basic AAs | GLP-1 |
Neutral AAs | GLP-2 | |||
PYY (Satiation motility) | ||||
Jejunum Ileum | K cell | Unknown | AAs | GIP |
