(1)
Institut Bergonié, Bordeaux, France
Abstract
Apoptosis is one of the main modalities of cell death, so important that it has outshined all others that only begin to be rediscovered. Apoptosis is an active, programmed way of cell death, implemented in response to intracellular or extracellular signals. Intracellular signals can originate from DNA lesions, mitosis defects, oxidative stress or other stresses, while extracellular signals correspond to death messages emitted by other cells. Apoptosis leads to the activation of proteases, called caspases, able to hydrolyse the cell constituents independently from the proteasome. Apoptosis plays many fundamental roles during embryology and tissue homoeostasis; to provide only one example, it is responsible for thymus regression during the passage from childhood to adulthood. Many authors have considered apoptosis as the obligate pathway to cell death induced by anticancer drugs, but in many cases it is only a phenotypic consequence, secondary to other mechanisms of drug-induced cell death, which only indirectly involve apoptosis. Apoptosis is often opposed to necrosis, but comparisons can also be made with senescence and mitotic cell death.
We present in this chapter the signalling pathways which lead to the execution of the cell death programme, but we neither describe the techniques that allow to detect or quantify apoptosis nor the execution of the apoptosis programme. In the first part, we present the most important proteins involved in apoptosis, from effectors to initiators. We present then the intracellular messages and the ‘intrinsic’ pathway that drive cells to death after they have undergone diverse types of stress and the extracellular signals that activate the ‘extrinsic’ pathway and drive cells to death on command. Various oncogenic alterations in apoptosis pathways have been identified, which lead to the identification of pharmacological targets.
18.1 Proteins Involved in Apoptosis
18.1.1 Caspases
Caspases are the effector enzymes of apoptosis; these are proteases characterised by the presence of a cysteine residue in their active centre and by the capacity of hydrolysing polypeptide chains at the level of an aspartic acid residue, which explains the name of caspase. Some caspases (class I) are able to activate signalling molecules through proteolysis, such as interleukin 1 (IL1) (Chap. 12). Other caspases (class II) are the apoptosis effector caspases and can hydrolyse and destroy a large variety of cell proteins; caspase 3 (CASP3) is the main class II caspase. Finally, class III caspases, such as caspase 8 and caspase 9, are apoptosis initiator caspases, which activate the class II caspases by proteolysis.
Protein activation by mild proteolysis on definite sites is one of the most important mechanisms of post-translational modulation of protein activity (Annex C). Cell proteins should normally be protected from proteolytic enzymes; most of them exist therefore under inactive forms (procaspases in the case of caspases), which themselves are activated by mild proteolysis. Of course, this requires an initiating event in order to carry out the first proteolytic activity of the cascade. In the case of apoptosis, this event consists in the formation of transient supramolecular edifices, sometimes called platforms, which arrange the procaspases, with the help of adapter proteins, so that they will become able to mutually hydrolyse themselves and thus become activated.
As a general mechanism, the activation of effector caspases requires initiator procaspases, adapter proteins enabling them to organise a platform and the effector procaspases awaiting proteolytic activation. In the case of the intrinsic pathway, the initiator procaspase is procaspase 9, and the activation platform is called the apoptosome; in the case of extracellular signal-triggered apoptosis, the initiator procaspase is procaspase 8, and the activation platform is called the DISC (death-inducing signalling complex). The activation of a procaspase into a caspase involves the elimination of an N-terminal prodomain, the break of a C-terminal domain that remains non-covalently attached to the rest of the molecule and the dimerisation of two rearranged caspase monomers (Fig. 18.1). Initiator and effector procaspases differ by the structure of their N– and C-terminal domains; initiator procaspases harbour in their N-terminal part specific recognition domains, which are called CARDs (caspase recruitment domains) or DEDs (death effector domains) as appropriate. All caspases have kept the same amino acid sequence in their active centre (QACXG) and the capacity, one activated and dimerised, to split polypeptide chains immediately ahead an aspartic acid residue. Figure 18.2 presents the two steps of the activation of an initiator procaspase into a caspase and of an effector procaspase into a caspase as well as the ultimate intervention of an activated effector caspase on a target protein.
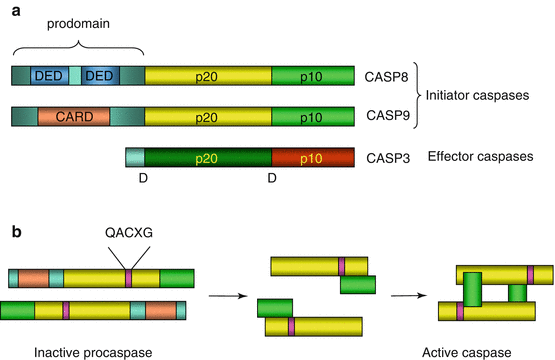
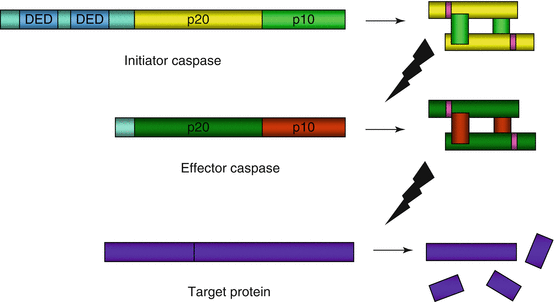
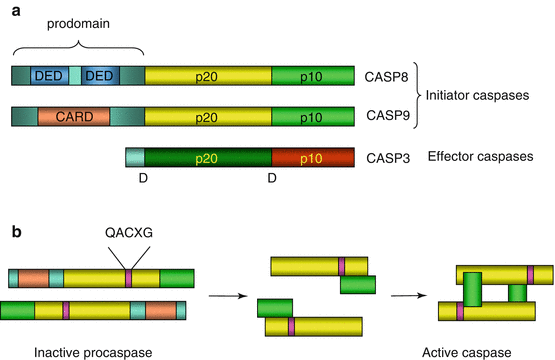
Fig. 18.1
Caspase structure and activation. (a) General structure of caspases. All procaspases contain two domains, p20 and p10, the cleavage of which, at the level of an aspartic acid residue D, allows the transition to an active conformation. They also contain a prodomain whose elimination is required for catalytic activity. Initiator procaspases have an especially long prodomain, which bears DED (caspase 8 and caspase 10) or CARD (caspase 9) interaction domains. (b) Caspase activation. The example represented here is that of caspase 9. The catalytic site responsible for cleavage is characterised by the QACXG sequence. The arrangement of procaspases in apoptosome or DISC enables the proteolytic cleavage of one molecule by another one at the level of the aspartic acid residue. After prodomain elimination, the rearrangement of p10 and p20 domains and the dimerisation of the protein activate the catalytic function
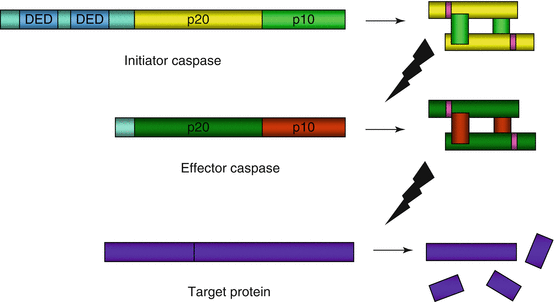
Fig. 18.2
Caspase proteolytic cascade. An initiator caspase (here caspase 8, with two DED domains for the recognition of the adapter proteins FADD and TRADD), which is itself autocatalytically activated, activates in turn an effector procaspase at the level of two aspartic acid residues. The cleavage products, after dimerisation, constitute the active caspase that can hydrolyse in turn various cell proteins, always at the level of an aspartic acid residue
18.1.2 BCL2 Family Proteins
BCL2 (B–cell lymphoma 2) proteins constitute a family of 22 proteins, which are either mitochondrial or able to associate to mitochondria during their activation process. They present sequence homologies explaining why they belong to the same family, but their functions may be quite different from one to another and it is necessary to divide this family into subfamilies. They are characterised by the presence of specific domains of different types called BH (BCL2 homology) domains. Some of them comprise four BH domains (BH1 to BH4) and a transmembrane domain enabling their insertion in the mitochondrial outer membrane. BH1 and BH2 domains are involved in the regulation of the permeabilisation of this membrane; the BH3 domain, in association with BH1 and BH2 domains, is a protein–protein interaction domain allowing their homo- or heterodimerisation; the BH4 domain, essentially intracytoplasmic, is an interaction domain for the adapter protein, APAF1 (apoptotic peptidase-activating factor 1). The BCL2 protein subfamilies are the following ones (Fig. 18.3):
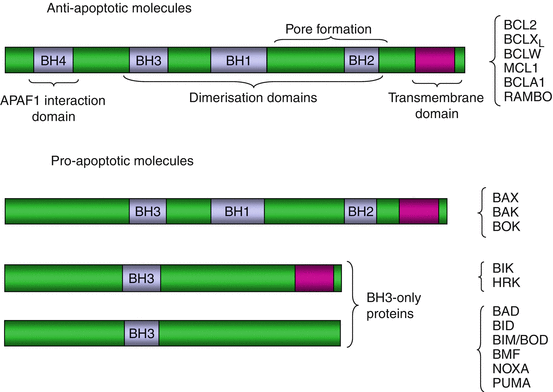
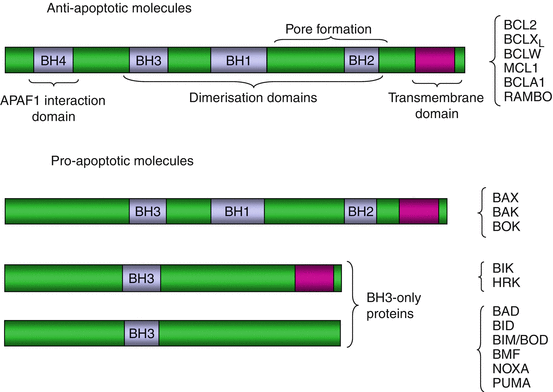
Fig. 18.3
BCL2 family proteins. These proteins can contain a transmembrane domain and up to four special interaction domains, involved in dimerisation (BH1, BH2 and BH3 domains), in the opening of the mitochondrial permeability transition pore (BH1 and BH2 domains) and in the interaction with APAF1 (BH4 domain). The BCL2-type antiapoptotic proteins are inserted in the mitochondrial outer membrane thanks to their N-terminal portion bearing the transmembrane domain and mobilise APAF1 in the cytosol through their C-terminal portion. The BAX-type proapoptotic molecules also have a transmembrane domain, dimerisation domains and protein–protein interaction domains. The BH3-only proteins have generally no transmembrane domain and are not inserted in the mitochondrial membrane but display mitochondrial tropism due to their BH3 domain, which can interact with the corresponding domains of the other BCL2 family proteins
Proteins containing all four BH domains and a transmembrane domain: BLC2; BCLXL/S (gene BCL2L1), which are splicing variants with opposite functions; BCLW (gene BCL2L2); MCL1 (myeloid cell leukaemia sequence 1) or BCL2L3; BCL2A1 or BCL2L5; BCL-RAMBO (gene BCL2L13); BNIP3 (BCL2/adenovirus-interacting protein 3); and DIVA (death -inducing vBCL2/Apaf1-binding protein, gene BCL2L10); these proteins are antiapoptotic, except the BCLXS variant.
Proteins containing three BH domains (the BH4 domain is lacking): BAX (BCL2–associated X protein) or BCL2L4, BAK (BCL2 homologous antagonist/killer) or BCL2L7 (gene BAK1), BCL2L12, BCL2L14 and BOK (BCL2–related ovarian killer) or BCL2L9; these proteins are proapoptotic.
Proteins containing only a BH3 domain (BH3–only proteins), which are proapoptotic; some of them also contain a transmembrane domain: BIK (BCL2-interacting killer) and HRK (Harakiri BCL2–interacting protein), while others do not: BAD (BCL2–associated agonist of cell death) or BCL2L8; BID (BH3 domain-interacting death agonist); BIM (BCL2–interacting mediator of cell death), also known as BOD (BCL2–related ovarian death agonist) (gene BCL2L11); BMF (BCL2-modifying factor); BFK (BCL2 family kin, gene BCL2L15); NOXA (gene PMAIP1, phorbol myristate acetate-induced protein 1); and PUMA (p53–upregulated mediator of apoptosis) (gene BBC3, BCL2-binding component).
We describe the mechanisms by which these proteins are engaged in the control of apoptosis triggering in the part devoted to the intrinsic apoptosis pathway.
18.1.3 IAP Family Proteins
IAPs (inhibitor of apoptosis proteins) or BIRCs (baculovirus IAP repeat-containing proteins) constitute a group of eight cytoplasmic caspase inhibitors. These proteins harbour a series of characteristic domains (Fig. 18.4). Some are constant, the BIR1, BIR2 and BIR3 domains, and allow protein–protein interactions, especially with caspase 3. Others are inconstant, the CARD (caspase recruitment domain) domains, which allow their interaction with initiator caspases or adapter proteins, and the RING (really interesting new gene) domains, which display an E3 ubiquitin ligase activity toward caspases, driving them to the proteasome and adding caspase destruction to caspase inhibition. In addition to their role in apoptosis inhibition, these proteins play an important role in cytodieresis, at the end of mitosis (Chap. 17), and in the regulation of NFκB pathway (Chap. 12).
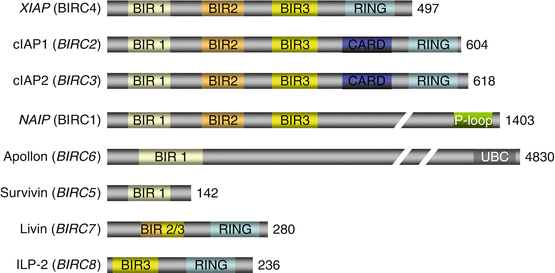
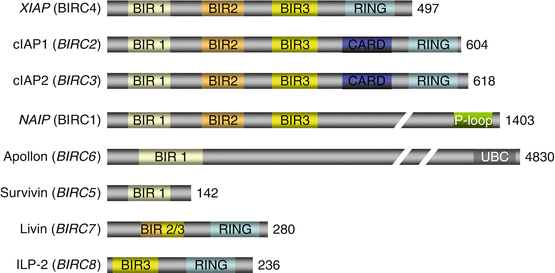
Fig. 18.4
IAP family proteins. These eight proteins with caspase inhibitory function are cytosolic. They harbour various interaction domains, especially a CARD domain enabling their interaction with caspases, several BIR domains, a RING domain for E3 ubiquitin ligase activity or a UBC domain for E2 ubiquitin-conjugating activity. Gene names are in italics. The number of constitutive amino acids in indicated on the right
The proteins equipped with a CARD domain, and therefore able to interact with CASP8, are cIAP1 and cIAP2 (cellular inhibitor of apoptosis protein) (genes BIRC2 and BIRC3, respectively). Together with XIAP (chromosome X inhibitor of apoptosis protein), alias BIRC4 or ILP1, they also have a RING domain, indicating their function of E3 ubiquitin ligase toward caspases. Apollon or bruce (gene BIRC6) contains in contrast a ubiquitin-conjugating (UBC) domain and functions as an E2 ubiquitin-conjugating enzyme. NAIP (NLR family apoptosis inhibitory protein), alias BIRC1, seems to be confined to neural cells, preventing them from apoptosis. Survivin (gene BIRC5), livin (gene BIRC7) and ILP2 (IAP–like protein 2) (gene BIRC8) are involved in cancer cell apoptosis; livin and ILP2 harbour a RING domain.
Most of these proteins are themselves inhibited by a mitochondrial protein, SMAC (second mitochondria–derived activator of caspase) (gene DIABLO, direct inhibitor of apoptosis–binding protein with low pI). This protein acts as a dimer and binds the BIR3 domains of the IAP, so that their binding to caspases is inhibited.
18.1.4 Death Receptors and Their Ligands
Death signals are brought by proteins that are members of the TNF superfamily (TNFSF). TNFSF members that are type II transmembrane proteins (intracellular N-terminal extremity) can be cleaved and generate diffusible ligands. They recognise, on the plasma membrane of the target cell, cognate receptors of the TNF receptor superfamily (TNFRSF). There exists a total of about 17 TNFSF members and 26 TNFRSF members; only those involved in cell death induction are studied here. Three TNFSF members are involved in apoptosis signalling: FASL (FAS ligand) or TNFSF6 (gene FASLG), TNF (tumour necrosis factor) or TNFSF2 and TRAIL (TNF–related apoptosis–inducing ligand) (gene TNFSF10).
The TNFRSF members that recognise these death signals are called death receptors. These are type I transmembrane proteins that harbour an extracellular ligand-binding domain, a single transmembrane domain and an intracellular signalling domain (Fig. 18.5). Death receptors contain a death domain (DD), which enables their interaction with an adapter protein harbouring a homologous domain; there are four main death receptors: FAS (fragment for apoptosis stimulation), also known as CD95 or TNFRSF6; TRAILR1 or DR4 (gene TNFRSF10A); TRAILR2 or DR5 (gene TNFRSF10B); and TNFR1 or DR1 (gene TNFRSF1A). These receptors recognise the extracellular ligands FASL, TRAIL and TNF, respectively. FAS, TRAILR1 and TRAILR2 bind to the same intracellular adapter protein, FADD (FAS–associated death domain protein), whereas TNFR1 binds to the adapter protein TRADD (TNF receptor-associated death domain protein). Both adapter proteins can activate an initiator protein, caspase 8.
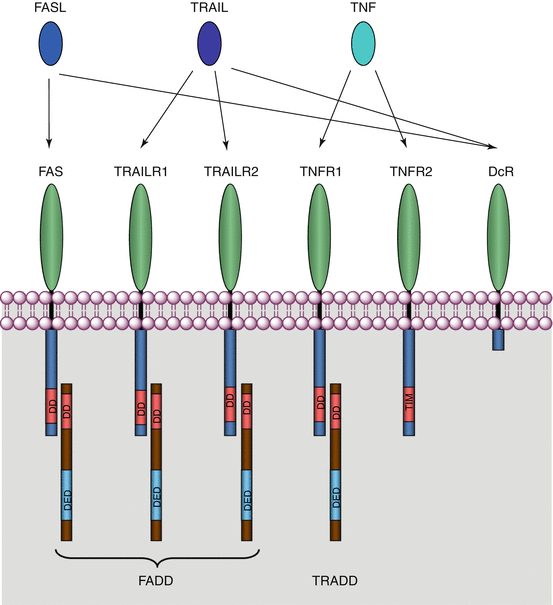
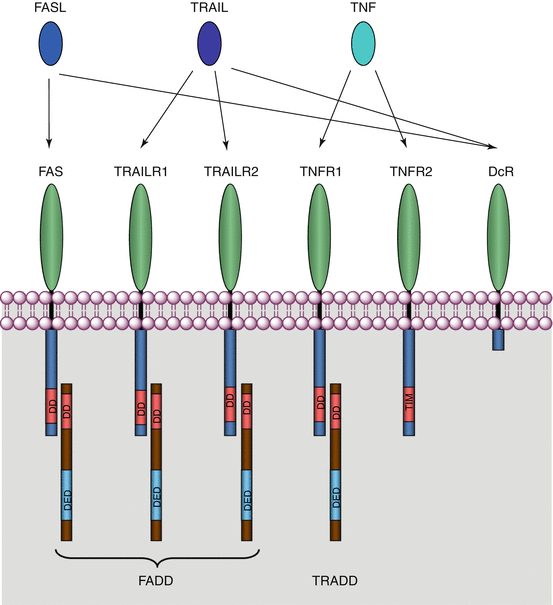
Fig. 18.5
Cell death receptors, ligands and adapter proteins. The ligands of the TNF superfamily (FASL, TNF and TRAIL) recognise and bind receptors of the TNFR superfamily (FAS, TNFR1, TRAILR1 and TRAILR2) as well as decoy receptors (DcR) devoid of binding domains with an adapter protein. Many other ligands members of the TNF superfamily can bind cognate receptors of the TNFR superfamily outside the field of apoptosis
In addition, some receptors harbour no intracellular binding domain and transduce no message. They have either a very short intracellular domain or are simply attached to the membrane by a GPI anchor. These are decoy receptors, DcR1 or TRAILR3 (gene TNFRSF10C), DcR2 or TRAILR4 (gene TNFRSF10D) and DcR3 (gene TNFRSF6B), which are able to block the signals brought by TRAIL (for DcR1 and DcR2) or FAS (for DcR3) and divert the information received.
We describe the mechanisms by which these proteins are engaged in the control of apoptosis triggering in the part devoted to the extrinsic apoptosis pathway. A variety of other proteins also harbour interaction domains with the adapter proteins FADD and TRADD; some are found in the caspase family and in the IAP family and have been already mentioned; others will be described with the extrinsic apoptosis pathway.
18.2 Intrinsic (Mitochondrial) Apoptosis Pathway
18.2.1 Activation of the Intrinsic Pathway
The activation of the intrinsic apoptosis pathway depends upon the emission of signals that are sent to the mitochondria; the mitochondria integrate these signals and elaborate a response which leads to the activation of an initiator caspase, caspase 9, from which ensues the activation of the effector caspases, caspase 3 and caspase 7 (Fig. 18.6). The signals that reach the mitochondria consist of a variety of proteins of the BCL2 family called the BH3–only proteins, which are produced in response to various stresses by several types of mechanisms. Some BH3-only proteins are simply regulated by transcriptional control, and others undergo post-translational modifications that govern their activity. DNA damage, which enables p53 activation (Chap. 17), leads in particular to the transcription of the genes encoding PUMA and NOXA. Similarly, microtubule damage leads to the transcription of the genes encoding BIM and HRK. In contrast, BAD deactivation is induced by AKT-mediated phosphorylation (Chap. 3) in response to growth factor signalling, and BID is activated by proteolysis carried out by caspase 8, the initiator caspase of the extrinsic pathway.
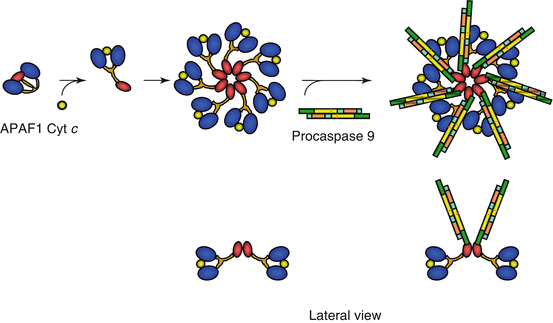
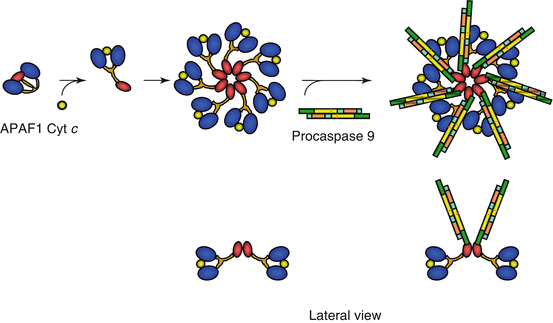
Fig. 18.6
Formation and activation of the apoptosome. APAF1, which is folded at the basal state, is able, in the presence of cytochrome c, to undergo a conformation change and form a heptameric structure, apoptosome, to which are associated procaspase 9 molecules. The APAF1 CARD domains can interact with the corresponding domains of caspase 9, allowing autocatalytic activation of caspase 9
BH3-only proteins are able, thanks to their BH3 domain, to interact at the level of the mitochondria with other BCL2 family proteins equipped with transmembrane domains allowing their mitochondrial membrane insertion. These interactions between BCL2 proteins control the permeabilisation of the mitochondrial outer membrane; the proapoptotic proteins BAX and BAK are able to form oligomers constituting transmembrane channels or inducing its permeabilisation through lipid–protein interactions. The exact mechanisms used by antiapoptotic (BCL2 type) and proapoptotic proteins (BAX type) to regulate the permeability of the mitochondrial outer membrane are not fully understood and remain still controversial.
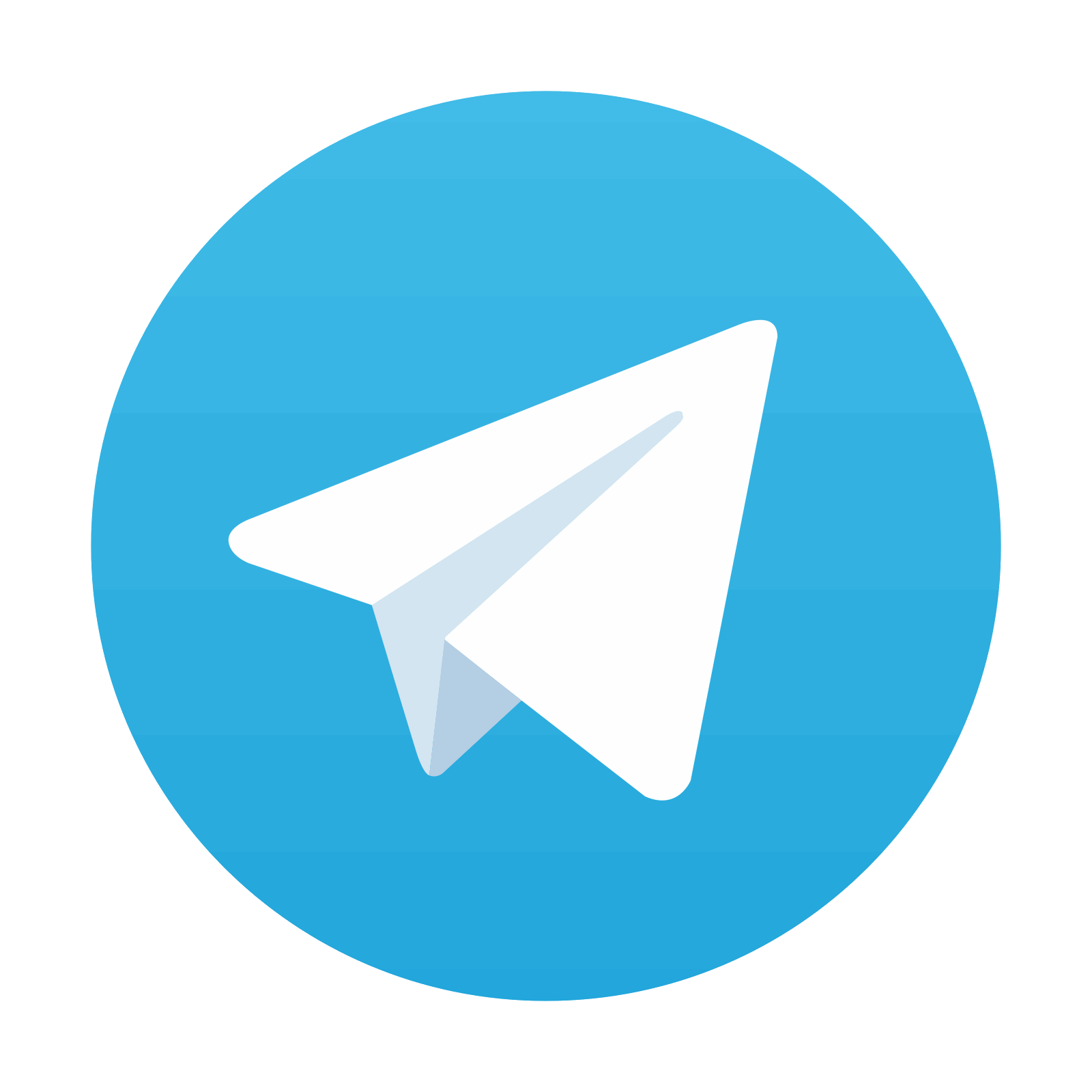
Stay updated, free articles. Join our Telegram channel

Full access? Get Clinical Tree
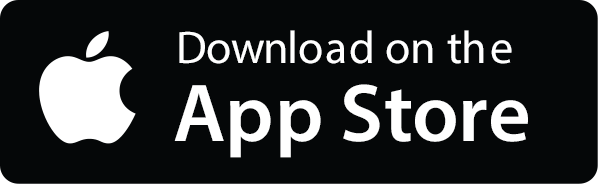
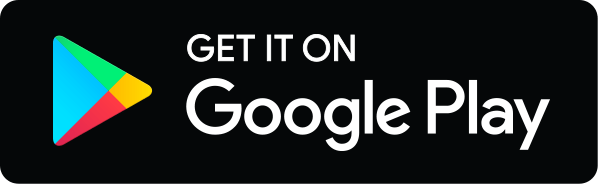