Fig. 15.1
Targets of systemic antifungal agents
Clinical outcomes in susceptible isolates are best predicted by the peak serum level to MIC ratio. Serum drug levels are infrequently measured and are typically available only in the research setting [6]. Resistance to AMB is common in Aspergillus terreus, Pseudallescheria boydii complex, Scedosporium prolificans, Trichosporon spp., and Candida lusitaniae (Table 15.1). Resistance has been reported in other species and changes in ergosterol biosynthesis and/or the synthesis of alternative sterols is the proposed mechanism for reduced AMB activity in these isolates [7].
Table 15.1
Antifungal spectrum of activity against common molds and yeast
Organism | AMB | FLU | ITR | POS | VOR | ANI | MFG | CAS | 5FC |
---|---|---|---|---|---|---|---|---|---|
Aspergillus fumigatus | + | − | + | + | + | + | + | + | − |
Aspergillus flavus | +/− | − | + | + | + | + | + | + | − |
Aspergillus terreus | − | − | + | + | + | + | + | + | − |
Aspergillus niger | + | − | +/− | + | + | + | + | + | − |
Candida albicans | + | + | + | + | + | + | + | + | + |
Candida glabrata | + | +/− | +/− | + | + | + | + | + | + |
Candida krusei | + | − | +/− | + | + | + | + | + | +/− |
Candida parapsilosis | + | + | + | + | + | +/− | +/− | +/− | + |
Cryptococcus spp. | + | + | + | + | + | − | − | − | + |
Blastomyces spp. | + | + | + | + | + | +/− | +/− | +/− | − |
Histoplasma spp. | + | +/− | + | + | + | +/− | +/− | +/− | − |
Coccidioides spp. | + | + | + | + | + | − | − | − | − |
Fusarium spp. | +/− | − | − | + | + | − | − | − | − |
Phaeohyphomycosesa | + | − | + | + | + | + | + | + | − |
Scedosporium apiospermum | +/− | − | +/− | + | + | − | − | − | − |
Scedosporium prolificans | − | − | − | +/− | +/− | − | − | − | − |
Mucorales b | +/− | − | − | + | − | − | − | − | − |
AMB is available in inhalational, oral, and intravenous formulations. The well-known nephrotoxicity seen with intravenous amphotericin B deoxycholate (AmBd; Fungizone) has prompted the development of lipid formulations with more favorable side-effect profiles so that in patients with hematological malignancies lipid formulations of AMB have largely replaced the use of AmBd. Liposomal amphotericin B (L-AMB; Ambisome), amphotericin B lipid complex (ABLC; Abelcet), and amphotericin B colloidal dispersion (ABCD; Amphotec, Amphocil) are all currently available lipid formulations with a high degree of protein binding (>95 %, primarily to albumin) and comparably long half-lives.
Cerebrospinal fluid levels are low (<5 % of concurrent serum concentration) yet AMB remains the drug of choice for cryptococcal meningitis [8]. Intrathecal AMB has been used in the salvage setting for both coccidioidal and candidal meningitis; however, this practice is seldom used due to poor patient tolerability, the difficulty of administration, and the availability of alternative agents.
Similarly, AMB has low vitreous penetration (0–38 %) and intraocular injections are often required in the treatment of deep-seated ophthalmologic fungal infections including candidal endophthalmitis [9, 10]. The route of elimination of AMB remains unknown and dosing need not be adjusted in patients with a reduced glomerular filtration rate.
The broad antifungal spectrum and experience with the use of amphotericin B accounts for its continued use despite toxicity concerns. Liposomal amphotericin B remains a recommended antifungal during the treatment of neutropenic fever following an open-label, randomized international trial comparing L-AMB to voriconazole [11]. A recent meta-analysis also suggests L-AMB is associated with lower mortality during empiric treatment of neutropenic fever [12].
AMB was previously the preferred first-line agent during the treatment of invasive aspergillosis; however, a comparative trial has now demonstrated a lower mortality rate when voriconazole is administered in this setting [13, 14]. AMB remains the agent of choice for treatment of mucormycosis and a delay in the prescribing of an AMB formulation in this population is associated with a twofold greater risk of death [15].
AMB in combination with flucytosine remains the drug of choice in the treatment of cryptococcal meningitis and in most cases a lipid formulation is preferred due to the decreased incidence of nephrotoxicity. Severe infection caused by one of the endemic mycoses (histoplasmosis, coccidioidomycosis, blastomycosis, and sporotrichosis) should also be treated with an AMB formulation.
Intravenous AmBd is typically given in doses of 0.7–1 mg/kg, while the lipid formulations are given in higher doses (3–5 mg/kg). Recently, in an attempt to define the efficacy of even higher doses of lipid formulations, doses of L-AmB (3 mg/kg vs 10 mg/kg) were given in a randomized trial of invasive mold infections. Success rates were similar in both arms although more nephrotoxicity was seen in those receiving higher L-AmB doses [16]. Despite these findings, in cases of severe or life-threatening disease, escalating doses of lipid AMB formulations may be indicated.
Local delivery of amphotericin B has been attempted through use of aerosolized therapy. Lipid preparations are preferred for inhalation and aerosolized delivery has been found effective in the prevention of pulmonary IFIs in lung transplantation and in bone marrow transplant recipients [17, 18].
Side effects of AmBd infusion include fever, chills, rigors, myalgias, bronchospasm, nausea and vomiting, tachycardia, tachypnea, and hypertension [19]. The use of lipid formulations has decreased the incidence of these untoward effects although ABCD has been associated with the development of dyspnea and hypoxia and L-AMB has been associated with back pain during infusion [9]. The potential nephrotoxicity of amphotericin B is well known and may occur in up to 30 % of patients. Vascular smooth muscle dysfunction with resultant vasoconstriction and ischemia are the presumed mechanism of renal injury following AMB administration [20]. Lipid preparations have lower rates of nephrotoxicity and studies have shown that when AmBd is replaced by a lipid formulation after the development of acute kidney injury, renal function stabilizes or improves in a significant proportion of patients [21]. Despite the higher drug costs of lipid AMB formulations, the reduction in hospital days when toxicity is avoided has proven the lipid formulations more cost-effective than AmBd [21].
15.3 Triazoles
The triazoles exert their effects within ergosterol synthesis by inhibition of cytochrome P450 (CYP)-dependent 14-α-demethylase preventing the conversion of lanosterol to ergosterol (Fig. 15.1). This class has demonstrated both species- and strain-dependent fungistatic or fungicidal activity in vitro and the area under the curve to MIC ratio (AUC/MIC) is the best predictor of drug efficacy.
Azoles differ in their affinity for the 14-α-demethylase enzyme and these differences are largely responsible for their varying antifungal potency and spectrum of activity. Cross-inhibition of several human CYP-dependent enzymes (3A4, 2C9, and 2C19) is responsible for the majority of the clinical side effects and drug-drug interactions within this class. Itraconazole and posaconazole act as inhibitors of 3A4 and 2C9 with little effect on 2C19 and voriconazole acts as both an inhibitor and a substrate on all three isoenzymes. Although comprehensive lists of triazole drug interactions can be found elsewhere, concurrent administration should be avoided with most HMG-CoA reductase inhibitors, benzodiazepines, phenytoin, carbamazepine, cyclosporine, tacrolimus, sirolimus, methylprednisolone, buspirone, alfentanil; the dihydropyridine calcium channel blockers; the sulfonylureas, rifampin, rifabutin, vincristine, busulfan, docetaxel, trimetrexate; and the protease inhibitors ritonavir, indinavir, and saquinavir [22–28].
The triazoles have also been associated with QTc prolongation and coadministration with other agents known to have similar effects should be avoided. This class is additionally embryotoxic and teratogenic and is secreted into breast milk, so that administration should be avoided during pregnancy or while lactating [29–31].
15.3.1 Fluconazole
Fluconazole (Diflucan) remains one of the most prescribed triazoles due to its favorable bioavailability, tolerability, and side-effect profile. Greater than 80 % of ingested drug is found in the circulation, and excellent urine and tissue penetration (CSF levels 70 % of matched serum levels) are seen. A half-life of 27–34 h allows once daily dosing for most patients; however, in those with a reduced creatinine clearance, the normal dose should be reduced by 50 %.
Fluconazole is active against most Candida spp. with the exception of C. krusei and C. glabrata (Table 15.1). Susceptible C. glabrata isolates should be treated with higher doses (12 mg/kg/daily) of fluconazole than other species [10].
Fluconazole remains one of the first-line agents in the treatment of oropharyngeal candidiasis (OPC) [10] and patients with frequent relapse should remain on chronic suppressive fluconazole until immune reconstitution has been documented as chronic therapy is well tolerated when necessary [32].
Fluconazole has also been used for antifungal prophylaxis in high-risk patients. Initiation of 400 mg/day of fluconazole for the first 75 days following bone marrow transplantation (BMT) has been found effective in reducing cases of candidemia [33].
Following induction therapy with AMB and flucytosine, fluconazole is used for suppression of cryptococcosis following CNS disease until immunologic derangements have abated – otherwise life-long therapy is recommended [8]. Recent data has examined the use of high-dose fluconazole monotherapy during induction treatment of cryptococcal meningitis; however, this practice should be used only in resource limited settings and not when AMB is available [34].
Headache, alopecia, and anorexia are the most common side effects (10 %) with transaminase elevation in <10 %.
15.3.2 Itraconazole
Itraconazole is currently available as both capsules and an oral solution suspended in hydroxypropyl-β-cyclodextrin (HPCD). The intravenous preparation is no longer available in all developed countries. Itraconazole capsules are dependent upon an acidic environment for maximal absorption, and the concomitant administration of H2-receptor antagonists or proton pump inhibitors causes unpredictable drug absorption, and it is recommended that itraconazole capsules be taken with food or an acidic beverage [35, 36].
Itraconazole solution allows for greater oral bioavailability and the AUC and peak concentrations are both increased by 30 % when itraconazole solution is taken in the fasting state [37]. Oral loading (200 mg three times daily for 3 days) allows for more rapid attainment of therapeutic serum levels. Itraconazole is also highly protein bound with less than 1 % available as free drug and has a relatively high volume of distribution.
The recent development of more effective antifungal agents (i.e., voriconazole) has relegated itraconazole to second-line therapy during the treatment of invasive aspergillosis. Itraconazole does, however, remain the drug of choice for those with mild to moderate infection caused by histoplasmosis [38]. Itraconazole is also approved for allergic bronchopulmonary aspergillosis (ABPA) [14].
The recommended dosage of oral itraconazole in adults is 400 mg/day (capsules) and 2.5 mg/kg twice daily (HPCD solution) [14]. Dose adjustment is not indicated with use of the oral formulations of itraconazole when used in patients with renal insufficiency or those receiving hemodialysis. The half-life of itraconazole is prolonged in patients with hepatic dysfunction and dose adjustment, liver function testing, and drug interactions should be carefully assessed in this circumstance [39].
The most frequent side effects include nausea and vomiting (<10 %), hypertriglyceridemia (9 %), hypokalemia (6 %), liver enzyme elevations (5 %), skin rashes/pruritus (2 %), headache and dizziness (<2 %), and pedal edema (1 %) [30]. Gastrointestinal intolerance (46 %) is common with the oral solution at doses greater than 400 mg per day and may require discontinuation in up to 30 % of patients. The myocardial depressant effects of itraconazole are also well known and cases of congestive heart failure have been reported [40]. Past studies examining the effects of itraconazole have observed adverse events leading to discontinuation of treatment in up to 30 % of patients [41–43].
15.3.3 Posaconazole
Posaconazole is a lipophilic second-generation antifungal triazole. Its spectrum of activity includes agents of mucormycoses (formerly zygomycoses) and has improved in vitro activity against Aspergillus spp. compared to itraconazole.
Posaconazole is insoluble in water and no intravenous formulation is currently available and it is thus administered as a cherry-flavored suspension. Absorption is maximized when posaconazole is given as 2–4 divided doses administered with food or a liquid nutritional supplement. H2-receptor antagonists and proton pump inhibitors may decrease posaconazole serum levels and, if possible, coadministration should be avoided.
Posaconazole has demonstrated dose-dependent pharmacokinetics with saturable absorption above 800 mg per day; thus, oral loading is not possible therefore delaying attainment of therapeutic drug levels [44]. This prolonged time required to reach steady-state impacts the use of posaconazole as primary therapy for IFIs. Posaconazole is hepatically metabolized and renal clearance plays a minor role in the clearance of posaconazole which is predominantly eliminated fecally.
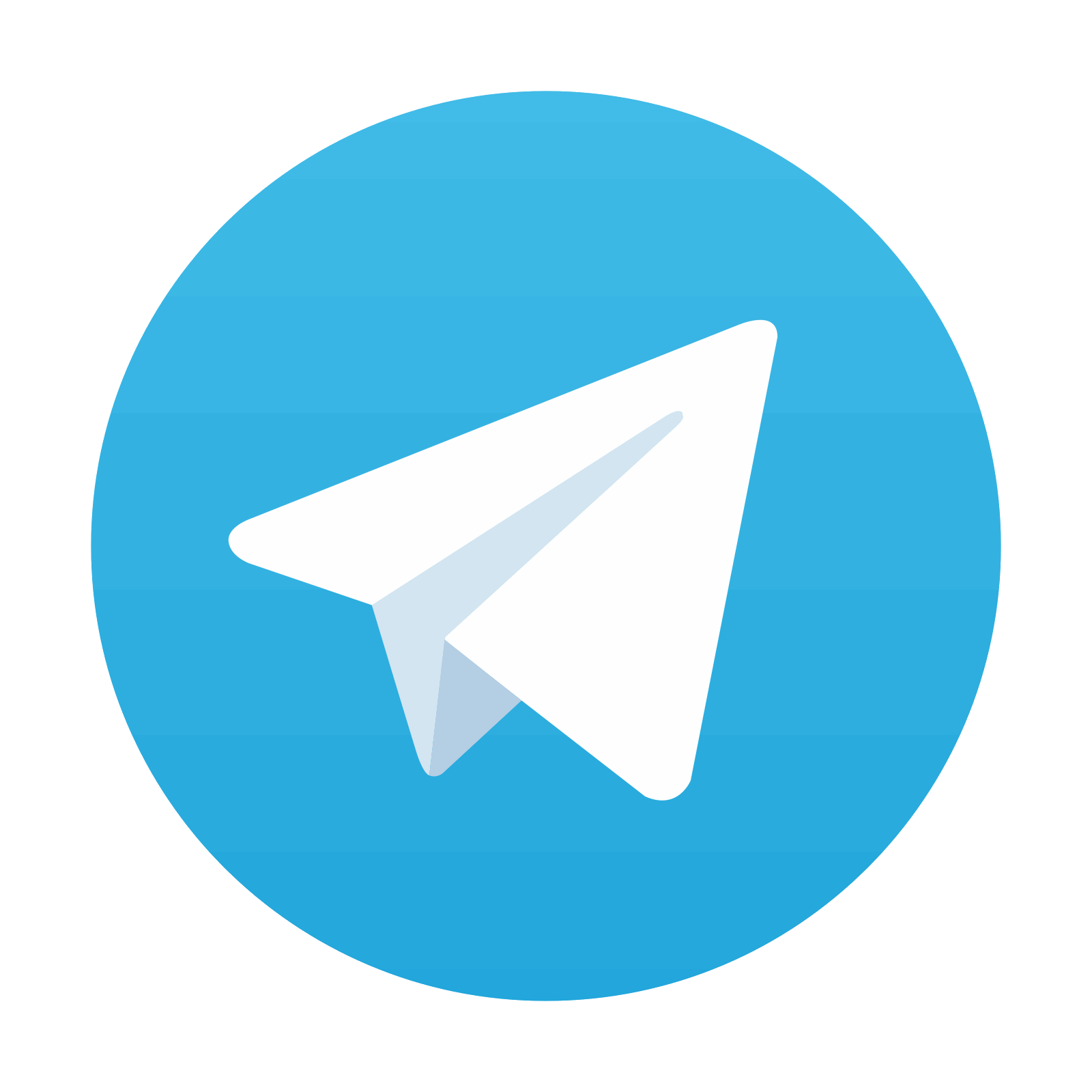
Stay updated, free articles. Join our Telegram channel

Full access? Get Clinical Tree
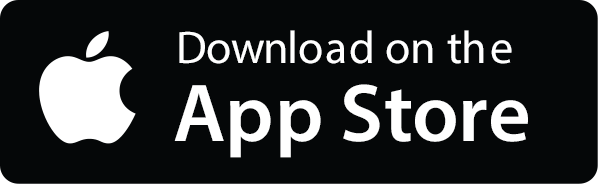
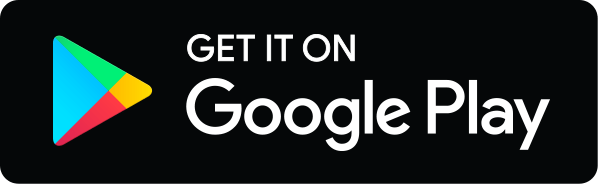