18 Andrew M Salter University of Nottingham The use of animals in research represents one of the most emotive topics on the scientific agenda. The population generally falls into two groups: those who on moral grounds oppose all animal experimentation; and those who support a well-controlled and regulated system that allows animal experimentation, but with strict consideration of animal welfare and the minimisation of pain and suffering. This is certainly not a new debate; in the UK the first legislation regulating the use of animals in experiments was introduced in 1876. A swell of public opinion against animal experimentation, which clearly caused suffering and pain to animals, led to a debate over the regulation of such procedures in Victorian England that included Charles Darwin himself. This resulted in the passing of the Cruelty to Animals Act 1835, which included a system of licensing and inspection of laboratories and the requirement that experiments likely to cause pain and suffering were regulated and licensed by the Home Office. This legislation, which imposed fairly minimal penalties, was finally replaced by a much stricter system of licensing and regulation over a century later in the Animal (Scientific Procedures) Act 1986. More recently, a European Union Directive (2010/63/EU) has set minimum standards for the regulation and monitoring of animal experimentation across the EU. Around the world the specific nature of laws and guidelines relating to experimentation with animals varies substantially, but most seek to limit the amount of pain and suffering that can be inflicted on animals. However, for a significant number of individuals such legislation is still not sufficient and they continue to call for a ban on animal experimentation. A frequently used argument is that no animal is a suitable model for humans and thus all such experiments are fundamentally flawed. Scientists fully accept the limitations of animals as models of humans, but point to the valuable contribution that animal experiments have made to human health and well-being. The aim of this chapter is to guide the reader through some of the contributions that animal experimentation has made, and is making, to our understanding of human nutritional requirements and the impact of nutrition on lifelong health and well-being. It is also important to note that not all nutritional experiments on animals have been undertaken to model humans. The extensive and precise science of farm animal nutrition has made major contributions to the efficiency and capacity of animal agriculture, the benefits of which have played a major role in alleviating hunger and nutrient deficiencies in large parts of the world. In all areas of science there are examples of badly designed experiments that, in hindsight, were never going to achieve the hoped-for outcomes. In most countries, where experiments directly involve human subjects there are processes in place to ensure, at the very least, that the potential to cause harm, suffering or loss of dignity is minimised and that the experiments are designed in such a way as to maximise the chances of testing the stated hypothesis. In human nutrition research this means that most interventions will be aimed at reducing disease risk or symptoms or, at worst, looking at their impact on biomarkers of disease. When using an animal model, the scientist potentially has the opportunity to test an intervention that can cause illness, pain or even death. However, in many countries society demands, and often enshrines in law, an obligation that animal experimentation undergoes similar ethical review to that in humans. Central to such regulation is the process, first described by UK scientists William Russell and Rex Burch in 1959, of the 3Rs: replacement, reduction and refinement. In essence these can be defined as: It is probably fair to say that the widespread adoption of the principle of the 3Rs following a House of Lords Review on Animals in Scientific Procedures in 2002 has substantially improved the quality of animal experimental design, as well as addressing public concern. Through the adoption of such principles one might expect the number of animals used in experimentation to have reduced; however, as will be discussed later in this chapter, the development of techniques to genetically manipulate animals (predominantly mice), often to produce more relevant models of human disease, has in fact increased the total number of animals used. Figure 18.1 shows recent trends in the number of normal and genetically manipulated (GM) animals used in regulated procedures in the UK. It can be seen that over the period 2002–11 the number of normal animals used remained stable, while the use of GM animals has more than doubled. Of the GM animals used, 92% were mice and 67% were specifically used for breeding purposes. It is of note, however, that even including GM animals, the total of approximately 3.8 million procedures carried out in 2011 is substantially less than the peak in use of animals seen in the 1970s, when annual totals frequently exceeded 5 million. Figure 18.1 A comparison of the use of normal and genetically modified (GM) animals in biological research, 2002–2011. Redrawn from data obtained from Home Office (2012) Statistics of Scientific Procedures on Living Animals. Great Britain 2011. Stationery Office, London. Figure 18.2 shows the use of different species in regulated procedures in the UK in 2011. It can be seen that 71% of all animal experiments were carried out on mice. Approximately half of these were undertaken on normal animals, while the other half represented those either presenting with a genetic mutation or specifically genetically manipulated. Many people will be surprised at the relatively small proportion (7%) of rats used, the number of which fell from 510 000 in 2002 to 202 000 in 2011. This, however, probably does not reflect the use of these species in nutrition research. A crude PubMed search for the year 2011 using the search terms rat/mouse and nutrition yielded similar numbers of publications, 990 and 1124 for rat and mouse respectively. A search for transgenic mouse and nutrition yielded only 220. Figure 18.2 Percentage distribution of the use of different species in licensed procedures in the UK in 2011. Redrawn from data obtained from Home Office (2012) Statistics of Scientific Procedures on Living Animals. Great Britain 2011. Stationery Office, London. Why is the rat such a popular species to study in terms of nutritional research? Pragmatically, rats are relatively cheap to buy, house and feed in carefully controlled environments, they are very compliant to changes in diet, they are omnivorous, will eat ‘almost anything’ and there are specific, well-defined genetic strains. Decades of research have carefully defined the nutritional requirements of rats and it is relatively easy to design an experimental diet that is guaranteed to meet those nutritional requirements while testing the effects of single components. However, there are some obvious disadvantages of this species. They are relatively short-lived and not particularly susceptible to some of the chronic diseases associated with ageing humans (particularly cardiovascular disease). Their gastrointestinal system varies in a number of significant ways to that of the human, including the presence of a well-developed caecum and the absence of a gall bladder. A number of important differences also exist in terms of metabolism, including relatively high rates of lipogenesis in adipose tissue and liver, high rates of hepatic cholesterol synthesis, but low levels of plasma cholesterol, almost all of which is normally carried in the high-density lipoprotein fraction. Perhaps most importantly, some of the very things that make the rat so attractive can potentially reduce its value as a model for humans. The human response to a particular nutrient, food or diet can be influenced by genetic polymorphisms, epigenetic changes in gene expression and the nature of the gut microflora, all things that we have sought to minimise by careful breeding and regulation of experimental animal housing environments. Thus, while the rat may represent a useful model of the essentiality of specific nutrients in the diet, it may be less useful in predicting the impact of a specific dietary change on susceptibility to a chronic disease in humans. Nevertheless, as a tool for proof of principle they can be invaluable. The mouse exhibits many of the strengths and weaknesses of the rat in modelling human nutrition. However, inbreeding in mice has produced a wide array of strains with different phenotypes and susceptibilities to disease, which have been used in understanding the interactions between genotypical, phenotypical and environmental (including dietary) influences on disease progression. More recently, the development of techniques for the genetic manipulation of mice has had a profound impact on laboratory animal experimentation. It is now possible to knock out or over-express specific genes in the whole body or in a tissue-specific way. Genes can be incorporated into the mouse genome from other species (including humans) that can specifically alter susceptibility to disease. While such technologies have made an immeasurable contribution to our understanding of the relationship between genetic and environmental influences on disease susceptibility and progression, they have also highlighted the ‘polygenic’ nature of many of the chronic diseases faced by the human race. In the vast majority of individuals, increased susceptibility to diet-induced diseases, including cardiovascular disease, diabetes and cancer, is the result of multiple gene polymorphisms rather than mutations at a single gene locus. While mice and rats have been extensively used in modelling human nutrition, they are certainly not the only species that have made contributions to our understanding. Other small mammals, including rabbits, guinea pigs and hamsters, have also been shown to represent important models of specific aspects of the interactions between diet and disease. While these species can sometimes exhibit phenotypes that model important aspects of human biochemistry and physiology, their use has been hampered by the lack of ‘molecular tools’ that are widely available for the study of mice and rats. Larger animal species have made important contributions to our understanding of nutritional principles. The understanding of the nutritional requirements of farm animals, primarily for the purposes of improving the efficiency of human food production, has made an immense contribution to the overall science of nutrition. The highly developed nature of the gastrointestinal tract and metabolism of ruminants, which allows them to exist on high-cellulose, plant-based diets, has limited their usefulness as a model of human nutrition and disease. However, the pig is frequently cited as an under-utilised model of human nutrition. This truly omnivorous species is relatively long-lived and displays many biochemical and physiological similarities to humans that would make it particularly attractive for the study of the impact of nutrition on the chronic disease of ageing. However, their size and dietary intake make them an expensive choice of model that can generally only be studied by those institutions with specialist facilities. Other species that have historically made important contributions to our understanding of nutrition include cats, dogs and primates. Their use in animal experimentation is highly regulated and, except for very specific reasons, they are rarely used as models of human nutrition. The use of dogs in animal experimentation in the UK is now largely restricted to pharmaceutical safety testing, while the total number of cats used in regulated procedures in the UK in 2011 was only 235. One area where primates are yielding potentially interesting results in the USA is on the impact of ‘calorie restriction’ on longevity, which will be discussed later in this chapter. The choice of species to be used in an experiment modelling human nutrition is vital, but experimental design is equally important. Factors that are perhaps most frequently overlooked in experimental design include the dose and the form in which a nutrient is delivered. For example, the beneficial effects of fruit and vegetables on human health are broadly accepted across the world. Numerous research groups have used animal experiments to attempt to identify specific phytonutrients that may contribute to their beneficial effects. However, such studies frequently use specific chemical entities extracted (often by extensive chemical processes) from plant material and fed at doses dramatically higher than found in natural diets, with little consideration of bioavailability or metabolism of the ‘active’ compound, either by the gut microflora or the host animal itself. From the seventeenth century onwards, animal experiments have been used to develop the basic principles on which modern nutritional science is based. It is not the aim of this chapter to try to document all of this history. However, some examples are presented of the way in which in these early animal experiments have shaped our understanding of such principles. Demonstrating a connection between combustion and life has been accredited to John Mayow of London in the seventeenth century, who showed that if a candle and an animal were placed in a confined, sealed space, both ‘died’ simultaneously. Laviosier, working in the late 1700s, went on to identify oxygen consumption and carbon dioxide production as the common factor in both the burning of the candle and the ‘respiration’ of the animal. He then developed the earliest whole-body calorimeter, which demonstrated that heat production by an animal was directly proportional to oxygen consumed. Subsequent work in the mid-nineteenth century by Regnault and Reiser, working in species as diverse as earthworms, frogs, rabbits and dogs, helped develop the principle of the respiratory quotient (RQ), which showed that the ratio of CO2 produced to O2 consumed varied depending on the nature of the food consumed. Around the same era, animal experiments indicated that carbohydrate, fat and protein were all potential ‘metabolic fuels’ that can provide energy for the body. This was encapsulated in Rubner’s Isodynamic Law, which indicated that, on an energy-equivalent basis, carbohydrate, fat and protein (when adjusted for urea excretion) are interchangeable as fuel sources. However, it was not until the end of the nineteenth century, when Atwater and colleagues designed and built the first calorimeter that could accommodate human subjects, that it was generally accepted that this principle also applied to people. The first half of the twentieth century is often regarded as the ‘golden years’ of nutritional science. It was certainly during this period that most of the nutrients essential for life were discovered. The major breakthrough came with a move from using natural foodstuffs to the use of purified diets in animal experiments. In 1913 McCollum reported the results of feeding rats diets containing casein, lard, lactose, minerals and starch. The results showed that while these animals could grow well for up to 14 weeks, they would then stop, or even lose weight. Adding an ether extract of egg or butterfat (but not cottonseed oil or olive oil) to the diet restored growth. It was concluded that such foods contained a lipid-soluble ‘Factor A’, deficiency of which was also shown to cause night blindness and xerophthalmia. This, of course, was the discovery of vitamin A, which was finally isolated from fish oil in 1939. The whole story was, however, complicated by the discovery that a highly coloured extract from leaves and carrots also appeared to relieve such symptoms; this was later found to be β-carotene, from which the active ‘vitamin’ could be derived. At a similar time it was shown that if even more highly purified casein and lactose were used in the diet, a deficiency in a water-soluble ‘Factor B’ resulted in the condition known as BeriBeri. The active factor was later identified as thiamin(e) or vitamin B1.
Animal Models in Nutrition Research
18.1 Introduction
18.2 Ethics of animal experimentation
18.3 Choosing the right species
18.4 The role of animal models in the early years of nutritional science
Animal experiments and energetics
Animal experiments and essential nutrients
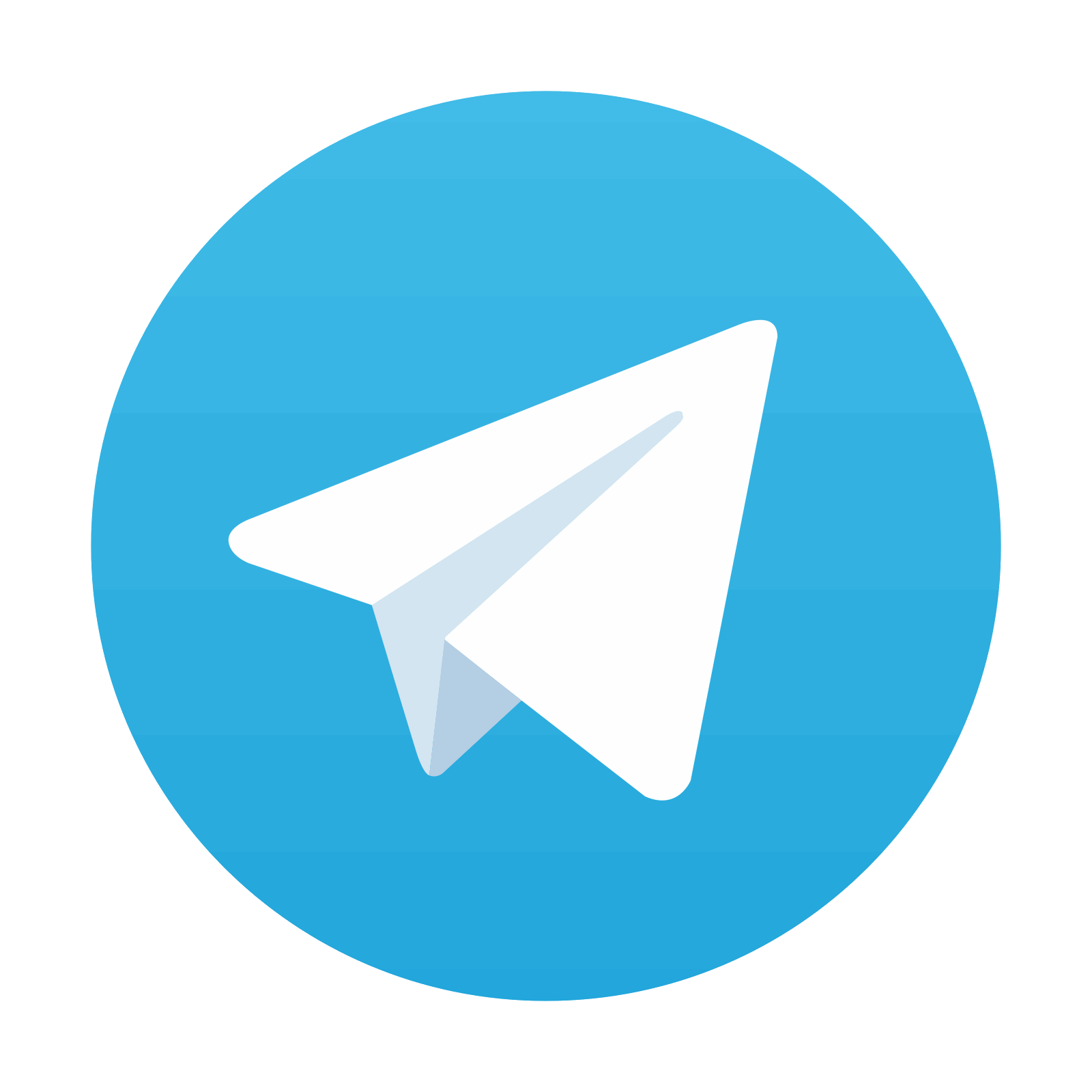
Stay updated, free articles. Join our Telegram channel

Full access? Get Clinical Tree
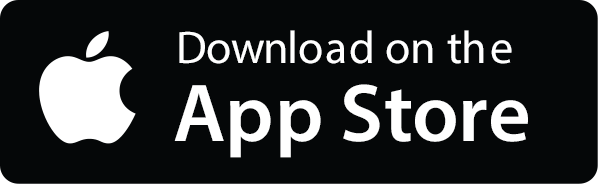
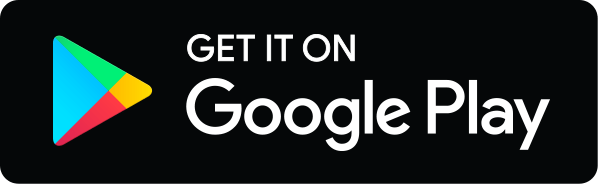