Anemia of inflammation (AI, also called anemia of chronic disease) is a common, typically normocytic, normochromic anemia that is caused by an underlying inflammatory disease. It is diagnosed when serum iron concentrations are low despite adequate iron stores, as evidenced by serum ferritin that is not low. In the setting of inflammation, it may be difficult to differentiate AI from iron deficiency anemia, and the 2 conditions may coexist. Treatment should focus on the underlying disease. Recent advances in molecular understanding of AI are stimulating the development of new pathophysiologically targeted experimental therapies.
Key points
- •
Anemia of inflammation results from hepcidin-induced hypoferremia combined with cytokine-mediated suppression of erythropoiesis and decreased lifespan of erythrocytes.
- •
Treatment of the cause of inflammation improves the anemia.
- •
Treatment with erythropoiesis-stimulating agents and/or intravenous iron is rarely necessary.
Clinical presentation
- •
Mild to moderate anemia (hemoglobin rarely <8 g/dL)
- •
Occurring in a setting of infection, inflammatory disease, or malignancy
- •
Low serum iron
- •
Systemic iron stores not depleted
Definitions
Anemia of inflammation (AI, formerly also called anemia of chronic disease or anemia of chronic disorders) is usually a mild to moderately severe anemia (hemoglobin rarely lower than 8 g/dL) that develops in the setting of infection, inflammatory disease, or malignancy. The defining biochemical features of AI include low serum iron despite adequate systemic iron stores. The concentration of serum transferrin is also decreased during chronic inflammation but this is a lagging indicator because of the long half-life of transferrin (about 8 days) compared with iron (about 1.5 hours). The erythrocytes are usually of normal size and have normal hemoglobin content but are reduced in number (normocytic, normochromic anemia). In some cases, particularly if the inflammatory disease is longstanding, the red cells are mildly decreased in size and hemoglobin content.
Related Conditions
Anemia of critical illness presents with a similar pattern of findings but develops within days in patients who are hospitalized in intensive care units with infections, sepsis, or other inflammatory conditions. Anemia of critical illness may be exacerbated by frequent diagnostic phlebotomies or increased gastrointestinal blood loss as is common in such settings. Anemia of aging is a chronic anemia similar to AI but often occurring in the elderly without a specific diagnosis of a predisposing underlying disease. The prevalence of this anemia increases with age, and detailed studies often detect evidence of inflammation, including increased serum C-reactive protein or other biomarkers of inflammation. Anemia of chronic kidney disease is commonly attributed to erythropoietin deficiency but accumulating evidence favors a more complex pathogenesis with a large component of AI whose exacerbations may be manifested as “erythropoietin resistance”.
Diagnosis
The traditional gold standard for the diagnosis of AI was anemia with hypoferremia or with low transferrin saturation, despite the presence of Prussian blue stainable iron in bone marrow macrophages. The main confounding diagnostic entity that also presents with anemia and hypoferremia is iron deficiency anemia where there is no stainable iron in the marrow macrophages. This gold standard has been challenged not only because of the invasive nature of the marrow sampling procedure but also because of findings that bone marrow iron readings are qualitative and not always consistent between evaluators and in multiple specimens and that iron therapy may cause marrow iron deposition in a poorly bioavailable form, which cannot be used by iron-deficient patients. The marrow iron stain has largely been replaced by serum ferritin determinations. Low serum ferritin (less than 15 ng/mL for general population, with some laboratories using age and gender-specific norms) is highly specific for iron deficiency (genetic deficiency of L-ferritin is an extremely rare exception ) and effectively rules out AI. AI is diagnosed when anemia and hypoferremia are accompanied by serum ferritin that is not low. Serum ferritin is increased by inflammation, in part reflecting direct inflammatory regulation of ferroportin synthesis and in part because serum ferritin originates in macrophages where its synthesis is increased by iron sequestration that takes place during inflammation. Iron deficiency is presumed to coexist with AI when ferritin is insufficiently elevated for the intensity of inflammation. Serum ferritin is also increased by tissue injury, especially to the liver.
Diagnostic Challenges
The determination of what constitutes “inappropriately low” ferritin may be difficult in practice because even patients with very high serum ferritin levels may respond to intravenous iron therapy by increasing hemoglobin. In principle, the limitations of serum ferritin could be circumvented by assaying additional markers of iron deficiency less affected by inflammation, most prominently soluble transferrin receptor. However, the relevant assays have not been standardized, the added value of such studies has not yet been convincingly demonstrated, and none have been widely adopted. When the anemia is clinically significant and a component of iron deficiency is suspected in a patient with AI, it may be reasonable to perform a therapeutic trial of intravenous iron. Current intravenous iron preparations are quite safe, but the very rare reactions to their administration and the possibility of exacerbating an existing or occult infectious process should be included in the risk-benefit analysis.
Prevalence
Detailed statistics about the prevalence of AI are not available. It is estimated that the aging of the population and the high prevalence of chronic infections and inflammatory disorders worldwide combine to make AI the second most common cause of anemia worldwide, after iron deficiency. The order may eventually reverse as iron deficiency anemia is more effectively treated or prevented by dietary iron supplementation and by public health measures that curb intestinal parasitic infections.
Clinical presentation
- •
Mild to moderate anemia (hemoglobin rarely <8 g/dL)
- •
Occurring in a setting of infection, inflammatory disease, or malignancy
- •
Low serum iron
- •
Systemic iron stores not depleted
Definitions
Anemia of inflammation (AI, formerly also called anemia of chronic disease or anemia of chronic disorders) is usually a mild to moderately severe anemia (hemoglobin rarely lower than 8 g/dL) that develops in the setting of infection, inflammatory disease, or malignancy. The defining biochemical features of AI include low serum iron despite adequate systemic iron stores. The concentration of serum transferrin is also decreased during chronic inflammation but this is a lagging indicator because of the long half-life of transferrin (about 8 days) compared with iron (about 1.5 hours). The erythrocytes are usually of normal size and have normal hemoglobin content but are reduced in number (normocytic, normochromic anemia). In some cases, particularly if the inflammatory disease is longstanding, the red cells are mildly decreased in size and hemoglobin content.
Related Conditions
Anemia of critical illness presents with a similar pattern of findings but develops within days in patients who are hospitalized in intensive care units with infections, sepsis, or other inflammatory conditions. Anemia of critical illness may be exacerbated by frequent diagnostic phlebotomies or increased gastrointestinal blood loss as is common in such settings. Anemia of aging is a chronic anemia similar to AI but often occurring in the elderly without a specific diagnosis of a predisposing underlying disease. The prevalence of this anemia increases with age, and detailed studies often detect evidence of inflammation, including increased serum C-reactive protein or other biomarkers of inflammation. Anemia of chronic kidney disease is commonly attributed to erythropoietin deficiency but accumulating evidence favors a more complex pathogenesis with a large component of AI whose exacerbations may be manifested as “erythropoietin resistance”.
Diagnosis
The traditional gold standard for the diagnosis of AI was anemia with hypoferremia or with low transferrin saturation, despite the presence of Prussian blue stainable iron in bone marrow macrophages. The main confounding diagnostic entity that also presents with anemia and hypoferremia is iron deficiency anemia where there is no stainable iron in the marrow macrophages. This gold standard has been challenged not only because of the invasive nature of the marrow sampling procedure but also because of findings that bone marrow iron readings are qualitative and not always consistent between evaluators and in multiple specimens and that iron therapy may cause marrow iron deposition in a poorly bioavailable form, which cannot be used by iron-deficient patients. The marrow iron stain has largely been replaced by serum ferritin determinations. Low serum ferritin (less than 15 ng/mL for general population, with some laboratories using age and gender-specific norms) is highly specific for iron deficiency (genetic deficiency of L-ferritin is an extremely rare exception ) and effectively rules out AI. AI is diagnosed when anemia and hypoferremia are accompanied by serum ferritin that is not low. Serum ferritin is increased by inflammation, in part reflecting direct inflammatory regulation of ferroportin synthesis and in part because serum ferritin originates in macrophages where its synthesis is increased by iron sequestration that takes place during inflammation. Iron deficiency is presumed to coexist with AI when ferritin is insufficiently elevated for the intensity of inflammation. Serum ferritin is also increased by tissue injury, especially to the liver.
Diagnostic Challenges
The determination of what constitutes “inappropriately low” ferritin may be difficult in practice because even patients with very high serum ferritin levels may respond to intravenous iron therapy by increasing hemoglobin. In principle, the limitations of serum ferritin could be circumvented by assaying additional markers of iron deficiency less affected by inflammation, most prominently soluble transferrin receptor. However, the relevant assays have not been standardized, the added value of such studies has not yet been convincingly demonstrated, and none have been widely adopted. When the anemia is clinically significant and a component of iron deficiency is suspected in a patient with AI, it may be reasonable to perform a therapeutic trial of intravenous iron. Current intravenous iron preparations are quite safe, but the very rare reactions to their administration and the possibility of exacerbating an existing or occult infectious process should be included in the risk-benefit analysis.
Prevalence
Detailed statistics about the prevalence of AI are not available. It is estimated that the aging of the population and the high prevalence of chronic infections and inflammatory disorders worldwide combine to make AI the second most common cause of anemia worldwide, after iron deficiency. The order may eventually reverse as iron deficiency anemia is more effectively treated or prevented by dietary iron supplementation and by public health measures that curb intestinal parasitic infections.
Pathophysiology
- •
Mildly shortened erythrocyte survival (increased destruction)
- •
Hypoferremia, iron-restricted erythropoiesis from cytokine-stimulated hepcidin increase
- •
Suppression of erythropoiesis by direct effects of cytokines on the marrow
- •
Variable effects of inflammation on erythropoietin production, renal excretion of hepcidin
Overview of the Causative Factors
Despite more than 50 years of investigation, our understanding of the pathophysiology of AI is incomplete. Already the earliest studies of AI indicated that the disorder is a consequence of a mild decrease in erythrocyte survival combined with impaired production of erythrocytes. The increased destruction of erythrocytes is predominantly attributable to macrophage activation by inflammatory cytokines but other hemolytic mechanisms may contribute in specific inflammatory diseases. The suppression of erythrocyte production has 2 major components, iron restriction and direct cytokine effects on erythropoietic progenitors. These effects combine to limit the erythropoietic response to erythropoietin, which becomes insufficient to compensate for the increased destruction of erythrocytes. In some situations, the production of erythropoietin may also be decreased, perhaps due to cytokine effects on the renal cells that produce the hormone. In severe inflammation, or when the primary pathology involves the kidneys, decreased renal excretion of hepcidin contributes to hepcidin accumulation and iron restriction. The complex pathogenesis of AI is summarized in Fig. 1 and discussed further.
Erythrocyte Destruction
Experiments with transfused erythrocytes showed that erythrocytes from AI patients and from normal controls survived longer in healthy recipients than in patients with AI. The shortened survival of erythrocytes in AI has been attributed to macrophage activation by inflammatory cytokines that causes the macrophages to ingest and destroy erythrocytes prematurely. Anemia and excessive erythrophagocytosis are prominent features of macrophage activation syndromes, especially those associated with systemic juvenile rheumatoid arthritis. Here, treatment targeting interleukin 1 (IL-1) or IL-6 is proving effective, suggesting an important (although possibly indirect) role of these cytokines in the pathogenesis of excessive erythrophagocytosis. In mouse models, multiple cytokines, including interferon-γ and IL-4, have been implicated in activating macrophages for erythrophagocytosis. With the exception of fulminant hemophagocytic states, which are fortunately rare, erythrophagocytosis in AI is only mildly increased and could be readily compensated if the production of erythrocytes was not also impaired.
Hypoferremia
A recent review of mechanisms governing iron homeostasis is provided elsewhere. Briefly, plasma iron concentrations are under homeostatic control of the hepatic iron regulatory hormone hepcidin and are normally maintained in the 10 to 30 μM range. Hepcidin acts by regulating the iron delivery to plasma from macrophages that recycle senescent erythrocytes, from duodenal enterocytes that absorb dietary iron, and from hepatocytes involved in iron storage. The molecular target of hepcidin is the sole known cellular iron exporter ferroportin, expressed on cell membranes in tissues that deliver iron to plasma. The binding of hepcidin to ferroportin causes ferroportin endocytosis and its subsequent proteolysis in lysosomes. The loss of ferroportin from cell membranes causes a proportional reduction of iron export to plasma. The production of hepcidin by hepatocytes is in turn regulated by plasma and hepatic iron concentrations and inflammatory cytokines, chiefly IL-6. Inflammatory stimuli administered to humans or experimental animals elicit a decrease in serum iron concentration within a few hours. The response depends on inflammation-induced increase in plasma concentrations of hepcidin. Increased hepcidin degrades cellular ferroportin and traps iron in macrophages, hepatocytes, and intestinal enterocytes so that less iron is delivered to plasma transferrin. The plasma iron compartment is then rapidly depleted of iron through continuing iron uptake by erythroid precursors.
Increased Hepcidin Causes an Iron-Restricted Anemia Even in the Absence of Inflammation
An experiment of nature, the syndrome of iron-refractory iron deficiency anemia (IRIDA), provides an important insight into the role of hepcidin in the regulation of erythropoiesis and as a pathogenic component of AI. The otherwise healthy children with IRIDA suffer from a severely microcytic, hypochromic anemia and hypoferremia that respond poorly to treatment with oral iron and incompletely even to treatment with intravenous (IV) iron. Guided by a mouse model of this condition, the pathogenesis of IRIDA is now partially understood. Most of the patients with IRIDA have homozygous or compound heterozygous mutations in the gene encoding the transmembrane serine protease TMPRSS6 (also called matriptase-2), leading to serum hepcidin levels that are high or inappropriately elevated considering that the patients are iron deficient. Hepcidin-mediated block in duodenal iron absorption is likely responsible for the ineffectiveness of oral iron therapy, and hepcidin-induced retention of iron in macrophages reduces the response to IV iron replacement therapy. Importantly, IRIDA patients continue to have microcytosis and hypochromia even after iron therapy, indicating that hemoglobin synthesis is impaired more than the production of erythrocytes. This is in contrast to AI, which is usually a normochromic normocytic anemia, indicating that in AI the impairment of hemoglobin synthesis is roughly balanced by decreased production of erythrocytes. Thus, direct suppression of erythrocyte production by inflammatory cytokines in AI may “compensate” for the effect of hypoferremia on hemoglobin synthesis, generating fewer erythrocytes but with normal size and hemoglobin content.
Suppression of Erythropoiesis by Inflammation
Inflammatory cytokines, including tumor necrosis factor (TNF) α, IL-1, and interferon-γ, have been reported to suppress erythropoiesis in vitro as well as in mouse models. Detailed understanding of the mechanisms involved has been hindered by the complexity of cytokine effects and the ability of each cytokine to regulate the production of many other cytokines. Nevertheless, several new and promising concepts about the effects of cytokines on erythropoiesis have recently emerged. Libregts and colleagues developed a mouse model where overproduction of interferon-γ leads to the development of a mild-to-moderate normocytic, normochromic anemia. The model manifests a 50% decrease in erythrocyte survival attributable to interferon-γ–mediated activation of macrophages in the splenic red pulp. The model also shows suppression of erythrocyte production affecting the erythroblast stages and the earliest erythroid-committed precursor burst-forming unit–erythrocyte but not proerythroblasts and colony-forming unit–erythrocyte (CFU-E). Importantly, myeloid CFU-granulocyte/macrophage colonies were increased. Microarray analysis of erythroblasts indicated that interferon-γ promotes the transcription of PU.1 and its target genes in an interferon regulatory factor 1–dependent manner but does not affect GATA-1 or its targets. PU.1 and GATA-1 antagonize each other’s activity, so the increase in PU.1 would be expected to promote myelopoiesis at the expense of erythropoiesis. During infections with viruses or intracellular pathogens known to induce interferon-γ, this mechanism may assure sufficient production of monocytes and macrophages, at the expense of temporary impairment of erythropoiesis. Whether other inflammatory cytokines use a similar or different mechanism remains to be determined.
Hepcidin-induced Hypoferremia and Interferon-γ Synergize to Suppress Erythropoiesis
Richardson and colleagues examined how inflammatory cytokines and hypoferremia interact to affect erythropoiesis during AI. Using in vitro culture of human CD34+ primary progenitors, they documented that hypoferremia (transferrin saturation ≤15%) potentiates the suppressive effects of TNF-α and interferon-γ on erythropoiesis. Surprisingly, erythropoietic suppression could be reversed by the addition of the Krebs cycle intermediate isocitrate, a product of the enzyme aconitase, which also functions as a cellular iron sensor. Isocitrate injections also reversed AI in a rat model of autoimmune arthritis induced by injection of streptococcal peptidoglycan-polysaccharide. The authors present evidence that hypoferremia activates PU.1 via a protein kinase C pathway, synergizing with the effect of interferon-γ. Isocitrate, acting on aconitase, reverses the effect of hypoferremia on PU.1 and relieves the suppression of erythropoiesis. It remains to be seen if these effects are important in other animal models and in human subjects.
Animal Models of AI Show Partial Dependence on Hepcidin
A new mouse model of AI was generated by a single intraperitoneal injection of heat-killed Brucella abortus . Like human AI, this model showed multifactorial pathogenesis including iron restriction from increased hepcidin, transient suppression of erythropoiesis, and shortened erythrocyte lifespan. Mice developed severe anemia with mild microcytosis and mild hypochromia, a hemoglobin nadir at 14 days and partial recovery by 28 days. After an early increase in inflammatory markers and hepcidin, the mice manifested hypoferremia despite iron accumulation in the liver. Erythropoiesis was suppressed between days 1 and 7, and erythrocyte destruction was increased as evidenced by shortened red blood cell lifespan and rare schistocytes on blood smears. Erythropoietic recovery began after 14 days but was iron-restricted, as documented by increased erythrocyte zinc protoporphyrin. In mice with ablated hepcidin-1 gene, anemia was milder, not iron-restricted, and with faster recovery, supporting the role of hepcidin in the development of AI.
In the same mouse model of AI, the therapeutic administration of antihepcidin monoclonal antibodies decreased the severity of anemia. Moreover, resistance to exogenous erythropoietin doses observed in this model was relieved by coadministration of the antibodies with erythropoietin. In the rat model of autoimmune arthritis induced by injection of streptococcal peptidoglycan-polysaccharide, suppressing hepcidin production by administration of the dorsomorphin derivative LDN-193189 or soluble hemojuvelin-Fc fusion protein, 2 agents that interfere with bone morphogenetic protein receptor signaling, also ameliorated anemia.
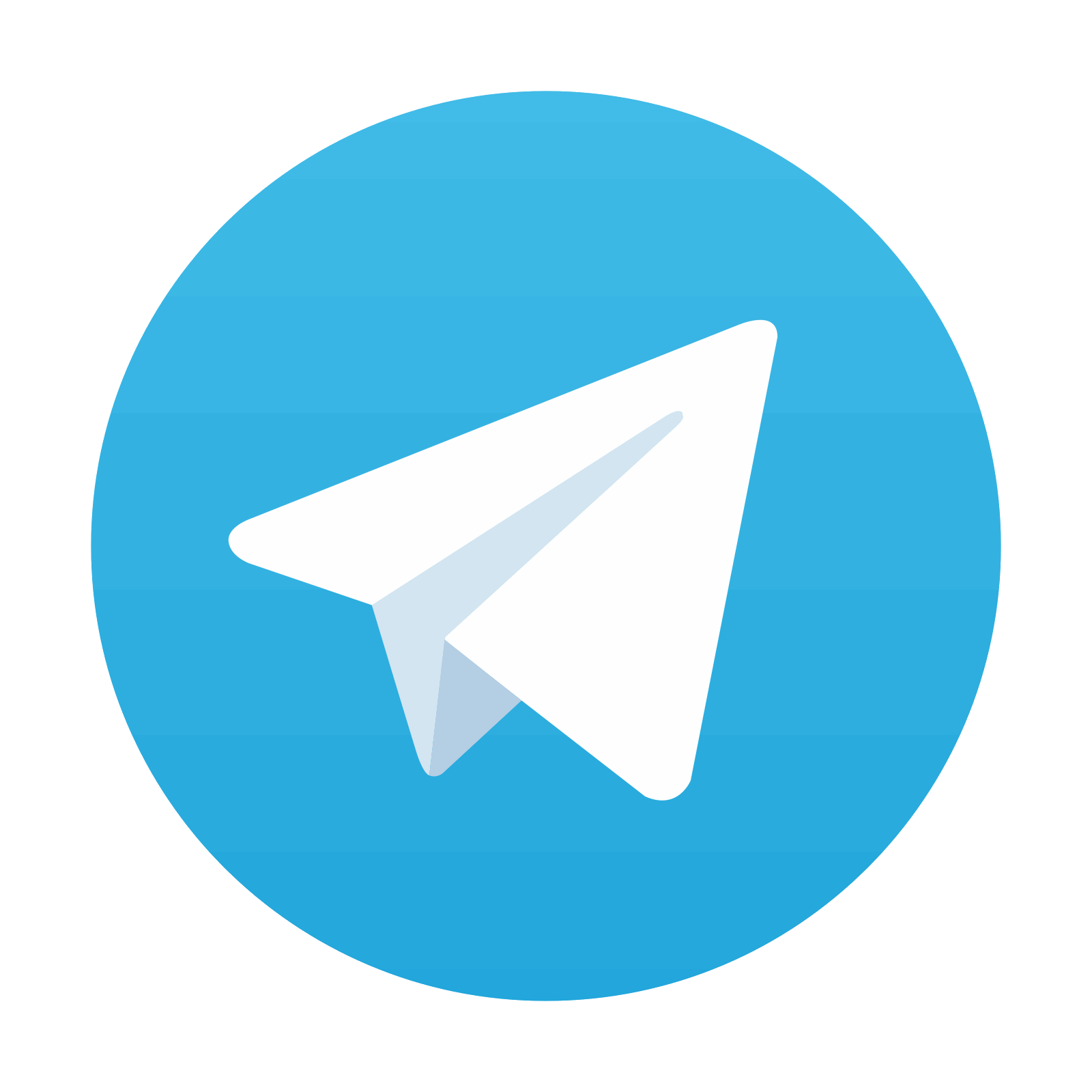
Stay updated, free articles. Join our Telegram channel

Full access? Get Clinical Tree
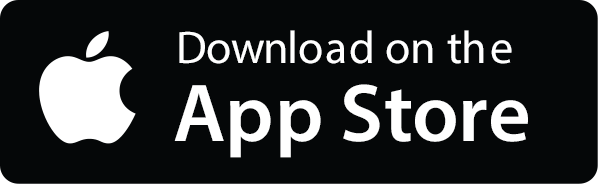
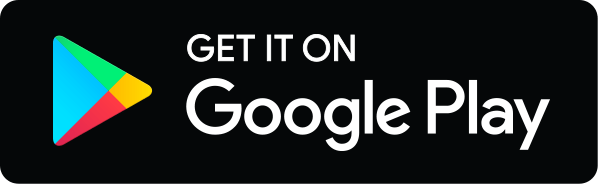