Fig. 5.1
Schematic of the full-length androgen receptor and exon structure of major splice variants (ARV7 (b) and ARV567 (c)). Domains of AR include the amino (N) terminal domain, the DNA-binding domain (DBD), the hinge-region (HR), and the carboxy (C)-terminal ligand-binding domain (LBD). Mutations which occur in specific regions are indicated (modified from Mostaghel et al. [70])
In the absence of ligand, the AR is primarily located in the cytoplasm and bound to chaperone or heat shock proteins (such as HSP-90) which maintain receptor conformation and prevent degradation. The AR is activated by interaction with multiple steroid hormones, including testosterone (T), dihydrotesterone (DHT), and adrenal androgens, though the latter interaction occurs with significantly lower affinity. Binding of steroid releases receptor chaperones and leads to conformational activation, receptor dimerization, phosphorylation, and nuclear translocation. The dimerized AR then binds to target DNA sequences (AREs) in promotor and enhancer regions of androgen-responsive genes, leading to selective recruitment of coregulator proteins and closely regulated activation or repression of gene expression.
Activation of the AR Axis in Castration Resistant Prostate Cancer
Like other hormones, AR signaling can mediate pleiotropic effects through affecting a broad array of genes that regulate cell cycle, survival, and proliferation [4–7]. Interference with androgen–AR interaction, such as by serum androgen suppression (androgen deprivation therapy), eliminates these signals and induces nearly universal responses in the treatment of prostate cancer as measured by declines in PSA and control of symptoms in symptomatic patients. Early events after initiating blockade of AR signaling (days 0–3) include downregulation of AR and expression of the negative cell cycle regulators, p21 and p27. Apoptosis resulting from AR signaling blockade ranges from 0 to 20 % within the first 7 days depending on the method of assay and the tissue evaluated [8, 9]. Apoptosis after androgen deprivation in men treated with castration increases within the first 24 h, with a maximum effect (2.5–3 % apoptosis) at 3–4 days with subsequent declines in the numbers of apoptotic cells to baseline over the following week [10, 11]. Subsequently (following day 3), the proliferation rate begins to decrease (as measured by Ki67 staining) and is associated with increase in cell cycle arrest in prostate cancer xenografts.
Despite initial responses to AR signaling blockade, the majority of cancers become resistant to androgen deprivation using the currently available agents. This disease state was initially considered a “hormone refractory” phenotype as most patients maintained anorchid serum testosterone levels. However, intra-tumoral AR signaling continues to drive cancer progression despite low testosterone blood levels, therefore this state is now considered “castration resistant” prostate cancer (CRPC). The increase in serum PSA in the context of clinical progression serves as compelling evidence supporting the importance of AR signaling in CRPC since PSA is directly and exclusively driven through AR-mediated transcription. Molecular analysis of tumor tissue demonstrates that the majority of the AR transcriptional program is reactivated in progressive CRPC [12]. Intra-tumor androgen levels in metastases from CRPC patients exceed intra-tumor androgen levels in primary prostate tumors from treatment naïve patients [13]. Sources of intra-tumor androgens outside the testes potentially include circulating adrenal androgens, as well as intracrine androgens synthesized de novo within prostate cancer cells [13–15].
Adaptation to the CRPC state also involves substantial upregulation of AR expression; not only by amplification of the AR locus (as seen in 20–30 % of CRPC tumors), but also by increased transcription and stabilization of mRNA or protein [16]. Increased AR expression serves to overcome castrate androgen levels, and has been shown to be both necessary and sufficient in inducing tumor growth in prostate cancer models [17].
Strong confirmatory evidence that both tissue androgens and AR itself are drivers of CRPC comes from the phase III studies of next generation agents targeting these two arms of AR biology. Abiraterone is a selective inhibitor of the steroidogenic enzyme CYP17 and suppresses both serum and tissue androgen levels more effectively than standard androgen deprivation [18–20]. This agent, combined with LHRH agonists, provided survival and quality of life benefits in both chemotherapy-naïve and post-docetaxel CRPC populations, leading to FDA approval in both settings and confirming that androgens drive disease progression in CRPC [21, 22].
Enzalutamide (formerly MDV3100) is a competitive AR antagonist that binds AR with 5–8 fold greater affinity than earlier anti-androgens. In the phase III randomized (AFFIRM) study, enzalutamide improved overall survival by 37 % compared to placebo in men with CRPC previously treated with docetaxel [23], again confirming that therapy directly targeting AR provides clinical benefit in CRPC [24].
AR-Specific Adaptation in CRPC
The selective pressures of androgen deprivation and AR antagonism lead to mutation and aberrant transcription of AR that minimize or eliminate the need for DHT and testosterone. AR mutations were initially identified in the LBD in a high proportion of cancer from patients with CRPC [25], suggesting these mutations as primary drivers of resistance to hormone therapy. Subsequently, multiple classes of AR mutations have been identified in additional regions of the receptor, which result in broadening of ligand specificity and/or conversion of antagonists to agonists (Fig. 5.1) [26, 27]. Evidence also suggests that AR mutations are more prevalent in patients previously treated with first generation AR antagonists such as flutamide and bicalutamide [28]. The frequency of AR mutation in CRPC tumors treated with LHRH agonist or orchiectomy alone is low (8–25 %), suggesting that anti-androgen exposure drives mutation, but that mutation does not a primary driver resistance to ADT alone [32, 28]. More recent studies are using whole exome sequencing of metastatic CRPC in patients before and after treatment with abiraterone and enzalutamide to describe the presence of AR mutations. These studies may reveal that AR mutation becomes a more critical mechanism of secondary resistance under the selection pressure of more effective AR axis blockade. Hints that this may be the case come from several studies documenting a novel AR mutation (F876L) generated by in vitro selection with enzalutamide. ARF876L renders the next generation AR antagonists, enzalutamide and ARN-509, into AR agonists [29–31]. ARF876L have been detected in plasma cell-free DNA from patients progressing on enzalutamide and ARN-509, suggesting that this mutation may be clinically relevant. Further interrogation of ARF876L from patients progressing on abiraterone and enzalutamide, including in metastases, will determine the degree to which ARF876L and other AR mutant forms affect resistance in the context of more complete suppression of AR signaling.
An alternative AR-mediated mechanism of resistance to androgen deprivation is the induction of alternative pre-mRNA splicing to generate constitutively active AR species. During pre-mRNA alternative splicing, aberrant combinations of coding (exons) and non-coding (introns) regions of pre-mRNA transcripts from a single gene and are translated into protein isoforms with differing biological functions. Recent evidence suggests that aberrant AR splicing results in AR variants (ARVs) lacking the LBD and expressing ligand-independent constitutive activity [32–37] (Fig. 5.1). These ARVs can homodimerize or heterodimerize with full-length AR and initiate AR signaling in the absence of ligand [36]. Although a large number of ARVs have been described from cell lines [38], only ARV7 and ARV567 variants have been commonly identified in human CRPC tumors [32, 34, 36]. ARV7 and ARV567 exhibit both unique and overlapping transcriptional programs compared to wild-type AR [39]. While ARVs lacking the LBD drive ligand-independent growth when evaluated in prostate cancer models, the precise role of ARVs in prostate cancer development and progression remains controversial. However, a number of findings suggest that ARVs play a role in prostate cancer pathogenesis: First, ARV7 can be identified in normal prostate epithelium and is associated with a shorter time to recurrence after prostatectomy [34, 35]. Second, murine transgenic expression of ARV567 in prostate epithelium leads to adenocarcinoma by 50 weeks (S. Plymate, in preparation). Finally, increased levels of ARV7 and ARV567 were associated with shorter survival in patients with CRPC and bone metastases [32, 34, 35].
A recent debate has focused on whether the presence of ARVs is relevant given that the relative proportion of ARVs vs. wild-type AR in experimental settings, with splice variants found at levels a log lower than wild-type. It is important to note that a subgroup of bone metastases demonstrated nearly equivalent protein levels of full-length and truncated ARVs by western blot [32].
The induction of ARVs following castration may be an important step in prostate tumor survival, or may provide a bridge to induction of additional tumor growth mechanisms [40]. Additional controversy arises regarding whether outgrowth of ligand-independent tumor cell clones ARVs are generated primarily through aberrant splicing or through genomic rearrangement of AR [41].
Adaptation in AR-Regulated Pathways
Recent work suggests that the mechanism by which AR undergoes nuclear translocation occurs through binding of a canonical nuclear localization signal in exon 4 within AR to cellular microtubules at dynein motors [42, 43]. Although the activity of taxanes in other malignancies is primarily ascribed to microtubule stabilization, it has been proposed that this unlikely to be the primary mechanism of action in prostate cancer due to its relatively slow proliferation [44]. In a recently proposed model, taxanes inhibit prostate cancer growth not only through G2-M arrest, but also through inhibition of AR translocation to the nucleus. This model is supported by the correlation of cytoplasmic sequestration of AR in circulating tumor cells (CTCs) with clinical response to taxane therapy [44].
Importantly, taxane-mediated nuclear exclusion may affect ARVs differently compared to wild-type AR. The nuclear localization signal of ARV7 is located in the cryptic exon and is distinct from that of wild-type AR and ARV567 [45]. As a result, ARV7 is resistant to taxane-mediated nuclear exclusion, potentially abrogating taxane efficacy [46]. This may be clinically relevant since not only does preclinical data demonstrate that ARVs contribute to resistance to abiraterone and enzalutamide, but emerging clinical data also suggests patients with tumors resistant to abiraterone and enzalutamide are less sensitive to docetaxel [47]. This implies that ARV expression may regulate sensitivity to both AR targeting agents and taxanes, and, further suggests that targeting the NTD may enhance taxane efficacy in ARV-expressing tumors.
Nuclear Receptor Superfamily Crosstalk in AR Signaling
Androgen deprivation exerts selection pressure to preserve AR signaling in two ways: First, it can broaden AR ligand specificity to include ligands of the closely related steroid receptor superfamily (e.g., progesterone, cortisol). Second, other members of the nuclear receptor superfamily (e.g., glucocorticoid receptor (GR), mineralocorticoid receptor and progesterone receptor) can be recruited to maintain AR signaling. These receptors have strong sequence homology in the DBD to AR, and may maintain AR signaling in the androgen-deprived state by allowing access to transcription factor binding sites in AR-regulated genes. For example, the GR shares transcriptional response elements with AR in multiple gene targets, and activates a transcriptional program largely overlapping with that activated by AR [48]. The FOXA1 transcription factor regulates differential binding of GR and AR to gene targets, and thereby regulates GR function in prostate cancer [48]. The strongest preclinical and clinical evidence to support a role for GR in castration resistance comes from a study characterizing prostate cancer cell lines selected for resistance to ARN-509 and enzalutamide [49]. Glucocorticoid receptor was markedly upregulated as measured by expression array analysis and confirmed by Western blot, and GR knockdown partially abrogated the enzalutamide-resistant phenotype when cell lines were grown as xenografts [50]. Moreover, AR and GR share largely overlapping cistromes and transcriptional programs. Evaluation of bone metastases in patients treated with enzalutamide has demonstrated increased expression of GR in enzalutamide-resistant metastases compared to enzalutamide-responsive metastases.
A post-hoc analysis of the phase III AFFIRM trial (a study of enzalutamide in patients previously treated with docetaxel) suggested that use of glucocorticoids was associated with inferior survival (independent of other known prognostic factors) and could be a factor in driving adverse biology [51]. A similar analysis of the phase III COU-301 trial (a study of abiraterone in patients previously treated with docetaxel) suggested that use of glucocorticoids was associated with greater comorbidity and worse disease outcomes [52]. A more thorough understanding of how GR biology drives progression in CRPC and the impact of glucocorticoid use in patients with CRPC will require additional clinical and translational studies.
Growth Factor Crosstalk in AR Signaling
A number of signaling pathways, including receptor/nonreceptor tyrosine kinases and cytokines, have been proposed to mediate ligand-dependent and independent AR activation. The proposed mechanism is that activation of tyrosine kinases or G-protein coupled receptors then activates AR transcriptional programs through alteration of the AR itself through phosphorylation or through modulation of components of the AR coregulator complex.
The cytokine IL-6 is produced by multiple cell types, including CRPC, and is a strong negative factor for patients with CRPC [53]. IL-6 induces the AR coregulator p300 and activates AR transcriptional programs in an androgen-independent manner [54, 55]. To date, targeting of IL-6 with monoclonal antibodies alone, or in combination with chemotherapy, has failed to provide clinical benefits for patients with CRPC [53, 56].
Many kinase pathways have been proposed to modulate AR activity by phosphorylation, including SRC, EGFR, ELK, HER2, and IGF-1R [57–61]. Androgen receptor phosphorylation modulates stability, nuclear translocation, and binding to relevant regulatory elements [57, 62]. Targeting a specific kinase pathway has been challenging given the number of potential redundancies between pathways. To date, clinical studies targeting these kinases, including EGFR, HER2 and SRC, have failed to identify effective agents, leaving this approach attractive but unproven [63–65].
Modulation of AR Co-regulatory Networks
Following AR translocation from the cytoplasm into the nucleus, the dimeric receptor binds to androgen response elements (ARE) on DNA and recruits co-regulatory proteins (including transcriptional coactivators and corepressors) to modulate transcription of target genes. The resulting protein complexes may change AR transcription by modulating chromatin structure and interacting with the RNA polymerase transcription complex.
AR is distinguished from other nuclear steroid receptors in that many of its binding sites do not contain the canonical nuclear receptor-binding motif, and may contain only a fraction of the ARE [66]. This suggests that co-regulatory complex formation, including other transcription factors, plays a more critical role in AR target gene transcription compared to other nuclear steroid receptors. Mapping of AR transcription factor binding sites in castration sensitive and CRPC tumors demonstrates they have distinctly different transcriptionally active sites, particularly with respect to genes regulating mitosis and cell cycle [5].
FOXA1 is a member of the forkhead family of transcription factors and has been proposed to play a critical role in CRPC. Members of this family are called “pioneer factors” because of their role in relaxing condensed chromatin and initiating recruitment of transcription factors to relevant binding sites. FOXA1 recruits multiple nuclear receptors to DNA binding sites, and is therefore expected to facilitate AR binding in prostate cancer cells. However, the role of FOXA1 may be more nuanced, as silencing of FOXA1 resulted in marked redistribution of AR binding sites in chromatin [48]. These findings strongly suggest that FOXA1 not only facilitates access of AR to some binding sites, but also masks other binding sites. Subsequent work suggests that FOXA1 regulates the considerably overlapping transcriptional programs of both AR and GR, and thus may have an important role in mediating anti-androgen resistance via GR signaling [49, 67].
p300 is a histone acetyl transferase and, together with IL-6, coactivates AR transcription [55], therefore has also been proposed as a critical coregulator in CRPC progression. Androgen deprivation substantially increases p300 levels, though the presence of a functional AR pathway is required for p300 modulation [68]. Knockdown of p300 decreased proliferation by suppressing cyclin proteins critical for cell cycle progression and suggests that under androgen-deprived conditions, p300 is upregulated to maintain AR signaling and cell survival.
Summary
The AR axis remains the central target in treating the most lethal form of prostate cancer, a conclusion supported by several phase III studies documenting clinical benefit from more potent AR blockade. Although most solid tumors are, by nature, heterogeneous, the majority of CRPC appears to be one of the two phenotypes: (1) “intracrine-dependent” tumors, which are those capable of activating AR through elaboration of tumoral androgens and (2) “ligand-independent” tumors, which remain dependent on aberrant AR signaling, including AR crosstalk with other pathways. The mechanisms of resistance to our current most effective androgen blocking agents, abiraterone and enzalutamide, continue to drive an AR transcriptional program [20, 31, 49, 69]. The challenge now is to determine to what degree aberrant AR isoforms, breakthrough androgen synthesis, or parallel signaling through other nuclear receptors are clinically relevant resistance mechanisms and how they can be effectively targeted. Until the AR-axis has been effectively extinguished, we will be unable to answer the ultimate question of whether AR targeting can cure both early- and late-stage prostate cancer.
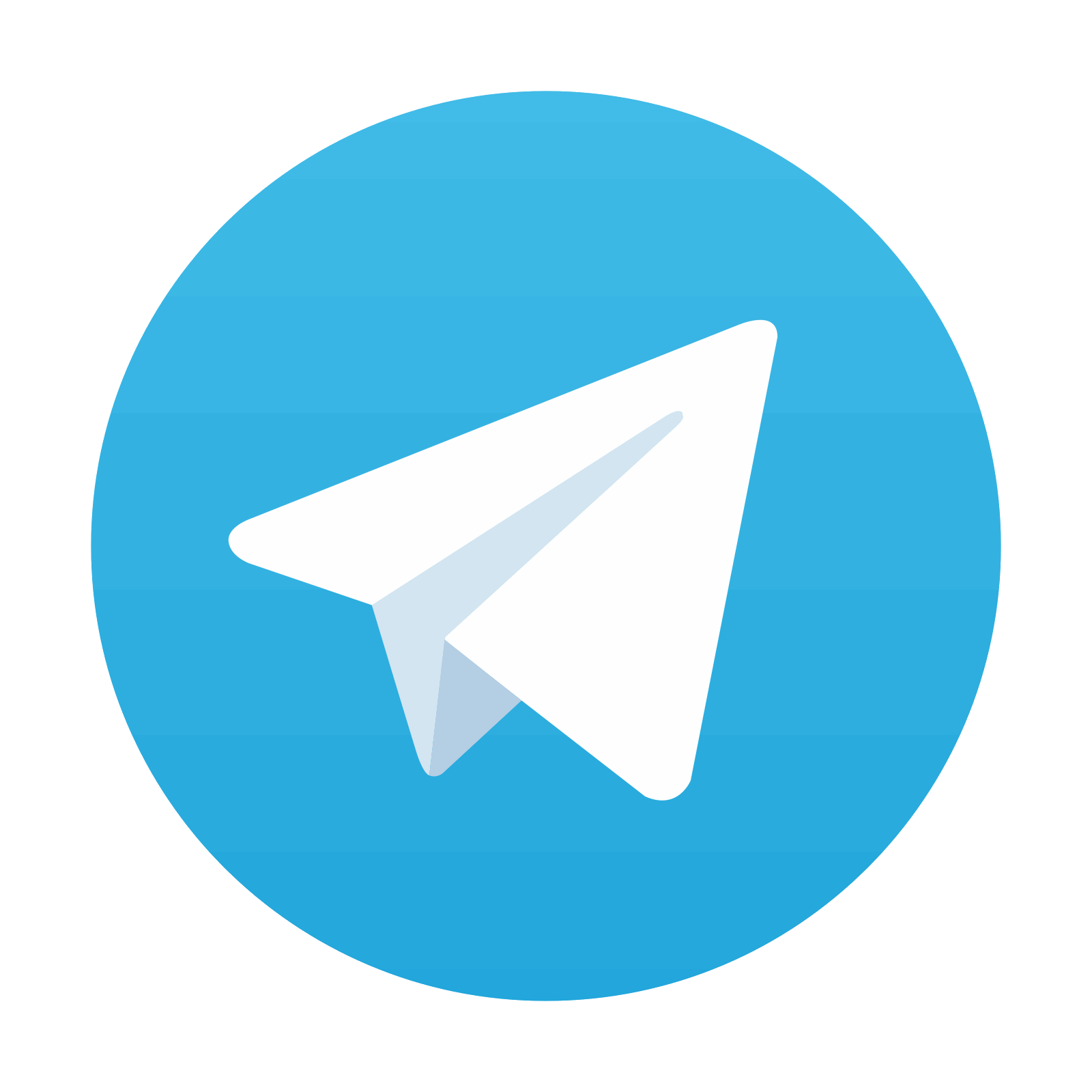
Stay updated, free articles. Join our Telegram channel

Full access? Get Clinical Tree
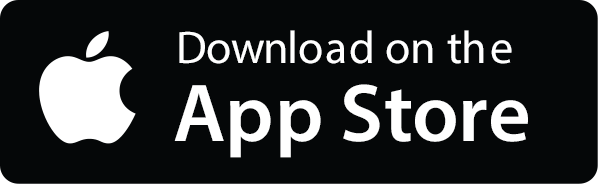
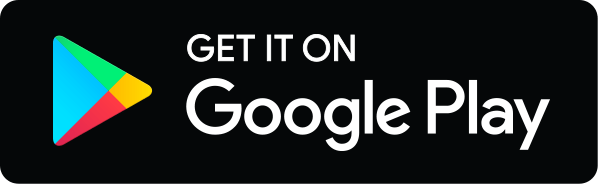