Fig. 1
Computed tomography scan with calcification in the suprasellar region indicative of craniopharyngioma
MRI is the diagnostic imaging of choice. It is useful to confirm the presence of cystic components and accurately measure the size and extent of the tumour. Craniopharyngiomas are typically located in the parasellar region, with a prominent suprasellar component in the majority. These tumours are described in relation to the optic chiasm (pre-, retro-, or subchiasmatic). Prechiasmatic tumours extend anteriorly displacing the chiasm postero-superiorly and they may also extend toward the frontal lobes and cause displacement of the A-1 segment of the anterior cerebral artery. Retrochiasmatic tumours tend to displace the optic chiasm anteriorly. Growth of retrochiasmatic tumours into the third ventricle can result in obstructive hydrocephalus. Posterior and inferior extension can result in displacement of the basilar artery and compression of the midbrain. Compression of the temporal lobes occurs with lateral growth. Approximately 30% of craniopharyngiomas extend anteriorly, 25% extend into the middle cranial fossa, and up to 20% have retroclival extension to the foramen magnum or the cerebellar pontine angle [6] (Fig. 2).
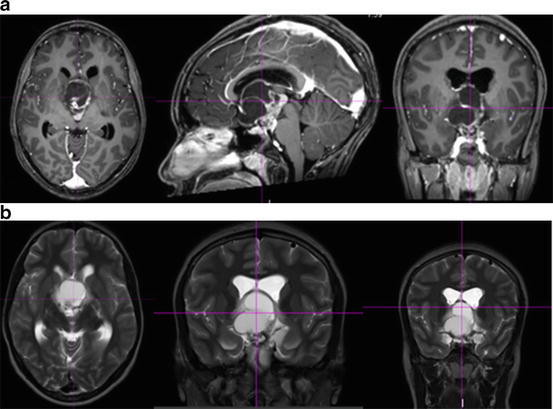
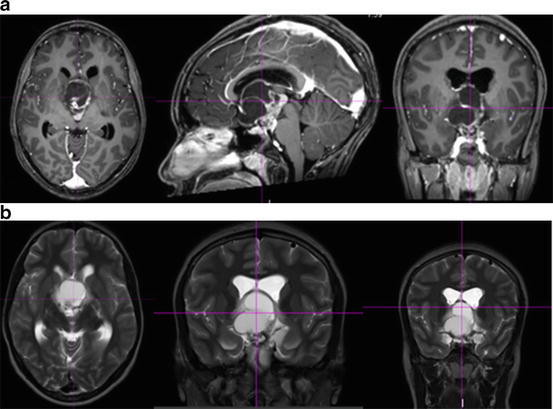
Fig. 2
Axial, sagittal, and coronal slices of (a) T1-weighted and (b) T2-weighted sequences of a magnetic resonance imaging scan demonstrating a solid/cystic suprasellar lesion
On T2-weighted sequences , including those with fluid-attenuated inversion recovery (FLAIR) , the solid portion is heterogeneous in intensity, and the cysts are mostly or partly hyperintense. Fluid-attenuated inversion recovery is useful in differentiating the cystic portions of the tumour from loculated portions of the third ventricle or other craniospinal fluid spaces that tend to be isointense. Calcifications are hypointense on T2 sequences. Susceptibility sequences, such as gradient recalled echo (GRE), are helpful in demonstrating calcifications and haemorrhage [4]. On T1 sequences, the cysts tend to be iso- to hyperintense. The solid component is generally smaller in proportion to the cystic component and enhances with the administration of contrast. Magnetic resonance angiography is an important study in identifying patients with baseline vessel stenosis or collateralisation. On magnetic resonance spectroscopy, cysts demonstrate a significant lipid content [4].
Differentiation between the more common childhood adamantinomatous craniopharyngioma and the adult squamous-papillary craniopharyngioma based on MRI features was reported by Sartoretti-Schefer et al. Of the 42 cases examined, 25 were adamantinomatous, 15 had squamous-papillary, and 2 mixed subtypes. Adamantinomatous craniopharyngiomas were seen mainly in children but also occasionally in adults. They were predominantly cystic and suprasellar in location. On T1-weighted images , the cysts were typically hyperintense. The tumour was mostly lobulated with a tendency to encase blood vessels. Calcifications were less commonly seen in the squamous-papillary subtype. The squamous-papillary type occurred in adults and was predominantly solid and occasionally demonstrated calcifications. When this subtype contained cysts, they appeared hypointense on T1-weighted images. There was no encasement of blood vessels and these tumours were mostly spherical in shape. Some of these features achieved statistical significance: squamous-papillary were predominantly solid and spherical with rare hypointense cysts; adamantinomatous type were lobulated, with frequent hyperintense cysts and vessel encasement [3].
The types of tumours in this area can often be distinguished based on imaging characteristics. Differential diagnoses of sellar and suprasellar mass lesions include Rathke’s cleft cyst (cystic, homogeneous, non-enhancing, rare calcification), pituitary adenoma (enlarged sella, homogeneous, and less cystic), meningioma (isointense on T1 and T2 and rarely cystic), and optic pathway glioma (may be cystic but are usually non-calcified) [5].
While the role of diagnostic imaging in craniopharyngioma is well established, and in some cases can obviate the need for biopsy, imaging can also be used to assist in the therapeutic management of craniopharyngiomas. Intraoperative MRI provides guidance for dissection. Hofmann et al. report a 16% increase in the rate of total tumour resection (TTR) using intraoperative MRI, resulting in a gross total resection (GTR) rate of 80% in their series with similar rates of new ophthalmologic (8%; 2/25) and endocrine deficits (44%; 11/25) compared with the literature [7–9].
Radiotherapy in the Management of Craniopharyngioma
Adamantinomatous craniopharyngiomas most commonly arise in the paediatric population, with a peak incidence between ages 5 and 14 years. The management of craniopharyngiomas has been debated for decades based on the theory that surgical resection of a benign tumour should achieve cure. Nevertheless, this simple solution does not always apply given the complex nature of this benign brain tumour . The location and intimate association of the tumour with critical structures such as the visual pathway, pituitary, hypothalamus, intra-cerebral vasculature, brainstem, and temporal lobes, and the morbidity associated with aggressive surgery have led to a multimodality approach using limited, safe surgery followed by radiotherapy with the goal of local control while minimising treatment-related sequelae.
According to recently published data from the Surveillance, Epidemiology, and End Result Program database [10], 644 patients were identified with craniopharyngioma between 2004 and 2008. On multivariate analysis, factors associated with prolonged survival included younger age, smaller tumour size, subtotal resection (STR), and radiotherapy. Black race was associated with an incidence rate of 2.1 cases per 1,000,000 person-years versus 1.7 cases per person-years and an age-adjusted relative risk for craniopharyngioma of 1.26 (95% confidence interval, 0.98–1.59) compared with white race. Survival rates at 1 and 3 years for the entire cohort were 91.5% and 86.2%, respectively. Black race was also associated with worse overall survival compared to white patients; 87.4% and 74.8% at 1, and 3 years versus 92.2% and 89.9%, respectively.
A significant risk of progression is present in cases without gross total resection and aggressive surgery carries a higher risk of surgical morbidity. Individualised therapeutic management in a multidisciplinary approach involving neurosurgery, radiation oncology, paediatric oncology, neuro-ophthalmology, neuro-psychology, and endocrinology is invaluable to achieve optimal outcomes with maximal tumour control and minimal morbidity [11–13]. Overall recurrence-free survival (RFS) rates at 5 and 10 years were 62% and 49% according to a study by investigators at the Children’s Memorial Hospital (Chicago, IL) [13]. The extent of tumour resection influenced RFS. With GTR, RFS rates were 83% and 70% at 5 and 10 years, while the RFS decreased to 71% and 36%, at 5 and 10 years, respectively, with STR and radiotherapy. Authors of this study concluded that recurrence rates and surgical complications remained high following radical resection.
In a retrospective analysis of 86 children who underwent GTR (103 operations), three children (3%) experienced perioperative deaths. The tumour recurred in 14 of 17 (20%) after GTR [14]. Factors negatively affecting overall survival and progression-free survival (PFS) included STR, tumour size >5 cm, and the presence of hydrocephalus or a ventriculoperitoneal shunt. Authors conclude that radical resection performed by experienced surgeons at presentation offers good chance of disease control and, while GTR does not preclude recurrence, it is more difficult to achieve in recurrent tumours [14].
The role of limited surgery followed by radiotherapy to doses of 5000–6550 Roentgens leading to improved disease control was reported as early as 1961 by Kramer et al. and was updated by Rajan et al. in 1993 [15, 16]. In 2010, based on an extensive literature review of 32 published reports, Merchant et al. concluded that conservative surgery and radiotherapy resulted in disease control rates ranging from 44 to 100%, with modern studies showing at least 90% disease control with 5-year follow-up [17]. In addition, other studies [18, 19] have shown similar rates of PFS (86% and 65%) with postoperative radiotherapy.
A large retrospective analysis of 122 patients treated between 1980 and 2009 included 46 patients who were ≤18 years old. In patients treated with GTR versus STR + radiotherapy no difference in overall survival or PFS rates was identified (p = 0.544 and p = 0.735, respectively) [20]. As expected, STR alone resulted in a significantly inferior PFS compared to STR + radiotherapy or GTR (p = <0.001 for both). STR was associated with significantly decreased overall survival compared to STR + radiotherapy (p = 0.050) with a trend to shorter overall survival compared to GTR (p = 0.066). GTR was also significantly associated with a greater risk of developing diabetes insipidus (56.3% vs 13.3%, p < 0.001) and panhypopituitarism (54.8 vs 26.7%, p = 0.014) compared with STR + radiotherapy.
The effect of modern treatment techniques and the use of combined-modality therapy with limited surgery and radiotherapy were evaluated in a retrospective analysis of children with craniopharyngioma treated from 1976 to 2003 [21]. Recurrence rates after limited surgery and radiotherapy were 21% in the pre-1988 era and 5% in the post-1988 era. Investigators also noted that patients treated with surgery alone were 7.7 times more likely to recur compared to those treated with the combined approach (95% confidence interval: 2.0 to 28.7). Although there was no difference in the 10-year overall survival rate between patients treated with surgery alone versus those treated with combined-modality therapy (97% vs 93%; p = 0.53), the 10-year local control rate was higher for patients treated with combined-modality therapy compared to surgery alone (84% vs 52%; p = 0.006). Radiotherapy in this series varied from a conventional three-field, 3D conformal technique, stereotactic fractionated radiotherapy, arc therapy, and intensity-modulated radiotherapy (IMRT) .
The timing of radiotherapy invites debate. Lin et al. [22] in 2008 reported overall survival and PFS rates in a retrospective study of 31 children; early radiotherapy showed a 100% local control rate compared to 32% when radiation was delivered at relapse. The data is countered, however, by several other studies [13, 23–26] that have indicated comparable overall survival and PFS with early versus delayed or salvage radiotherapy.
Acceptable local control and overall survival achieved with combined-modality therapy appropriately raise concerns of the late effects of radiotherapy, a highly relevant survivorship issue as it can permanently affect function and quality of life. Young age is an established risk factor for neurocognitive decline in children receiving cranial radiotherapy [27–29]. With improved dose conformality and decreased exposure of adjacent critical structures, technological advancements in radiotherapy may maintain disease control while reducing in the long-term toxicity of treatment.
Radiotherapy
External-beam radiotherapy involves directing beams of high-energy radiation from outside the body using various modalities such as X-rays (photons), gamma rays, and electron beams, and particles such as protons or heavy ions like carbon. Another form of therapeutic radiation known as brachytherapy is delivered via a radioactive source placed within a body cavity or tissue, as in the treatment of uterine or prostate cancer. Radiation oncologists exploit the different physical characteristics of each type of ionising radiation to produce an individual treatment plan for each patient. Ionising radiation causes damage to cell DNA, leading to cell death. Unlike abnormal tumour cells, normal cells have the ability to repair this damage.
Preparation and Delivery of Radiotherapy
Patient Set-up and Data Acquisition
Patient position , immobilisation, and reproducibility of position for daily treatment are important considerations when planning external-beam radiation. For the treatment of a craniopharyngioma, patients are typically in the supine position and immobilised using a face/head mask with or without a bite plate to reduce intra- and interfraction motion (Fig. 3). Patients undergo a CT simulation scan with a slice thickness of 1–2 mm. Marks on the mask in conjunction with triangulating lasers in the room are used for the daily alignment of the patient for treatment. Daily image guidance is recommended to minimise set-up uncertainties. Cone-beam CT , when available, provides robust image guidance. For proton therapy , daily image guidance is currently performed with orthogonal kV X-rays typically aligned to bony landmarks of the skull (Fig. 4). Digital radiographic reconstructions (DRRs) generated from the CT simulation images are used to reproduce the position set during CT simulation. As bone development is incomplete in the prepubertal age group, bony landmarks may be suboptimal for daily patient alignment in this age group [30]. As a result, some institutions recommend placement of four small titanium fiducial markers prior to proton radiotherapy for these young patients to assist in optimal daily alignment. In addition, daily patient set-up can be aligned to existing surgical hardware when present.
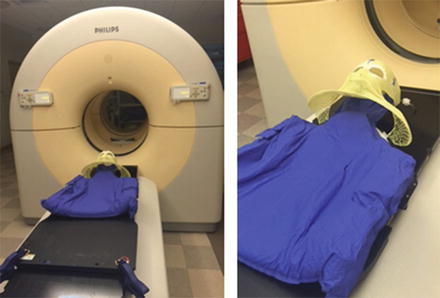
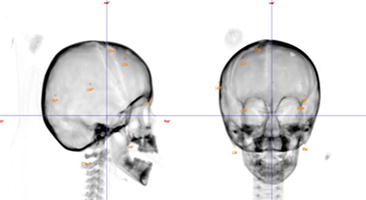
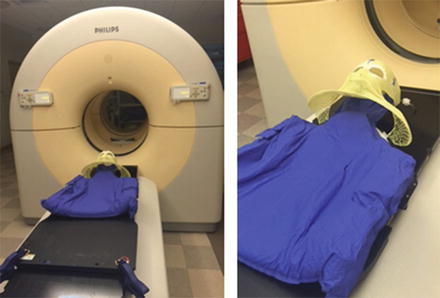
Fig. 3
Computed tomography simulation with face mask and body mould
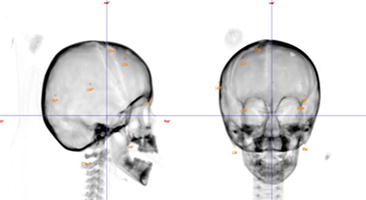
Fig. 4
Orthogonal images using bony landmarks for daily patient alignment in proton therapy
In the delivery of proton therapy, patients undergo a CT simulation scan with a slice thickness of 1 mm. A similar set-up is used for fractionated stereotactic radiotherapy and stereotactic radiosurgery. Young children may require a general anesthetic for CT simulation as well as for each fraction of radiation treatment delivery.
Target Volume Delineation
Target volumes are defined on the CT simulation images. Diagnostic high resolution MR (1–1.5 mm slice thickness) T1 and T2 weighted post contrast and FLAIR sequences are used, with image fusion to the planning CT images for accurate delineation of targets and organs at risk. Calcifications seen on the CT scan also help define the gross tumour volume (GTV). The gross tumour is expanded by 5 mm to obtain the clinical target volume (CTV), which may be subsequently enlarged to encompass any surface originally in contact with the tumour, or decreased based on anatomical constraints. A further 3–5 mm institution specific margin is added to the CTV for set-up uncertainty to establish the planning target volume (PTV). A 10-mm CTV margin is known to be adequate [31]. Other data showed no difference in progression-free survival at 5 years between those treated with a >5 mm versus ≤5 mm CTV margin [32]. An ongoing phase II protocol (RT2CR) at the University of Florida in collaboration with St. Jude Children’s Research Hospital is examining the efficacy of limited surgery, proton therapy, and a limited margin (5 mm CTV) for craniopharyngioma. Cyst enlargement during radiation is well recognised and, therefore, early, regular surveillance and adaptive planning are recommended (Fig. 5) [33–35].
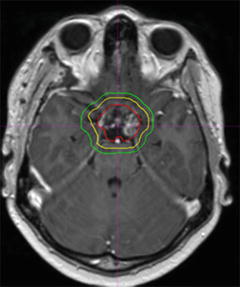
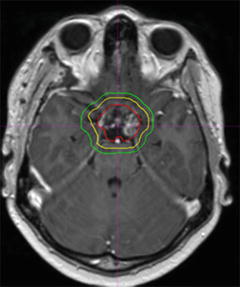
Fig. 5
Representative slice of craniopharyngioma target definition. Gross tumour volume in red; clinical target volume in yellow; planning target volume in green
In addition to the treatment target volumes, normal tissues or organs at risk are contoured as avoidance targets, including the optic chiasm, optic nerves, brainstem surface, brainstem core, bilateral retinae, lens, bilateral cochlea, lacrimal glands, temporal lobes, hippocampal head and tail bilaterally, pituitary, hypothalamus , bilateral mastoid air cells, posterior nasopharynx, scalp, and supratentorial brain.
Types of Radiotherapy
Conventional External-beam Radiotherapy and 3-Dimensional Conformal Radiotherapy
Conventional external-beam radiotherapy , the most common type of radiation used worldwide, is delivered in the form of photon beams (X-rays) using linear accelerators. The total dose of radiation is usually delivered over a fractionated course of 1.8–2 Gy per fraction, each day, allowing for normal tissue repair between fractions. Normal tissue tolerance to radiotherapy along with the therapeutic dose required to adequately treat the tumours guide radiotherapy design. The fundamental principle of radiotherapy is to deliver a therapeutic dose to the target volume while minimising the dose to adjacent normal structures. The tolerance dose of the brainstem is 54–59.4 Gy and that of the optic nerves and chiasm is 55–60 Gy [37]. Sensitivity of the pituitary’s endocrine function varies with growth hormone demonstrating the highest sensitivity (QUANTEC) [38]. These goals are achieved by incorporating modern techniques in the radiotherapy planning process, such as the use of three-dimensional (3D) imaging including CT and MRI to assist with target volume and normal structure delineation, reproducible patient immobilisation, and enhanced precision delivery techniques like image guidance.
A 3D conformal plan to treat craniopharyngioma is typically delivered using non-coplanar beams shaped by a multileaf collimator. A common beam arrangement consists of a superior oblique beam arrangement and opposing lateral or posterior oblique beams (Fig. 6).
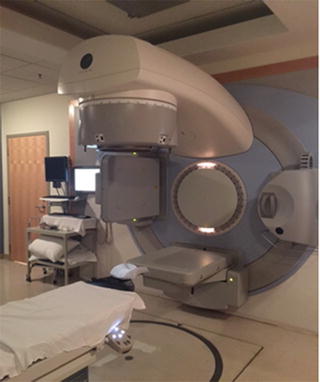
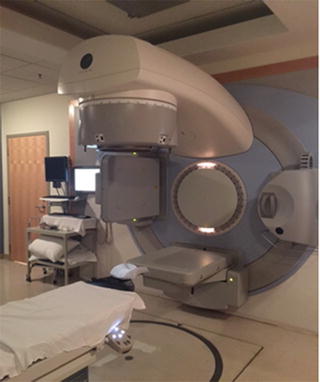
Fig. 6
Linear accelerator ± 3-dimensional conformal craniopharyngioma plan
Rajan et al. reported one of the largest and earliest series of patients treated with limited surgery and external-beam radiotherapy for craniopharyngioma [16]. Of the 173 patients, 22 received radiotherapy alone. At a median follow-up of 12 years, the 10- and 20-year PFS rates were 83% and 79%. The 10- and 20-year overall survival rates were 77% and 66%. Age (<40 years old) and use of modern techniques were found to be significant independent positive prognostic factors for survival. Other prognostic factors that influenced outcome was extent of surgery, radiation dose of <55 Gy, and treatment prior to the routine use of diagnostic CT scans (between 1961 and 1974) [19].
A smaller series from Philadelphia [39] with 19 paediatric patients showed a higher 20-year survival rate for those treated for primary disease (78% versus 25%) compared to those treated for a recurrence [24]. Overall, the 20-year survival rate was 62%. In addition, surgical extent, radiation dose (≤54 Gy vs ≥54 Gy with a recurrence rate of 50% in those receiving <54 Gy and 15% in those receiving >54 Gy), and treatment prior to the routine use of CT scans (between 1961 and 1974) appeared to have a major influence on patient outcomes.
Another large retrospective analysis included 61 children treated between 1979 and 1990 [18]. Among them, nine children were treated with radiotherapy alone, 15 with surgery alone, and 37 with both surgery and radiotherapy to a median dose of 5464 cGy. At a median follow-up of 10 years (range, 2–20.5 years), the 10-year actuarial overall survival rate was 91% for all patients. The 10-year actuarial freedom from progression rate for the surgery group was 31% compared with 100% for the radiation-alone group and 86% for the patients treated with surgery and radiotherapy at diagnosis. This study established tumour size greater than 5 cm to be an important predictor of local recurrence.
Intensity-Modulated Radiotherapy
IMRT uses multiple small beamlets of different intensities to treat the target volume by precisely sculpting the high-dose volume around complex target shapes, thereby significantly reducing the dose to adjacent organs at risk. IMRT is delivered using either a dynamic or static multileaf collimator or by a multileaf intensity-modulating collimator using rotational arc therapy (such as RapidArc or volumetric arc therapy). IMRT can significantly reduce the dose to adjacent organs at risk due to its ability to conform the high dose to complex shapes. However, the cost of the conformal high dose is an increased low dose exposure of surrounding normal tissue due to the multiple beams or arcs used to achieve the conformal high target dose (Fig. 7).
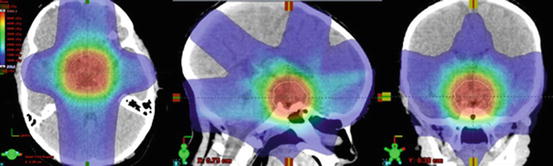
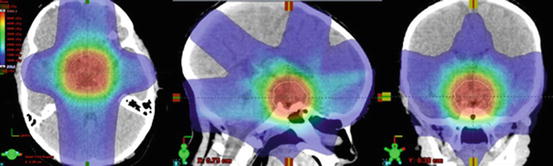
Fig. 7
Axial, sagittal, and coronal slices demonstrating a low dose entry and exit doses in an IMRT plan
In a retrospective review of 24 children treated with IMRT, the 5- and 10-year PFS rates were 65.8% and 60.7% [40]. The 5- and 10-year cystic PFS rates were 70.2% and 65.2%, respectively. The solid PFS rates were no different at 90.7%. Half of these patients had received radiotherapy as salvage therapy for disease progression on imaging following initial surgical treatment. Dynamic cyst changes, both expansion and reduction (range, −20.7% to 82%), can occur during radiotherapy [33] and warrant adaptive planning based on surveillance weekly MRI. Adaptive targeting allows for both target volume coverage and the limiting of dose to adjacent critical structures such as the optic chiasm, which is particularly relevant when a smaller CTV is used with modern techniques. Compared to traditional conformal RT (CRT) using a 5-mm CTV margin and 3-mm PTV margin, IMRT with similar margins reduced the mean dose to the cochlea from 18.2 to13.3 Gy (p < 0.001), temporal lobes from 14.3 to 7.9 Gy (p < 0.001), and hippocampus from 26.8 to17.6 Gy (p < 0.001). Although IMRT plans are more susceptible to the effects of dynamic cyst changes compared to 3D–conformal plans, IMRT is favoured to reduce the dose to critical structures when weekly surveillance imaging is available [33].
Fractionated Stereotactic Radiotherapy
Fractionated stereotactic radiotherapy combines the precise dose delivery of stereotactic radiosurgery through noninvasive immobilisation with the radiobiological advantage of fractionation, enabling the treatment of tumours that may be too large for stereotactic radiosurgery [41]. A study on stereotactic fractionated radiotherapy included 39 patients with a median age of 18 years (range, 3–68 years) treated with stereotactically guided conformal radiotherapy of 30–33 daily fractions over 6 to 6.5 weeks to a total dose of 50 Gy using 6-MV photons. All patients had previously undergone one or more surgical procedures. The 3- and 5-year PFS rates were 97% and 92%. The 3- and 5-year overall survival rates were 100%. Two patients required debulking surgery for progressive disease at 8 and 41 months. Twelve patients (30%) experienced acute clinical deterioration secondary to cyst enlargement and required cyst aspiration. Seven patients with normal pituitary function prior to radiotherapy had no deficits following radiotherapy at a median follow-up of 40 months. One patient with severe visual impairment before radiotherapy deteriorated and lost vision after radiotherapy.
Long-term outcomes from another study report local control rates of 95.3%, 92.1%, and 88.1% at 5, 10, and 20 years, respectively, with a median follow-up time of 128 months (range, 2–276 months) after stereotactic fractionated radiotherapy to a dose of 52.2 Gy (range, 50–57.6 Gy) at 1.8 Gy per fraction [42]. The overall survival rate at 10 and 20 years was 83.3% and 67.8%, respectively. This study included 55 patients with a median age of 37 years (range, 6–70 years) treated between 1989 and 2012, 8 of whom were younger than 18 years old. Toxicities reported included 1 patient with complete anosmia and 1 patient with further deterioration in vision. Of note, neuropsychological testing was not performed.
Proton Therapy
Protons are positively charged particles with a unique property of depositing their maximum energy in tissue shortly before they come to rest [43]. Therapeutic proton beams are generated from a cyclotron, a type of particle accelerator, to attain the desired energy and thus the desired depth of penetration. As protons enter and travel through tissue, they deposit little dose until their maximum depth is reached, at which point they deposit dose in a characteristic sharp, narrow distribution called the Bragg peak. As protons come to rest abruptly after the Bragg peak, the “exit dose,” or dose distal to the target, is eliminated. This lack of dose deposition beyond the target lies in contrast to photons, where additional dose is deposited beyond the target as the beam exits the body beyond the distal aspect of the target. A range of energies is used to create a spread-out Bragg peak (SOBP) . The SOBP allows dose deposition over a greater length of tissue, resulting in high dose to the entirety of the target volume. This unique property of protons results in the primary clinical advantage of decreased normal tissue exposure and thereby reduced toxicity (Fig. 8).
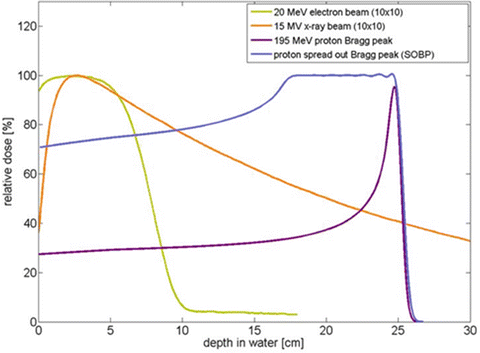
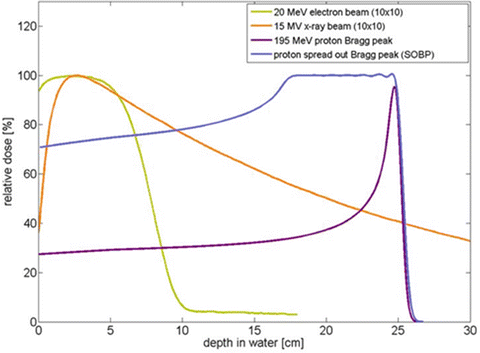
Fig. 8
Depth dose distribution for 195 MeV proton displaying the low entrance dose, Bragg peak with a sharp distal drop off; spread-out Bragg peak (SOBP); 15 MV X-ray (photons) demonstrating an initial buildup of dose followed by a gradual decline with depth; 20 MeV electron beam displaying the higher entrance dose and low dose at depth
Protons, like photons, are an ionising radiation with a low linear energy transfer. The relative biologic effectiveness (RBE) of protons is approximately 1.1; therefore, unlike heavy ion therapy, which also eliminates the exit dose but carries a high linear energy transfer, protons and photons have a similar and more predictable impact on normal tissue.
The primary benefit of proton therapy in the treatment of craniopharyngioma is a decrease in the undesired dose to adjacent critical structures and lower integral dose while maintaining adequate dose to the tumour. This decreased dose to surrounding critical structures is expected to translate into fewer acute and late toxicities from radiotherapy . The reduction in late toxicity is particularly relevant in this predominantly childhood tumour where durable local control is achievable (Fig. 9).
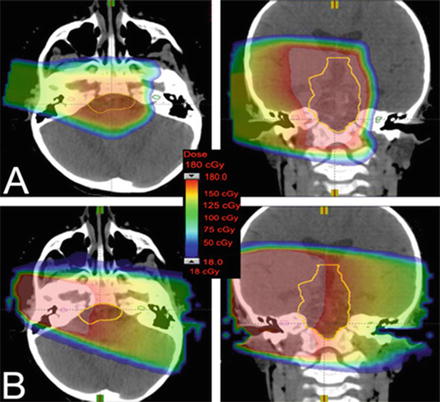
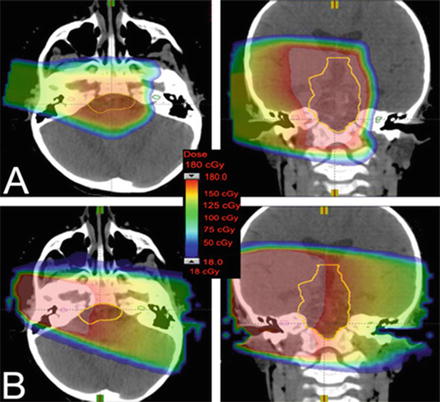
Fig. 9
(a) Dose distribution from one axial and coronal proton beam compared to (b) one axial and coronal photon beam demonstrating the higher entrance dose for photon beams and the lack of exit dose with the proton beam
This advantage of proton therapy possible through exploiting the Bragg peak has been evaluated in multiple dosimetric studies. A study of ten paediatric craniopharyngioma patients compared three-dimensional conformal proton therapy, IMRT, and intensity-modulated proton therapy (IMPT) [44]. Coverage of the PTV was adequate for all modalities. The IMRT and IMPT plans were the most conformal, but offered a less homogenous dose distribution. 3D conformal proton and IMPT plans allowed a relative reduction in the integral dose to the hippocampus, dentate gyrus, subventricular zone, vasculature, infratentorial and supratentorial brain, brainstem, and whole brain. Another study on 14 patients with craniopharyngioma compared double-scatter proton therapy, IMPT, and IMRT [45]. Conformality index was higher with IMPT and IMRT. Heterogeneity and the dose to organs at risk, such as the cochlea, optic chiasm, and brain, was lower with double-scatter proton therapy.
Decreasing the integral dose with proton therapy should decrease the incidence of radiation-induced tumours. According to the Childhood Cancer Survivor Study assessing all types of tumours, the cumulative incidence of a second malignancy at 30 years after diagnosis was 7.9% [46]. The relative risk of a second malignancy in patients treated with radiotherapy was 2.7. A 2- to 15-fold decrease in the rate of second malignancies with proton therapy compared to photon radiotherapy was demonstrated [47].
Another potential means to reducing the integral dose with the use of proton therapy is through the development of cognitive dose models to allow predictive comparison of outcomes. Based on one such longitudinal model in patients with craniopharyngioma, a diminution in intelligence quotient (IQ) was noted in younger patients (5 years vs 9 years) and with the use of photons compared to double-scatter proton therapy [48]. A large prospective study of 70 craniopharyngioma patients who underwent cognitive testing at baseline, 6 months, after radiotherapy, and then annually for 10 years demonstrated an association of longitudinal IQ with mean whole-brain radiation dose and time with a loss of 0.0027 points/cGy/year [32]. Table 1 summarises current evidence of outcomes from proton therapy for patients with craniopharyngioma.
Table 1
Evidence of outcomes from proton therapy for patients with craniopharyngioma
Study; no. of patients | Median follow-up (years) | Treatment modality | Actuarial 5-year local control rate | Acute toxicity | Late toxicity | Mortality (absolute number) |
---|---|---|---|---|---|---|
Fitzek et al. 2006; N = 15 | 13.1 | Surgery/biopsy + proton-photon | 93% 5-year | none,7; nausea, 1; fatigue,3; headaches,4 | Visual deficits,2; endocrinopathy,15; learning difficulty,1 | PD, 2; vascular complications, 1; treatment-related hypothalamic syndrome, 1 |
Luu et al. 2006; N = 16 | 5 | Surgery + proton or proton alone (Surgery+RT, 4; recurrent after surgery, 12; re-resection + RT, 7; RT, 4) | 94%b (recurrence at 80 months, 1) | NR | Panhypopituitarism, 1; CVA, 1 with full recovery; meningioma, 1 following reirradiation | 3 at 12, 52, and 120 months after re-resection and RT (PD, 1; sepsis, 1; MCA infarct, 1) |
Chang et al. 2009a; N = 14 | 1.3 | Surgery/biopsy + proton therapy | 100%b | NR | Vision, stable or improved; endocrinopathy, 11 (of 11 with results) | 0 |
Alapetite et al. 2012a; N = 49 | 4.4 | Surgery + proton-photon, 10; surgery + proton , 39 | 90%b | NR | Altered short-term memory, social and emotional functioning and significant school difficulties in children who had RT after several surgeries. Behavioural disorder rates lower after STR+RT | NR |
Confer et al. 2012a; N = 13 | 0.7 | Surgery/biopsy + proton | 85%b | Grade 2 headache, 1 | NR | 0 |
Indelicato et al. 2013a; N = 40 | 0.7 | Surgery/biopsy + proton therapy | 100%b | Emesis, 1; headache, 1; presyncope, 2; nausea, 9 | None to date | 0 |
Bishop et al., 2013a; N = 19 | 2.7 | Surgery/biopsy + proton, 15; proton alone, 4 | 91% | NR | Vasculopathy, 2; endocrinopathy, 17, of which 15 were post-op and 2 new after RT | secondary to surgically induced DI, 1 |
Winkfield et al. 2008a; N = 24 | 3.7 | Surgery/biopsy + proton therapy | 100% | NR | NR | intracranial haemorrhage at 1 year, 1 (in a child with 3 previous surgeries followed by RT) |
Early reports on the use of proton therapy in craniopharyngioma primarily include patients treated with a combined proton-photon technique. In one analysis of 15 patients treated between 1981–1988, one-third of the cohort was treated with proton therapy alone and two-thirds with a combined technique [49]. The analysis included five children and ten adults. Due to a change in the calibration for proton dosimetry in 1997, the dose delivered was recalculated and found to be 6.5% greater than the prescribed dose. The median delivered dose was 55.6 Gy (RBE) for the paediatric patients and 62.7 Gy (RBE) for the adult patients. Proton dose contribution to the total dose in those who underwent a combined treatment was 47% (median dose, 26.9 Gy [RBE]). All treatments were delivered using the same non-coplanar three-field technique with two lateral beams and one superior oblique field. The actuarial 5- and 10-year survival rates observed were 93% and 72%, respectively. The 5- and 10-year local control rates were 93% and 85%, respectively. No paediatric patients experienced tumour progression. These findings were further substantiated by data from the same institution in a report of 24 children who underwent proton therapy for biopsy-proven craniopharyngioma to a median dose of 52.2 Gy (RBE) (range, 52.2–54 Gy [RBE]) between 2001 and 2007 [50]. The local control, PFS, and overall survival rates were 100%, 100%, and 94%, respectively, at a median follow-up of 45 months (range, 3–95 months).
In a study by Luu et al., proton therapy was utilised as salvage therapy in 16 patients who had undergone primary radical resection [51]. Of these, seven patients underwent more than one surgical resection. Nine others underwent one resection. Four of these received adjuvant proton therapy, five were treated with proton therapy at recurrence. One patient was lost to follow-up. At a mean follow-up of 60.2 months (range, 12–121 months), local control was achieved in 14 patients and only one patient had a relapse at 80 months.
Alapetite et al. reported on 49 patients with craniopharyngioma, 39 of whom were treated with protons alone and ten with a combination of protons and photons between 1994 and 2009. At a median follow-up of 53 months (range, 24–156 months), five patients experienced disease relapse, one of which occurred along the surgical access route after 56 months; the other four were cystic relapses at a median time of 43 months (range, 3–68 months) [52].
Indelicato et al. from the University of Florida reported early clinical outcomes in a retrospective analysis of 40 children with a median age of 7.8 years (range, 1.5–19.3 years) treated with double-scatter proton therapy from 2008 to 2012. Of these children, 35 received 54 Gy (RBE) (range, 50.4–54 Gy [RBE]). At a median follow-up of 0.7 years (range, 0.1–3.7 years), the solid tumour control and survival rates were 100% [53].
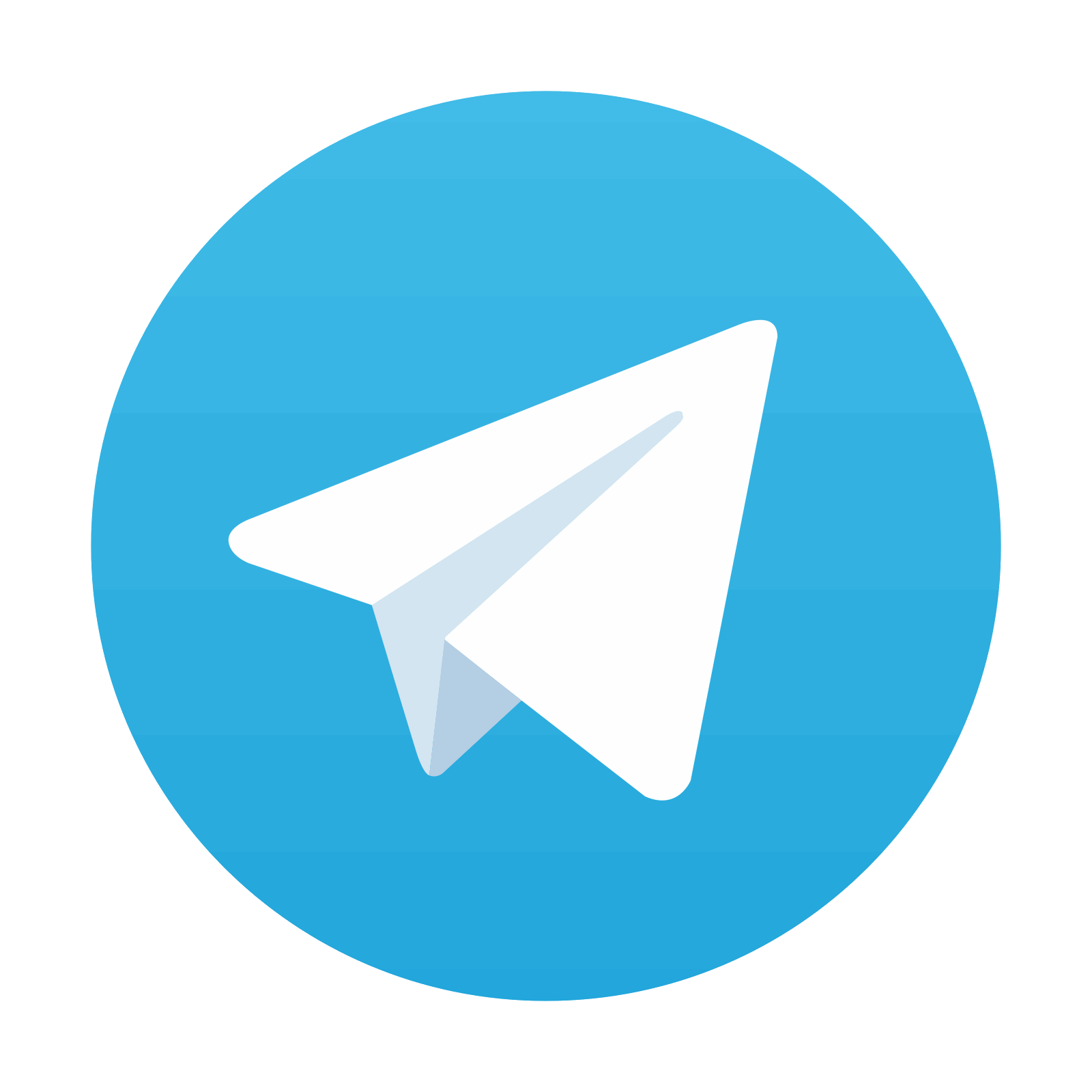
Stay updated, free articles. Join our Telegram channel

Full access? Get Clinical Tree
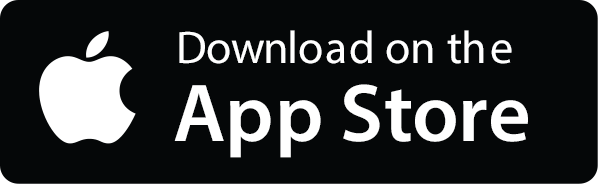
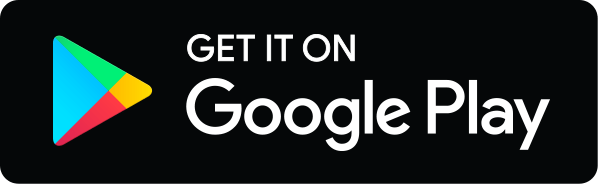
